DOI:
10.1039/C1AN15745K
(Paper)
Analyst, 2012,
137, 98-105
Production of monoclonal antibodies against fullerene C60 and development of a fullerene enzyme immunoassay†
Received
17th August 2011
, Accepted 26th September 2011
First published on 3rd November 2011
Abstract
The aim of the present study was to produce monoclonal anti-fullerene C60 antibodies and to develop the enzyme immunoassay for the detection in the first use of free fullerene C60 both in solutions and in multicomponent biological probes. The immunization of mice with the conjugate of fullerene C60 carboxylic derivative with thyroglobulin synthesized by carbodiimide activation led to the production of eight clones of anti-fullerene antibodies. The specificity of the antibody-fullerene binding was confirmed. Indirect competitive enzyme-linked immunosorbent assay (ELISA) was developed for the determination of water-soluble protein–conjugated fullerene, the fullerene aminocaproic acid, fullerenol and for pristine fullerene in solution. To solubilize extremely hydrophobic free fullerene C60 a specially selected water–organic mixture compatible with immunoassay was proposed. The detection limit of free fullerene C60 in solution was 2 μg L−1. Fullerene C60 was also detected by ELISA in organ homogenates of rats intraperitoneally or intragastrically administered with fullerene. To reduce the influence of biomatrices on the assay results a technique was developed for the biological sample pretreatment by the extraction of C60 from bioprobe by toluene followed by the evaporation of toluene and dissolution of the fullerene-containing extract in the selected water-organic media. The ELISA procedure in the first use allowed the detection of fullerene C60 in different tissues.
Introduction
Due to an increasing growth in manufacturing fullerenes, engineered carbon nanoparticles with a unique structure, and their wide application for industrial and biomedical purposes1–5 there is a need for the development of new approaches for the fullerene control in living organisms and environment. In comparison to high performance liquid chromatography which nowadays serves as a main technique for the determination of fullerene6–8 an immunochemical assay can be considered as a promising alternative for the detection of fullerene nanoparticles. The latter method has such advantages as the rapidity, high sensitivity, the low detection limit, the absence of complicated preparatory steps and the possibility of automation.9 However, carbon nanoparticles appear to be unconventional immunogens, which do not have inducers of immune response typical of native biopolymers. This may be an impediment to the production of fullerene-specific antibodies. In addition, obstacles to solubilization of hydrophobic fullerenes in an aqueous medium or at least in polar solvents compatible with immunoassay are expected to be additional problems. The first research on the production of specific anti-fullerene C60 antibodies and the development of an immunoanalytical system for the detection of fullerene C60 was accomplished by Chen et al.10 In the cited study, the authors succeeded in producing anti-fullerene C60 polyclonal antibodies and developed the enzyme-linked immunosorbent assay (ELISA) of water-soluble fullerene C60 derivatives (conjugates of [1-methylsuccinate-4-methylcyclohexene-2]-buckminsterfullerene with the proteins rabbit and bovine serum albumins and the peptide pentalysine). The detection limit of C60–protein conjugates was approximately 2 μg ml−1. Analysis of free fullerene was not carried out by the authors due to its extremely low solubility in aqueous media. Then Braden et al.11 produced monoclonal antibodies specific for fullerenes. The affinity of the interaction between anti-fullerene C60 antibodies and the water-soluble fullerene C60–BSA derivative as the antigen measured by competitive ELISA was 20 nM. In the cited work the fullerene binding site was determined by X-ray crystallographic analysis of the anti-fullerene C60 antibody Fab fragment and by the model building. Erlanger et al.12 showed that the anti-fullerene C60 monoclonal antibodies recognize and bind specifically to colloid-dispersed single-wall carbon nanotubes confirmed by ELISA and atomic force microscopy.
The reported binding of antibodies to water-soluble protein–conjugated forms of fullerene is of obvious interest because fullerene nanoparticles are known to form complexes with proteins after the introduction into living organisms.13,14 However, these pioneering results obtained in 1998–2001 were not followed by further studies of interactions between anti-fullerene antibodies and pristine fullerene and the development of an immunoanalytical system for the detection of free fullerene in solutions and biological samples.
In our previous studies15 we immunized rabbits with C60–protein immunogens and produced polyclonal anti-fullerene antibodies with properties similar to those obtained in ref. 10. The enzyme immunoassay developed in ref. 15 allowed the determination of fullerene-conjugated forms with a detection limit of up to 0.04 μg L−1 (calculated for coupled fullerene C60) that considerably exceeded characteristics of ELISA described in ref. 10.
The aim of the present work was to produce and comprehensively characterize monoclonal anti-C60 antibodies that can recognize not only modified water-soluble fullerene derivatives but also free fullerene both in solutions and in multicomponent biological samples thus considerably increasing the facilities of immunodetection of this compound.
Experimental
Fullerenes C60 with 99.95% of purity and C70 with 99% of purity were purchased from SES Research, USA. Multi-walled carbon nanotubes (MWCNT, diameter 5–50 nm) were purchased from Nanocyl, Belgium. Fullerene C60 carboxylic derivative ((1,2-methanofullerene C60)-61-carboxylic acid)), N-hydroxysuccinimide, 1-cyclohexyl-3-(2-morpholinoethyl)carbodiimide metho-p-toluenesulfonate, 3,3′,5,5′-tetramethylbenzidine, 2,4,6-trinitrobenzenesulfonic acid, porcine thyroglobulin (PTG), bovine serum albumin (BSA), PAMAM dendrimer, 1,4-diaminobutane core, generation 1.0, were purchased from Sigma-Aldrich, USA. A fullerene aminocaproic acid (C60(H)3(NH(CH2)5COONa)3) and fullerenol (C60(OH)24) both of 98% purity were purchased from Intelfarm, Russia. Soybean trypsin inhibitor (STI) and N,N-dimethylformamide (DMF, certified A.C.S. grade) were purchased from ICN Biomedicals, USA. Peroxidase-labeled goat anti-mouse antibodies were purchased from the Gamaleya Research Institute of Epidemiology and Microbiology, Moscow, Russia. Pyridine (certified A.C.S. grade) and Triton X-100 were purchased from Panreac, Spain. 2,4-Dichlorophenoxyacetic acid (2,4-D) was purchased from the Institute of Chemical Means of Plant Protection, Moscow. Flavourzyme 500L was purchased from Novozymes A/S, Denmark. Other reactants and buffer components were of analytical grade. To control specificity of the immune interaction monoclonal antibodies against ochratoxin A, chloramphenicol, plum pox virus and potato virus X produced earlier by the authors were used.
Fullerene–protein conjugates (see structure (a) in Fig. 1) were synthesized as described in ref. 10 with some modifications.15 Initially, 1.6 μmol of the fullerene C60 carboxylic derivative ((1,2-methanofullerene C60)-61-carboxylic acid) were dissolved in 0.25 ml of dry pyridine. Then a solution of 70 μmol of N-hydroxysuccinimide and 43 μmol of 1-cyclohexyl-3-(2-morpholinoethyl)carbodiimide metho-p-toluenesulfonate in 0.15 ml of dry pyridine was added. The reaction mixture was stirred for 48 h at room temperature and then added dropwise within a period of 5 min to 1 ml of a protein solution in 20 mM sodium carbonate buffer, pH 9.5, so that the initial hapten to protein molar ratios were 100
:
1, 20
:
1 and 10
:
1 for porcine thyroglobulin (PTG), bovine serum albumin (BSA) and the soybean trypsin inhibitor (STI), respectively. The incubation of the reaction mixture was performed with orbital shaking for 4 h at room temperature. Then the reaction mixture was dialyzed against 50 mM phosphate buffered saline, pH 7.4, with 0.1 M NaCl (PBS) overnight at 4 °C and then centrifuged at 1500 g for 30 min. The quantity of hapten molecules per protein molecule was estimated spectrophotometrically at 330 nm.
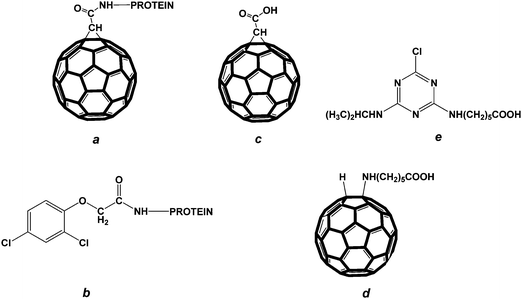 |
| Fig. 1 Structures of the compounds used in the study: (a) fullerene–protein conjugate; (b) conjugate of 2,4-D with protein; (c) fullerene carboxylated derivative, (1,2-methanofullerene C60)-61-carboxylic acid; (d) fullerene carboxylated derivative, a fullerene aminocaproic acid; (e) atrazine carboxylated derivative. | |
The conjugates of 2,4-dichlorophenoxyacetic acid (2,4-D) with PTG, BSA and STI (see structure (b) in Fig. 1) were synthesized by the carbodiimide/hydroxysuccinimide technique according to Dzantiev et al.16
Production of monoclonal antibodies
BALB/c mice weighing 16–18 g were twice immunized with the C60–PTG conjugate in a two week interval. Each single dose of the immunogen was 100 μg. The immune response was monitored by ELISA within 3 days after the third immunization.
Animals producing antisera with titers of at least 1
:
10
000 were used to obtain hybridomas with sp2/0 lines using standard protocols.17Antibody clones were characterized by ELISA (see below). Antibodies of selected clones were produced in ascites and purified on a protein G Sepharose column (LKB, Sweden).
Eight clones, namely, B1, B2, B11, C12, D3, F11, G4 and H1 were obtained.
Antibody testing by indirect enzyme-linked immunosorbent assay (ELISA)
To test the monoclonal antibodies, the C60–protein conjugate (0.5 μg ml−1) in PBS was added to Costar 9018 microplate wells (Corning, USA) and incubated for 16 h at 4 °C. The plate was washed four times with PBS containing 0.05% of Triton X-100 (PBST). Then a series of dilutions of the antibodies were added to the microtiter plate wells, and the incubation was carried out for 1 h at 37 °C. After washing, peroxidase-labeled goat anti-mouse antibodies were added to the wells (1
:
6000 dilution of the commercial preparation), and the incubation was carried out for 1 h at 37 °C. After final washing, the peroxidase activity of the resulting complexes was measured. For this purpose, a substrate solution containing 0.42 mM 3,3′,5,5′-tetramethylbenzidine and 1.8 mM hydrogen peroxide in 0.1 M sodium citrate buffer, pH 4.0, was used. Then the solution was added to each microplate well and the incubation was carried out for 15 min at room temperature. The enzymatic reaction was terminated by adding 50 μl of 1 M H2SO4 to each well. The optical density of the oxidation product was measured at 450 nm.
In preliminary experiments, the C60–protein concentrations for the immobilization on the microplate were varied from 0.02 to 5 μg ml−1.
The antibody titer was determined as the maximum antibody dilution, at which the interaction of antibodies with antigens in the above-described scheme can be reliably detected.9
Competitive enzyme-linked immunosorbent assay of fullerene C60 samples
To detect different fullerene forms, the chosen C60–protein conjugate was immobilized on microplate wells as described above. After washing the microplate wells, a series of dilutions of fullerene C60 samples and the monoclonal antibodies at chosen concentration were added to microplate wells and the incubation was performed for 90 min at 37 °C. After washing, the incubation with peroxidase-labeled goat-mouse rabbit antibodies and peroxidase activity measurement of the peroxidase activity of the resulting complexes were carried out as described above.
The plots of the optical density (y) versus the antigen concentration in the sample (x) were fitted to a four-parameter logistic function y = A2 + {A1 − A2/[1 + (x/x0)p]} using the Origin 7.5 software (OriginLab, USA), where A1 is the maximum signal amplitude, A2 is the minimum signal amplitude, p is the slope of the calibration curve, x0 is the antigen concentration causing 50% inhibition of the antibody binding (IC50). The antigen concentration resulting in 10% inhibition (IC10) was calculated by the following formula: IC10 = (1/9)1/p × x0 and then regarded as the detection limit of the assay.18
Intraperitoneal administration of rats with fullerene C60
Male Vistar rats weighing 160–200 g in two parallels were once intraperitoneally injected with a water dispersion of fullerene C60 (250 mg of fullerene C60 per animal) containing Tween 80 and carboxymethyl cellulose that increased the viscosity of the solution thus stabilizing it.19 For that purpose to 5 ml of a 0.9% NaCl solution containing 0.02% Tween 80, 0.1 g of carboxymethyl cellulose was added portionwise under vigorous stirring. The mixture was stirred for 1.5 h at room temperature, incubated at 4 °C overnight, and additionally stirred for 1 h at room temperature and for 1 h at 50 °C. Then the resultant hot solution was added to a glass vial containing 250 mg of fullerene C60. The vial was vigorously agitated until the formation of a uniformly black solution and then agitated for 30 min at 50 °C. After that it was sonicated for 20 min and sterilized by autoclaving.
Control animals were injected with the same solution without C60. Rats were killed under ether anesthesia at 3, 7, 14 or 28 days after administration and selected organs were excised. Liver, kidneys, brain, spleen, lungs and intestine were homogenized as described below.
Intragastric administration of rats with fullerene C60
Three groups of male rats weighing 160–200 g in six parallels (18 rats totally) were daily intragastrically administered with fullerene C60 in a freshly prepared 1% starch solution in three doses (0.5, 5 and 50 mg of fullerene C60 per kg). The volume of the injected solution did not exceed 3 ml per each animal. Control animals (18 rats totally) were administered with the same solution without fullerene C60. Rats were killed under ether anesthesia on 7, 18 or 30 days of administration and selected organs were excised. Liver, kidneys, brain, spleen, lungs and intestine were homogenized as described below.
For homogenization of organs of rats intraperitoneally and intragastrically administered with fullerene C60, an accurately weighted amount of biological sample was chopped with a scalpel and placed into a plastic test tube with a flat bottom. Then 2 ml of PBS was added. The biological sample was homogenized on a T25 digital ULTRA TURRAX high-performance disperser with an S 25 N-18 G dispersing element (IKA Werke, Germany) for 1 min at 16
000 rpm at room temperature. As a result a stable dispersion of the biomaterial (without visible tissue fragments) was yielded. The homogenates were stored at −18 °C.
Biomatrix pretreatment
a) with concentrated
nitric acid
: the method described in ref. 20 with modifications was used. For that purpose 24 M nitric acid was added to a homogenate in a 4
:
3 ratio (v/v). The resultant mixture was stirred overnight at 37 °C. Then the reaction mixture was brought to pH 7.4 with 10 M KOH.
b) with the enzymatic complex: homogenates of biological samples were treated with Flavourzyme 500L, the enzymatic complex containing fungal proteases and peptidases. For that purpose a solution of Flavourzyme 500L (200 mg ml−1 in 50 mM sodium phosphate buffer, pH 7.0) was added to a homogenate (0.1 g of Flavourzyme 500L per one g of the homogenate). The resultant mixture was vigorously stirred and incubated for 16 h at 37 °C.
c) with
acetic acid
and
toluene
: the biomatrix was pretreated according to a procedure described in ref. 21 with modifications. Initially, 200 μl of glacial acetic acid were added to 100 μl of the homogenate. Then the mixture was stirred for 10 min and sonicated in an ultrasound bath for 20 min. After that 2 ml of toluene were added and the resultant mixture was vigorously stirred for 1 h at room temperature. The toluene fraction was collected and then 2 ml of toluene were added to the residue. The extraction was carried out three times. Finally, the collected toluene fractions were mixed and evaporated. The residue was dried in an oven for 2 h at 70 °C.
Fullerene was extracted by adding DMF to the lysate obtained in (a) and (b). The mixture was vigorously stirred and sonicated in an ultrasound bath for 1 h. Then the mixture was centrifuged for 5 min at 1500 g at room temperature. The supernatant was collected and filtered through a 0.2 μm filter (Millipore, USA). The dry residue obtained in (c) was dissolved in 200 μl of DMF and filtered through a 0.2 μm teflon filter. The equal volume of 5 mM PBS was added to a C60 solution in DMF before the analysis.
Results and discussion
Preparation of specific reagents
Conjugates of the fullerene carboxylic derivative, (1,2-methanofullerene C60)-61-carboxylic acid (see structure (c) in Fig. 1), with porcine thyroglobulin (PTG), soybean trypsin inhibitor (STI) and bovine serum albumin (BSA) were synthesized by the carbodiimide activation of the hapten derivative followed by the interaction of activated hapten with protein amino groups. The initial hapten to protein molar ratios were 100
:
1 for PTG, 20
:
1 for BSA and 10
:
1 for STI in accordance with the molar weight of protein carriers (and, consequently, the quantity of reactive groups and the hapten loading capacity). The UV-Vis spectra of the conjugates show a peak at 330 nm. The hapten to protein molar ratios were determined from the optical densities of the fullerene carboxylic derivative and the fullerene–protein conjugates. These values were calculated and given in ref. 15. They were 36
:
1, 9
:
1 and 2
:
1 for C60–PTG, C60–BSA and C60–STI, respectively. Solutions of the C60–protein conjugates were stable for at least one year.
Antibody production and testing
After immunization eight clones of antibodies were obtained. The antibody titers characterized by indirect ELISA varied from 0.5 to 1000 μg L−1 depending on the clone and the C60–protein conjugate adsorbed on the solid phase. The typical titration curves for monoclonal antibodies are given in Fig. 2A. The fact that no binding of the obtained antibodies to the adsorbed 2,4-D–protein conjugates (see curves (d), (f) in Fig. 2A) as well as to the unmodified immobilized proteins (curves (c), (e) in Fig. 2A) was observed confirmed that antibodies are specific to fullerene but not to the structure of the protein carrier coupled with fullerene or to the bridge between the fullerene hapten and the protein molecule. It should be noted that the chosen 2,4-D hapten–protein conjugates were synthesized by the same carbodiimide/succinimide method16 and have the structurally similar linkage group between the hapten and the carrier protein (see structures (a), (b) in Fig. 1). No binding of non-specific monoclonal antibodies (antibodies to ochratoxin A, chloramphenicol, plum pox virus, potato virus X) with immobilized C60–protein conjugates was detected (Fig. 2B). This is evidence that immunoglobulins are not absorbed on the fullerene C60 cage which was observed for some proteins, such as serum albumins and HIV protease,13,14,22 but recognize and specifically bind fullerene.
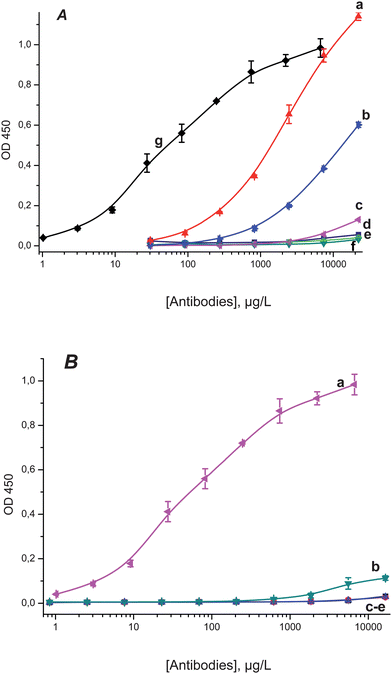 |
| Fig. 2 A: Titration curves for anti-C60 antibodies: (a) clone H1 on immobilized C60–PTG; (b) clone B1 on immobilized C60–PTG; (c) clone B1 on immobilized PTG; (d) clone H1 on immobilized 2,4-D-PTG; (e) clone H1 on immobilized PTG; (f) clone B1 on immobilized 2,4-D-PTG; (g) clone C12 on immobilized C60–STI. B: titration curves for monoclonal antibodies: (a) clone C12 of anti-C60 antibodies on immobilized C60–STI; (b) against ochratoxin A, (c) against chloramphenicol, (d) against plum pox virus, (e) against potato virus X, all on immobilized C60–PTG. | |
Enzyme immunoassay of fullerene C60 conjugated forms
In the first step of competitive ELISA development, protein-bound fullerene C60 that is assumed to be formed after fullerene administration to living organisms13,14 was detected. In this assay, the C60–protein conjugate adsorbed on the solid phase and the C60–protein conjugate in solution (analyzed sample) interacted competitively with the anti-fullerene antibodies. Combinations of immobilized antigen-clone of antibodies pairs from the three synthesized C60–protein conjugates and eight clones of antibodies were tested. To optimize the assay (to decrease the detection limit) the mode of competitive interaction (the duration was varied from 30 to 120 min), as well as the type and concentration of the immobilized C60–protein conjugate (in a range of 0.1–5 μg ml−1) and the concentration of monoclonal antibodies (in a range of 20–800 μg L−1) were chosen. From the comparison of competitive curves the 90 min duration of the competitive step providing 73% of the maximum binding was chosen for ELISA. The 0.5 μg ml−1 concentration of all C60–protein conjugates was chosen for the immobilization; a further decrease in concentration of conjugates upon the adsorption on the solid phase led to a significant reduction of the signal (approximately 80% of the signal value compared to the signal at a concentration of 0.5 μg ml−1 for all C60–protein conjugates).
There were no interactions of anti-C60 monoclonal antibodies with solutions of protein carriers that did not contain the coupled hapten or contain the alternate hapten (2,4-D), which confirmed the specific nature of the antibody binding (see Fig. S-1 A as an example†).
The detection limits of competitive ELISA for all immobilized antigen–antigen in solution-clone of antibodies combinations were determined; the values not exceeding 20 μg L−1 are presented in Table S-1.†
It was shown that fullerene C60 in the conjugated form with STI can be determined with the best sensitivity using the clone H1 and the immobilized C60–PTG conjugate at a concentration of 0.5 μg ml−1 (Fig. S-1 A†). The limit of detection of fullerene C60 in the conjugates was estimated as 0.2 μg L−1 based on the C60–STI detection limit (3 μg L−1 by protein) and the hapten
:
protein ratio in this conjugate. It should be noted that in one and the same system the competition curves for different hapten–protein conjugates in solution gave similar detection limits calculated for coupled C60 (Fig. S-1 B†).
Enzyme immunoassay of water-soluble fullerene forms
Free unmodified fullerene is a hydrophobic compound insoluble in aqueous media. For this reason we first examined the possibility of detecting its water-soluble carboxylated derivative, the fullerene aminocaproic acid (C60(H)3(NH(CH2)5COONa)3) (see structure (d) in Fig. 1), by the competitive ELISA based on the obtained reactants. To reduce the detection limit, the assay conditions, such as the concentrations of immunoreagents, the ionic strength of the reaction medium and the detergent, were also optimized. Since fullerenes tend to coagulate upon the addition of electrolytes,14 we investigated the influence of the salt content in the reaction medium on the detection limit. For that purpose the competitive immune interaction was carried out both in a standard potassium phosphate buffer (50 mM, containing 100 mM NaCl, pH 7.4) and in phosphate buffers with the reduced KH2PO4/NaCl content (25 mM/50 mM, 5 mM/10 mM, 2 mM/4 mM, correspondingly, all at pH 7.4). All combinations were tested using Triton X-100 or Tween 80 as detergents. The lowest detection limit for the fullerene aminocaproic acid was achieved when PBST with the 5 mM/10 mM KH2PO4/NaCl content and Triton X-100 were used as the reaction medium and the detergent, respectively. This fact can be interpreted as the mutual influence of two factors. Immunoglobulins are unstable in solutions at a low ionic strength. A high ionic strength causes the aggregation of fullerene thus reducing the accessibility of the surface antigenic determinants for antibodies. All immobilized antigen–antibody combinations were tested and those providing the lowest detection limits were distinguished (values not exceeding 10 μg L−1 are presented in Table S-2†).
The best detection limit of 0.8 μg L−1 was achieved with the use of immobilized C60–STI and the clone B1 (Fig. S-2, curve a†). It is interesting that the H1 clone, which provided the best sensitivity in the case of protein–conjugated fullerene, does not have high specificity to the fullerene carboxylated derivative (the detection limit is about 2 μg ml−1).
No competitive interactions between the immobilized C60–protein conjugate and two control compounds – aminocaproic acid, NH2(CH2)5COOH, and the carboxylated derivative of atrazine (see structure (e) in Fig. 1) with anti-C60 monoclonal antibodies – were detected. Both these compounds contained the same –(CH2)5 moiety and the terminal carboxylic group as in the detected fullerene aminocaproic acid complex. This phenomenon is strong evidence that the obtained monoclonal antibodies are specific directly to the fullerene core but not to functional groups of its modified derivative.
Anti-fullerene antibodies also cross-react with hydroxylated fullerene, fullerenol, C60(OH)24. All immobilized antigen–antibody combinations were tested and those providing the lowest detection limits were distinguished (values not exceeding 10 μg L−1 are presented in Table S-3†). The best detection limit of fullerenol was to 0.9 μg L−1 for the case of immobilized C60–STI and the clone C12.
Enzyme immunoassay of free fullerene
It is known that, being non-polar compounds, free fullerenes are insoluble in an aqueous media and have very low solubility in polar solvents compatible with immunoassay, such as methanol, ethanol, and dimethyl sulfoxide.23,24 The reaction medium optimal both for immune interactions and the hydration of the reactants should be thoroughly selected in the case of non-polar antigens (e.g.surfactants,25,26 some pesticides,27,28 dioxins29–31). N,N-dimethylformamide (DMF) is known to have no effect on the immune properties of antibodies at low concentrations in the reaction medium.32 In addition, DMF is suitable for the solubilization of fullerene at low concentrations.24 We found that the long-term incubation of a fullerene C60 powder at a 50 μg ml−1 concentration in DMF under stirring and sonication resulted in the formation of a transparent light-brown solution stable for at least one year. Before the assay, the fullerene C60 solution in DMF was twice diluted with 5 mM PBS to keep the organic content at 50%. The dilution of an organic solution of fullerene C60 with standard 50 mM PBS led to the precipitation of the fullerene. Additional experiments showed that DMF diluted with PBS up to 12.5% did not reliably reduce the binding giving a 10% or less decrease in the binding value in ELISA (see Fig. S-3†). This allowed us to use DMF as a component of the reaction medium in the immunoassay procedure.
All immobilized antigen–antibody combinations were tested and those providing the lowest detection limits were distinguished (values not exceeding 100 μg L−1 are presented in Table S-4†). It should be noted that only the H1 clone can recognize free fullerene with a detection limit comparable to those for all the above-mentioned antigens. This value estimated by the developed ELISA using the H1 clone was 2 μg L−1.
To verify probable non-specific interactions between antibodies and the surface of a nanoparticle, we performed the control experiment with another nanoparticle (PAMAM dendrimer with the 1,4-diaminobutane core of generation 1.0). We revealed no competitive effect in this ELISA (Fig. 3). This fact additionally confirmed the specificity of the antibodies to fullerene C60.
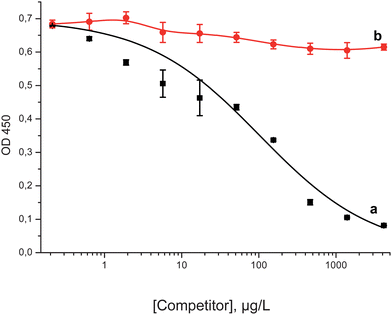 |
| Fig. 3 Competitive ELISA curves for fullerene C60 (a) and PAMAM dendrimer (b) for the clone H1 and immobilized C60–STI. | |
Besides, we carried out ELISA experiments for another class of carbon nanoparticles, multi-walled carbon nanotubes. It was shown that anti-fullerene antibodies cross-reacted with MWCNT; the detection limit was equal to 9 μg L−1 for the case of clone B1 (see Fig. S-4†). We suppose that such a high cross-reactivity can be explained by heterogeneity of the fullerenol sample we used. The amount of OH groups and their location on the surface of fullerene core may slightly differ from one molecule to another.
The cross-reactivity of the developed ELISA system with fullerene C70 solubilized in the same water–organic system was tested. It was shown that anti-C60 monoclonal antibodies can cross-react with this higher fullerene. The lowest detection limit for fullerene C70 was 5.5 μg L−1 with immobilized C60–PTG and the clone H1. Some similarity to the antibodies produced by Chen et al.10 should be mentioned here; the latter cross-reacted with the water-soluble conjugate of fullerene C70 and rabbit serum albumin.
Some characteristics of the best calibration curves for different competitors in ELISA of fullerenes were determined and summarized in Table 1.
Table 1 Characteristics of different competitors in ELISA of fullerenesa
Compound |
IC10, μg L−1 |
IC20–IC80, μg L−1 |
IC50, μg L−1nM−1 |
IC10 is the detection limit; IC50 is the antigen concentration causing 50% inhibition of the antibody binding; IC20–IC80 is the working range of detectable concentrations of antigen.
|
C60–STI |
0.2 (by C60) |
0.9–101 |
9.4/13.1 |
Fullerene aminocaproic acid |
0.8 |
2.9–230 |
25/18.4 |
Fullerenol
|
0.9 |
4.8–870 |
65/58 |
Fullerene C60 |
2.0 |
9.6–1100 |
100/139 |
Fullerene C70 |
5.5 |
2.0–2700 |
240/286 |
The coefficients of variation for the intra- and interassays (n = 3) for the estimated antigen concentrations in the working range of the assay were smaller than 10%.
It should be noted that all the above-mentioned antigens can be detected using the same immobilized antigen–antibody pair, namely, immobilized C60–STI and the C12 clone of antibodies.
Enzyme immunoassay of fullerene C60 in biological samples
The above-described results showed that the developed ELISA can be applied to the analysis of fullerene C60 in biological samples, e.g. in organs of rats administered with fullerene. According to investigations by Moussa et al.33fullerene undergoes in vivo metabolic transformations and tends to be changed after the in vivo administration. Various fullerene metabolites may have different IC50 values in the developed ELISA. Therefore, the quantitative determination of unmodified fullerene in organs and tissues is not quite correct and only qualitative regularities were studied.
To reduce the influence of the biomatrix on the assay results, a biomaterial should be pretreated before the detection of fullerene. Several techniques for the pretreatment of biological samples were tested. It should be noted that the influence of biomatrix components on the assay results is evident from the change in the form and the reduction of the amplitude of competition curves due to some non-specific binding effects. These effects were not eliminated by two of the proposed methods, e.g. by the treatment of homogenates neither with the enzymatic complex nor with nitric acid. It was evinced by the inhibition of the interaction between antibodies and the immobilized antigen by biological samples that did not contain fullerene C60. The extraction of fullerene C60 from homogenates with acetic acid and toluene followed by the evaporation and dissolution of the fullerene-containing extract in water–organic media used for the detection of free C60 proved to be the pretreatment of choice because it eliminates the above-mentioned influence of the matrix. In addition, this technique was more reproducible. The above procedure was employed for the pretreatment of all tested biological samples.
The ELISA procedure allowed the detection of fullerene C60 in biological samples (see Fig. 4A as an example). After single intraperitoneal administration, fullerene C60 was detected in liver and brain homogenates.
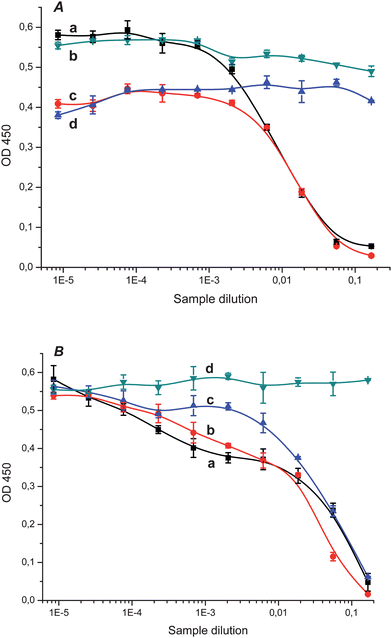 |
| Fig. 4 A. Competition curves for the ELISA detection of fullerene C60 in brain homogenate from rat intraperitoneally administered with fullerene at day 28 (a) and in control brain homogenate (b), in liver homogenate from rat intraperitoneally administered with fullerene at day 28 (c) and in control liver homogenate (d), all with clone H1 and immobilized C60–STI. B. Competition curves for the ELISA detection of fullerene C60 in brain homogenates from rat intragastrically administered with fullerene on days 7 (a), 18 (b) and 30 (c) (dose 50 mg kg−1) and in control brain homogenate (d) with clone H1 and immobilized C60–STI. | |
Fullerene C60 was found in liver, brain, and intestine of rats at days 7, 18 and 30 of intragastrical administration with different doses (0.5, 5 and 50 mg kg−1) of fullerene C60. The competition curves for the detection of fullerene C60 in extracts of brain homogenates at different days of administration (the dose was 50 mg kg−1) are presented as an example in Fig. 4B. The similarity of the competition curves obtained at different days of administration of animals with fullerene may be evidence of the slow fullerene elimination from the organism.
Conclusions
In the present study, monoclonal antibodies against fullerene C60 were produced. The interaction between anti-C60 antibodies and different fullerene derivatives was studied by ELISA. The experimental results showed that the developed ELISA allows the detection of modified and, for the first time, of free fullerene C60 in solutions and homogenates of rat organs. These results open opportunities for applying the produced monoclonal antibodies and the developed approach to toxicological studies, environmental monitoring and technological control of the fullerene production.
List of abbreviations used
Acknowledgements
This work was financially supported by the Russian Federal Target Program “Development of Nanoindustry Infrastructure in the Russian Federation for 2008–2011”.
The authors are grateful to the staff of GLP vivarium of the Institute of Physiologically Active Compounds, Russian Academy of Sciences (Chernogolovka, Russia), Dr A.A. Martianov (M.V. Lomonosov Moscow State University) for the experimental work with rats and Prof. A.I. Yaropolov (A.N. Bach Institute of Biochemistry, Russian Academy of Sciences) for useful discussions.
References
- C. W. Isaacson, M. Kleber and J. A. Field, Environ. Sci. Technol., 2009, 43, 6463–6474 CrossRef CAS.
- R. Partha and J. L. Conyers, Int. J. Nanomedicine, 2009, 4, 261–275 CrossRef CAS.
- K. Tiede, M. Hassellöv, E. Breitbarth, Q. Chaudhry and A. B. Boxall, J. Chromatogr., A, 2009, 1216, 503–509 CrossRef CAS.
- A. Kahru and H. C. Dubourguier, Toxicology, 2010, 269, 105–119 CrossRef CAS.
- S. Zuin, C. Micheletti, A. Critto, G. Pojana, H. Johnston, V. Stone, L. Tran and A. Marcomini, Nanotoxicology, 2010, 2, 1–14 Search PubMed.
- X. R. Xia, N. A. Monteiro-Riviere and J. E. Riviere, J. Chromatogr., A, 2006, 1129, 216–222 CrossRef CAS.
- D. Bouchard and X. Ma, J. Chromatogr., A, 2008, 1203, 153–159 CrossRef CAS.
- J. R. Deye, A. N. Shiveley, S. A. Oehrle and K. A. Walters, J. Chromatogr., A, 2008, 1181, 159–161 CrossRef CAS.
-
The Immunoassay Handbook. Edited by Wild D. Elsevier: London, 2005 Search PubMed.
- B.-X. Chen, S. R. Wilson, M. Das, D. J. Coughlin and B. F. Erlanger, Proc. Natl. Acad. Sci. U. S. A., 1998, 95, 10809–10813 CrossRef CAS.
- B. C. Braden, F. A. Goldbaum, B.-X. Chen, A. N. Kirschner, S. R. Wilson and B. F. Erlanger, Proc. Natl. Acad. Sci. U. S. A., 2000, 97, 12193–12197 CrossRef CAS.
- B. F. Erlanger, B.-X. Chen, M. Zhu and L. Brus, Nano Lett., 2001, 1, 465–467 CrossRef CAS.
- H. Benyamini, A. Shulman-Peleg, H. J. Wolfson, B. Belgorodsky, L. Fadeev and M. Gozin, Bioconjugate Chem., 2006, 17, 378–386 CrossRef CAS.
- S. Deguchi, T. Yamazaki, S. A. Mukai, R. Usami and K. Horikoshi, Chem. Res. Toxicol., 2007, 20, 854–858 CrossRef CAS.
- O. D. Hendrickson, N. S. Fedyunina, A. A. Martianov, A. V. Zherdev and B. B. Dzantiev, J. Nanopart. Res., 2011, 13, 3713–3719 CrossRef CAS.
- B. B. Dzantiev, A. V. Zherdev, O. G. Romanenko and L. A. Sapegova, Int. J. Environ. Anal. Chem., 1996, 65, 95–111 CrossRef CAS.
- G. Köhler and C. Milstein, Nature, 1975, 256, 495–497 CrossRef.
- G. S. Sittampalam, W. C. Smith, T. W. Miyakawa, D. R. Smith and C. McMorris, J. Immunol. Methods, 1996, 190, 151–161 CrossRef CAS.
- F. Moussa, F. Trivin, R. Ceolin, M. Hadchouel, P. Y. Sizaret, C. Greugny, A. Fabre and H. Rassat, Fullerenes, Nanotubes and Carbon Nanostructures, 1996, 4, 21–29 CAS.
- R. D. O'Brien, Anal. Biochem., 1964, 7, 251–254 CrossRef CAS.
- F. Moussa, M. Pressac, E. Genin, S. Roux, F. Trivin, A. Rassat, R. Céolin and H. Szwarc, J. Chromatogr., B, 1997, 696, 153–159 CrossRef CAS.
- S. H. Friedman, D. L. DeCamp, R. Sijbesma, G. Srdanov, F. Wudl and G. L. Kenyon, J. Am. Chem. Soc., 1993, 115, 6506–6509 CrossRef CAS.
- R. S. Ruoff, D. S. Tse, R. Malhotra and D. C. Lorents, J. Phys. Chem., 1993, 97, 3379–3383 CrossRef CAS.
- K. N. Semenov, N. A. Charykov, V. A. Keskinov, A. K. Piartman, A. A. Blokhin and A. A. Kopyrin, J. Chem. Eng. Data, 2010, 55, 13–36 CrossRef CAS.
- A. A. Mart'ianov, A. V. Zherdev, S. A. Eremin and B. B. Dzantiev, Int. J. Environ. Anal. Chem., 2004, 84, 965–978 CrossRef CAS.
- A. A. Mart'ianov, B. B. Dzantiev, A. V. Zherdev, S. A. Eremin, R. Cespedes, M. Petrovic and D. Barcelo, Talanta, 2005, 65, 367–374 CrossRef CAS.
- J. Horacek and P. Skladal, Anal. Chim. Acta, 2000, 412, 37–45 CrossRef CAS.
- M. A. Gonzalez-Martinez, A. Maquieira and R. Puchades, Int. J. Environ. Anal. Chem., 2003, 83, 633–642 CrossRef CAS.
- Y. Sugawara, S. J. Gee, J. R. Sanborn, S. D. Gilman and B. D. Hammock, Anal. Chem., 1998, 70, 1092–1099 CrossRef CAS.
- G. M. Shan, W. R. Leeman, D. W. Stoutamire, S. J. Gee, D. P. Y. Chang and B. D. Hammock, J. Agric. Food Chem., 2000, 48, 4032–4040 CrossRef CAS.
- T. Scharnweber, M. Fisher, M. Suchanek, D. Knopp and R. Niessner, Fresenius J. Anal. Chem., 2001, 371, 578–585 CrossRef CAS.
- Y. I. Melnikova, S. G. Odintsov, Z. I. Kravchuk and S. P. Martsev, Biochemistry, 2000, 65, 1256–1265 CAS.
- F. Moussa, S. Roux, M. Pressac, E. Genin, M. Hadchouel, F. Trivin, A. Rassat, R. Ceolin and H. Szwarc, New J. Chem., 1998, 22, 989–992 RSC.
Footnote |
† Electronic supplementary information (ESI) available. See DOI: 10.1039/c1an15745k |
|
This journal is © The Royal Society of Chemistry 2012 |
Click here to see how this site uses Cookies. View our privacy policy here.