DOI:
10.1039/C1FO10089K
(Paper)
Food Funct., 2012,
3, 40-48
Correlation of the Taq1 dopamine D2 receptor gene and percent body fat in obese and screened control subjects: A preliminary report†
Received
30th May 2011
, Accepted 28th September 2011
First published on 3rd November 2011
Abstract
While there is a considerable body of literature correlating the role of dopaminergic genes and obesity, body mass index, body type, overeating, carbohydrate binging, energy expenditure and low dopamine D2 receptor (D2R) receptor density, there is a paucity of research concerning the dopamine D2 receptor gene (DRD2) variants and percent body fat. We report here the potential association of DRD2 genotypes and the percent fat phenotype. In this study we genotyped 122 obese/overweight (O/OW) Caucasian subjects and 30 non-obese Caucasian controls, screened to exclude substance abuse. The subjects were assessed for weight, body mass index (BMI; kg m−2) and percent body fat using dual energy X-ray absorptiometry (DEXA). The sample was separated into two independent groups; those with the Taq1 A1 allele (A1/A1 or A1/A2) and those without the A1 allele (A2/A2). The controls had a normal range of body fat (25–31% for females and 18–25% for males). The O/OW subjects had a percent body fat value of over 32% for females and over 25% for males. For the O/OW subjects, the mean BMI was 29.3 ± 6.25 kg m−2, mean body fat was 42.1 ± 7.5% and mean weight was 82.7 ± 21.7 kg. The DRD2Taq1 A1 allele was present in 67% of the O/OW subjects compared to 3.3% of super controls (A group), 33.3% of screened (for drug abuse and obesity) controls (B group) and unscreened literature controls 29.4% (P ≤ 0.001). Comparing all cases with more than 34% body fat, utilizing logistic regression analysis, the DRD2 A1 allele accounts for 45.9% of the variance, which is statistically significant (χ2 = 43.47, degrees of freedom (df) = 1, P < 0.0001). These results are consistent with a role for the DRD2 gene in obesity, as measured by percent body fat as well as by weight and BMI.
Introduction
Overeating is a biogenetic condition that comes in many forms1 and is inheritable.2–8 While there are certain environmental factors which may reduce body fat, body mass and fat-free mass in both adults and children, including but not limited to, low-intensity, long-duration exercise, aerobic exercise combined with high-repetition resistance training and exercise programs combined with a behavioral-modification component, genetics may even play a more significant role.9
Compulsive eating disorders are likely to involve more than one defective gene because of their complexity.10–17 Given the considerable array of metabolic systems that contribute to overeating, it is not surprising that a number of neurochemical defects have been implicated. It is important to realize that carbohydrates cause the release of the pleasure-inducing brain chemical dopamine. There is general agreement that other pleasure-inducing substances such as alcohol and nicotine, like glucose, work through the dopaminergic pathways of the brain. This shows the common genetic thread of multiple addictions.18 There have been a number of investigations that have observed a significant association between an abnormal form of a gene known as the dopamine D2 receptor gene, and obesity; time of onset of obesity; carbohydrate preference or craving; high body mass index; co-morbid drug abuse; energy expenditure; hyperphagia (overeating);19 and low dopamine D2 receptors.1 Since obesity and overeating including binging behavior is polygenic, many genes have been identified. Most of these genes are susceptibility genes. These genes are necessary, but not sufficient alone, to cause obesity.
In 1990, Blum and colleagues, using the Taq1 polymorphism (gene variant) of the dopamine D2 receptor gene locus (DRD2), for the first time reported a strong association between a virulent form of alcoholism and the minor gene form (A1) of the DRD2 gene in this population.20 Other studies further support an association of the A1 form of the DRD2 gene with substance abuse vulnerability and other compulsive behaviors.21 In this regard, the National Institute on Alcohol Abuse and Alcoholism recently reported data that strongly suggest that DRD2 is a susceptibility gene for substance abusers across multiple populations. Specifically, a haplotype block of 25.8 kb was highly associated with alcohol dependence and heroin addiction. Furthermore, a low number of dopamine D2 receptors suggests a hypodopaminergic function, as described by Eliot Gardner in a series of published works.22 When there is a paucity of dopamine receptors the person will be more prone to seek any substance (including glucose) or behavior that stimulates the dopaminergic system, as a form of self-healing. In this regard, we know that substances such as alcohol, cocaine, heroin, nicotine and glucose, as well as a number of behaviors like gambling and sex, preferentially release dopamine at the nucleus accumbens. In one study,1 striatal dopamine D2 receptor availability was significantly lower in ten obese subjects compared to normal non-obese controls. In these obese subjects body mass index (BMI) correlated negatively with the measures of D2 receptors; the individuals with the lowest D2 values had the largest BMI.
Table 1 illustrates the role of dopaminergic genes in obesity and related behaviors. While we are making some progress in attempting to understand the cerebral mechanisms underlying the behaviors that lead to pathological overeating and obesity, dopamine, a neurotransmitter that modulates rewarding properties of food, is likely to be involved. To test the hypothesis that obese/overweight (O/OW) individuals having a high percent body fat compared to non-obese controls also have a high presence of the dopamine D2A1 allele, we measured percent body fat and genotyped individuals for the presence or absence of the dopamine D2 receptor A1 allele.
Table 1 The role of dopaminergic pathways in obesity and related behaviorsa
Source: see reviews Blum et al.19,20
|
DRD2
Receptors and psychoactive
drugs
|
Certain anti-psychotic drugs increase feeding behavior and cause weight gain because they block dopamine D2 receptors and dopamine response |
Drugs that stimulate either dopamine D1and/or D2 receptors reduce overeating |
Dopamine
D2 receptors and
gene
|
Dopamine stimulators normalize overeating, body fat and blood glucose levels by increasing serum dehydroepiandrosterone (DHEA). |
There is evidence that BMI and even personality disorders (temperament) are directly correlated with the ability of dopamine to bind to its respective brain receptors. |
Certain dopamine gene mutations associate with BMI. |
The A1 form of the dopamine D2 receptor gene is associated with increase fat stores. |
The A1 form of the dopamine D2 receptor gene is associated with a high BMI. |
Using PET scans, obese individuals have been found to have fewer dopamine D2 receptors than lean controls. |
Glucose causes a release of dopamine at brain sites. |
Dopamine increases the release of glucose from liver cells (hepatocytes). |
Other
dopamine
genes
|
Other genes, such as the dopamine 4 receptor, were also associated with obesity and a high BMI. |
Both dopamine D1 and D2 receptors are linked to glucose metabolism and subsequent feeding behavior. |
Certain dopamine genes form associates with low energy expenditure. |
Research methods and procedures
This study was conducted with IRB approval of both the Path Research Foundation (registration #IRB00002334) and the City of Hope National Medical Center, and all patients filled out and signed an approved consent form prior to entering this study. The genotyping was performed at the Department of Medical Genetics at the City of Hope National Medical Center, Duarte, California. The recruitment and characterization of the obese subjects was accomplished at the Health and Medical Research Foundation, San Antonio, Texas and the Sports Medicine Institute, Baylor College of Medicine, Houston, Texas. The recruitment and characterization of the well-screened controls were accomplished at the PATH Medical Research foundation, New York City, New York. The statistical analysis was performed in part at the University of Texas School of Public Health, San Antonio, Texas.
Subjects
Controls.
In order to perform scientifically sound genetic association studies in a complex disease such as obesity, certain exclusion/inclusion criteria must be satisfied. Since it is now known that polymorphisms of the DRD2 gene (A1, B1, C1, the haplotype In6-Ex7 and other variants) are associated with a number of impulsive-addictive/compulsive disorders including severe alcoholism, polysubstance dependence, crack/cocaine dependence, smoking, obesity (BMI over 25), carbohydrate binging, conduct disorder, defense-style personality, schizoid/avoidant personality, violent crimes, pathological gambling, autism, Tourette syndrome, attention deficit disorder with or without hyperactivity (ADHD), severe withdrawal, depression, parental history of alcoholism, drug abuse, obesity, and inability to cope with anxiety; we decided to develop stringent non-Hispanic Caucasian controls.19,23 It is well known that there is a high rate of genetic polymorphisms in different ethnic groups (e.g. Hispanic, African Americans) thus it seemed parsimonious to carefully stratify accordingly. Specifically, these included careful assessment of alcoholism, substance use disorder, family history of chemical dependence, obesity, nicotine dependence (smoking behavior), BMI over 25, carbohydrate binging, autism, Tourettes, ADHD, mood disorders, personality (novelty seeking) schizophrenia, movement disorders, migraine, pathological gambling and post-traumatic stress. Thus, we carefully stratified 184 individuals attending the Path Medical Clinic of New York City. These patients were stringently assessed to eliminate any impulsive, addictive (including carbohydrate binging behavior and obesity, as defined by BMI and scale weight) or compulsive behaviors including absence of both Axis 1 and Axis 2 diagnosis.
Computer analysis revealed a sub population that fit the above exclusion/inclusion criteria. This sub-population consisted of a total of 30 non-Hispanic Caucasian subjects (4 males and 26 females) with an average age of 44.8 (46.3% females and 43.4% male) recruited from the total patient population of the Path Medical Clinic in New York City. Both the BMI and body fat of the 30 controls was within the normal range (BMI normal range below 25 kg m−2; and having normal range of body fat between 25–31% for females and 18–25% for males). It is noteworthy that over a five year period, we studied a total of 1506 subjects (71% Caucasian, 1% black, 7% Hispanic, less than 1% Asian) attending the Path Medical Clinic utilizing DEXA, and found the average percent body fat to be 28.7.
Obese/overweight (O/OW) subjects.
A total of 130 unrelated non-Hispanic Caucasian O/OW subjects (BMI above 25 kg m−2 and percent body fat above 32%) were enrolled in the study, 122 (17 men and 105 women; mean (± S.E.) age, 42.3 years ± 8.8) of whom completed the testing (93.8%). Subjects were recruited from a variety of fitness and athletic clubs in San Antonio and Houston, Texas, by fitness instructors and sales personnel who provided information about the study to potential participants. In order to ensure compliance, the fitness instructors were paid to monitor the subjects as they progressed through the study to ensure that the subjects reported their physical activity levels and calorific intake, and completed the testing. All subjects were asked to consult with their personal physician before giving written informed consent. At the time of recruitment the selection of the subjects was completely randomized, without any knowledge of their DRD2 Genotype.
Dual energy X-ray absorptiometry
A number of studies have shown that DEXA can accurately measure fat and lean content of skeletal mass with a typical precision error for total body bone mineral content < 1%.24DEXA has also been shown to be a precise method for assessing body composition in obese and non-obese subjects. DEXA correlates highly with underwater weighing deuterium dilution and total potassium measurements. The reliability of DEXA makes it possible to monitor the effects of relatively short-term dietary restrictions and exercise on both regional and total body composition.
DEXA provides a three-compartment model of body composition: fat, lean tissue mass and bone mineral content. Measurements are made using a constant potential energy source at 78 kVp and a K-edge filter (cerium) to achieve a congruent, stable, dual-energy beam with effective energies of 40 to 70 keV. The unit performs a series of transverse scans moving from head to toe at 1 cm intervals; the area being scanned is approximately 60 × 200 cm. Data are collected for about 120 pixel elements per transverse, with each pixel approximately 5 × 10 mm in size. Total body measurements are completed in 10 to 20 min with a scan speed of 16 cm s−1, or in 20 min with a scan speed of 8 cm s−1. The rate value (ratio of low to high energy attenuation in soft tissue) ranges from 1.2 to 1.4.
Genotyping
A total of 152 subjects were genotyped for the dopamine D2 receptor gene (30 stringent controls subjects, group A and 122 O/OW subjects). All subjects were genotyped based on a neutral identification number and read without knowledge of the individual being typed.
Group B (105) control subjects, which had been previously genotyped in the laboratory of David Comings as part of a larger study (private communication), were screened for drug abuse and obesity. However, these controls were not screened for all reward deficiency syndrome (RDS) behaviors and were not screened with DEXA or genotyped in the present study.
Total genomic DNA was extracted from each coded blood sample and aliquots were used for polymerase chain reaction (PCR) analysis. The oligo-nucleotide primers, 5′-CCGTCGACCCTTCCTGAGTGTCATCA-3′ and 5′CCGTCGACGGCTGGCCAAGTTGTCTA-3′, were used to amplify a 310 base pair (bp) fragment spanning the polymorphic Taq1A site of the DRD2 gene. The D2A1 and D2A2 genotyping was performed by a PCR technique.20,25,26 PCR was performed in 30 μL reaction mixtures containing 1.5 mM MgCl2, 2 mM 2′-deoxynucleotide-5′-triphosphates (dNTPs) 0.5 μM primers, 1 μg of template DNA, 1.5 U of Taq polymerase (Boehringer Mannheim Corp., Indianapolis, IN), PCR buffer (20 mM tris-HCl (pH 8.4) and 50 mM KCl. After an initial denaturation at 94 °C for 4 min, the DNA was amplified with 35 cycles of 30 s at 94 °C, 30 s at 58 °C, and 30 s at 72 °C, followed by a final extension step of 5 min at 72 °C. The PCR product was digested with 5 U of Taq1 for 22 h at 65 °C for the Taq1A polymorphism. Digestion products were then resolved on a 3% agarose gel (5 V cm−1) containing 0.65 μg ml−1ethidium bromide. There were three DRD2Taq1A genotypes: 1) the predominant homozygote, A2/A2, which exhibits three restriction fragments of 180 and 130 bp; 2) the heterozygote, A1/A2, which exhibits three restriction fragments of 310, 180, and 130 bp; and 3) the rare homozygote A1/A1, which produces only the uncleaved 310 bp fragment.
Statistical analysis
Demographic, clinical, laboratory, interview and questionnaire data were coded and entered into a computer database. DRD2 allelic prevalence, obtained by personnel blind to the aforementioned information, was also coded and the two data sets were merged for analyses. t-Tests were used to compare interval data, and chi-squared statistics with Yates' correction for continuity,27 as appropriate, was used for group comparisons of ordinal and nominal values. We examined the relative role of the DRD2 gene in percent body fat. For the DRD2 gene we utilized univariate logistic regression analysis with percent body fat as the dependent variable and the DRD2A1 allele, sex, and age as independent variables.
The rationale for imitating these experiments relates to the unknown involvement in percent body fat as a function of DRD2 polymorphisms, especially the DRD2 A1 allele. Due to a remarkable list of behaviors known to associate with RDS (phenotype) we decided to utilize what we have referred to as “super-controls”. This is the first time any laboratory carefully selected out all subclass RDS behaviors to serve as a control group. We also decided to utilize logistic regression analysis to determine the percent contribution of the DRD2 A1 allele as a predictor of percent body fat.
Results
Baseline characteristics for the 30 controls are provided in Table 2. The age (mean ± S.E.) of the well-screened control individuals was 44.8 ± 7.1 years. The percent body fat (mean ± S.E.) for these subjects was 28.4 ± 3.4, the BMI (mean ± S.E.) for these subjects was 22.4 ± 2.9 kg m−2 and the scale weight (mean ± S.E.) for these controls was 61.3 ± 6.7 kg.
Table 2 Mean (SD) baseline demographic data and DRD2 A1 allele percent prevalence for 152 subjects
Subjects (n) |
Age (years) |
Weight (kg) |
Body fat (%) |
BMI (kg m−2) |
A1 allele (%) |
Source: Kaats et al., Current Therapeutic Research, 1998, 59(6), 379–388.
|
Control subjects A (n = 30) |
a44.8 ± 7.1 |
a61.3 ± 6.7 |
a28.4 ± 3.4 |
a22.4 ± 2.9 |
3.3 |
Control subjects B (n = 105) |
36.5 ± 6.9 |
60.5 ± 6.1 |
|
21.9 ± 2.9 |
33.4 |
O/OW Subjects (n = 122) |
a42.3 ± 8.8 |
a82.7 ± 21.7 |
a42.1 ± 7.5 |
a29.3 ± 6.25 |
67.0 |
Of the 130 subjects who were recruited for this study, only 8 failed to complete the final test: 1 subject became pregnant and was asked to withdraw from the study, 3 moved from the area, 1 was ill and 3 were lost to follow-up. Baseline characteristics for the 122 subjects who completed the study are provided in Table 2. The average age (years) of the O/OW participants was 42.3 ± 8.8; the mean percent body fat (%) for these subjects was 42.1 ± 7.5; mean BMI (kg m−2) was 29.3 ± 6.25 and the scale weight (kg) was 82.7 ± 21.7.
Using standard statistical analyses described above, we compared the “super” control (Group A) and O/OW groups with respect to age (A), scale weight (SW), percent body fat (BF) and BMI. The results obtained are as follows: A (P < 0.15), SW (P < 0.001), BF (P < 0.001), and BMI (P < 0.001) respectively (Fig. 1).
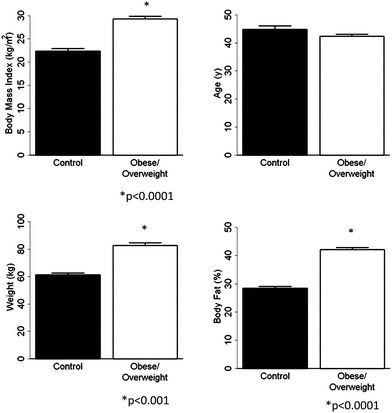 |
| Fig. 1 Data for age, percent body fat as measured by DEXA, BMI and scale weight in super control group A and O/OW populations. | |
Insert graphics
It is noteworthy that the genotyping results show that compared to non-abusing and non-obese literature controls (n = 3329) carrying the DRD2 A1 allele at 29.4%23 the defined 30 well-screened controls used in this study carried the DRD2 A1 allele at only 3.3%. However, the 122 O/OW subjects carried the DRD2 variant at 67%. Chi square analysis revealed a significant association with percent body fat compared to 30 super controls, (χ2 = 38.5, df = 1, P < 0.0001) (Fig. 2). Fig. 2 also shows the positive association of two other control data sets: 3329 unscreened population controls (at least P < 0.0001) and 105 subjects (Control B) (at least p < 0.0001). Even more importantly and surprisingly, comparing all cases with more than 34% body fat utilizing logistic regression analysis, the DRD2 A1 allele accounts for 45.9% of the variance which is statistically significant (χ2 = 43.47, df = 1, P < 0.0001.
 |
| Fig. 2 Percent presence of Taq1 dopamine D2 receptor A1 allele in population of unscreened controls (n = 3329); screened controls group B (n = 105); super screened controls group A (n = 30) and obese/overweight subjects (n = 122). | |
Discussion
While there is increasing evidence that genetic factors can influence differences in vulnerability to obesity, there still is controversy as to the exact genetic mechanism. Dopamine, acting through many receptors, can modulate the activity of neuronal reward pathways and thus effect a number of impulsive, addictive and compulsive behaviors, including overeating and carbohydrate binging. Over the last decade, a number of genetic variants have been associated with obesity and related substrates. Included in this list are CNS regulatory genes such as the OB (LEP) gene and the dopamine D2 receptor gene. In this regard a number of studies1,19,20 (see Table 1) have reported a positive association of the Taq1 dopamine D2 receptor A1 allele and low density of D2 receptors in obesity, carbohydrate binging, parental history of obesity, comorbid substance use disorder (SUD) and energy expenditure.
There are a number of plausible mechanisms that may contribute to these phenomena. The potential mechanisms involve: (1) the role of the DRD2 gene polymorphisms in obesity and related behaviors; (2) the role of the DRD2 mutations and energy expenditure; (3) the interaction of glucose and dopamine release and hyperphagia; (4) the role of dopamine and hyperglycemia; (5) the importance of using “super” normal controls in association studies; and (6) the relationship between personality, body type and dopaminergic genes.
In this population we found a very small number of individuals that carried the A1/A1 genotype in the O/OW group (7/122) thereby not allowing any meaningful further statistical analysis of the data set. It is important to note that in association studies the phenomena known as heterosis may have impact on the interpretation of understanding allelic meaning. Molecular heterosis occurs when subjects heterozygous for a specific genetic polymorphism show a significantly greater effect (positive heterosis) or lesser effect (negative heterosis) for a quantitative or dichotomous trait than subjects homozygous for either allele. At a molecular level heterosis appears counterintuitive to the expectation that if the 1 allele of a two-allele polymorphism is associated with a decrease in gene expression, those carrying the 1/1 genotype should show the greatest effect, 1/2 heterozygotes should be intermediate, and 2/2 homozygotes should show the least effect. For many association studies positive heterosis occurs often, especially for the DRD2 gene.
Role of the DRD2 gene polymorphisms in obesity and related behaviors
Specifically, the reinforcing properties of food have also led to an examination of the involvement of DRD2 polymorphisms in obesity. Haplotype 4 (GT) of intron 6 and exon 7 of the DRD2 gene was found to be associated with an increased risk of obesity.28 In another study, the DRD2 A1 allele was present in 45.2% of obese subjects,29 a prevalence similar to that found in alcoholics, nicotine- and other drug-dependent subjects. In addition, the A1 allele was significantly associated with carbohydrate craving. Polymorphisms of the OB gene and the DRD2 A1 allele are each associated significantly with obesity,16 these two polymorphisms together accounted for about 20% of the variance in BMI, particularly in younger women. Another study has ascertained the relationship of the DRD2 A1 allele in obese subjects with and without comorbid substance use disorders.30 In obese subjects, A1 allelic prevalence was significantly higher than in controls (P < 10−4). Moreover, the progressive increase in comorbid substance use disorders in these obese subjects was positively related to increased A1 allelic prevalence (P < 10−6). Furthermore, another case control study compared variants of the DRD2 gene in obese (BMI > 30) and non-obese control subjects.31 The DRD2 A1 allele was significantly higher in obese subjects compared to controls (P = 0.002) as was the DRD2 B1 allele (P = 0.003). The risk of obesity associated with the DRD2 A1 genotype was 3.48 compared to 4.55 for the DRD2 B1 genotype. Moreover, Thomas et al.32 assessed the Taq1A DRD2 alleles in 484 obese and 506 non-obese Chinese subjects. Obese subjects (using either BMI or waist-to-hip ratio criteria) had a significantly higher prevalence of the A1 allele (P = 0.02) and A1 allelic frequency (P = 0.03), than non-obese subjects. Finally, another study determined the association of an Nco1 polymorphism (C–T transition) in exon 6 of the DRD2 gene with hypertension.33 Subjects with the TT genotype had significantly higher systolic blood pressure than subjects with the CT genotype (P = 0.049). Moreover, subjects with TT genotype had significantly higher diastolic blood pressure than either subjects with the CT or CC genotype (P = 0.011). The importance of the latter finding relates to the fact that there is strong evidence from epidemiological studies of a positive relationship between increased body weight and hypertension.34
Role of the DRD2 mutations and energy expenditure
Two studies,35,36 assessed the role of other DRD2 mutations on weight and energy expenditure in Pima Indians. Individuals with a Cys-encoding allele had a higher BMI than those homozygous for the Ser311-encoding allele.35 and furthermore, total energy expenditure and 24 h resting energy expenditure were lower in homozygotes for the Cys311-encoding allele when compared to heterozygotes and homozygotes for the Ser311-encoding allele.36
The interaction of glucose and dopamine release and hyperphagia
Moreover, it is well known that pharmacological doses of the glucose analogue, 2-deoxyglucose (2DG), cause acute glucose deprivation and are associated with enhanced dopamine turnover in pre-clinical studies. In fact, lines of evidence indicate that a variety of metabolic stresses, including acute glucose deprivation are associated with dopamine release. Using PET, Adler et al.37 found that 2DG administration enhanced synaptic dopamine concentrations. The administration of 2DG is associated with significant striatal dopamine release even in healthy volunteers. This data is important because it further ties glucose levels to dopaminergic activity. Moreover, there is even a relationship between insulin levels and dopamine release in the tuberoinfundibular neurons. The insulin effect is dependent on Ca2+ ions and a protein kinase C Na+ – H+ exchange system. Additionally, when there is lowered glucose in the brain leading to cerebral global transient ischemia, monoamine release, especially dopamine, is inhibited. In this regard, Trugman et al.38 showed D1 antagonists lowered glucose utilization by 24–28% in the globus pallidus, entopeduncular nucleus, subthalmic nucleus, substania nigra and even the motor cortex, suggesting that stimulation of the D1 receptor by endogenous dopamine contributes to basal metabolism in these regions. In contrast both D1 and D2 agonists increase glucose utilization. These results suggest that feeding behavior is tied into the stimulation of both D1 and D2 receptors and provides metabolic evidence for the importance of D1 and D2 functional linkage in the brain, which relates to hyperphagia or overeating.
Role of dopamine and hyperglycemia
Furthermore, the direct effects of dopamine on glucose release from primary cultured rat hepatocytes were studied in Japan by Shiroyama et al.39 The authors investigated whether dopamine has any direct effect on glucose release from hepatocytes through the glycogenolytic and/or gluconeogenic pathways, and at the same time determined the main type of adrenergic receptor involved in glucose release. Dopamine caused the release of glucose which was inhibited by the beta blocker, propranolol. The authors conclude that dopamine has a direct effect on heptocytes, increasing glucose release via both glycogenolytic and gluconeogenic pathways, and is mediated by beta-adrenergic receptors.
The importance of using “super” normal controls in association studies
We believe that this is the first report that provides direct genetic evidence that the dopamine D2 receptor A1 allele (which has been associated with fewer D2 receptors in humans)1,40 is positively associated with increased percent body fat. We must caution against any false notions that the DRD2 gene alone causes fat storage, suggested by the high percent contribution found in this particular study. The process of fat storage and the role of genes is poorly understood, however, with confirming data, our findings point to a significant involvement of percent body fat in humans and dopamine functionality. It is plausible that utilizing “super” controls will be important for future association studies. In this regard, Neiswanger and Hill found a strong association of the D2 A1 allele and alcoholism.41 Hill suggested failures reported in the literature were due to poor assessment of controls. They recommend the appropriate use of “super” controls to more accurately assess a true phenotype, especially important when studying complex behavioral diseases.42 They also found evidence for linkage between the dopamine D2 receptor gene and severe alcoholism, early onset, physical dependence symptoms, and antisocial personality disorder.43 However, one caveat to the use of “super” controls may be that they are not appropriate as these individuals do not mirror the general population; thus these results should be regarded as preliminary and warrant significant additional research.
Relationship between personality, body type and dopaminergic genes
In a study by Yasuno44 from Japan, personality is a behavioral pattern, which differs among individuals. Fuemmeler et al.,45 categorized personality variants according to the concept of fundamental body types related to neurotransmitter genotypes. Several lines of evidence suggest that the central dopamine system may underlie the regulation of weight and personality trait. In this study, the authors examined the dopamine D2 receptor (D2R) binding together with BMI and personality trait on the temperament and character inventory in 16 subjects. The data demonstrate a significant relation between D2R binding in the amygdala, BMI and the personality trait of harm avoidance (this is in agreement with other work by Blum et al. showing significant association between D2RA1 variants and harm avoidance46). The authors conclude that variation of dopaminergic activity in the amygdala underlies the personality variants related to body type.47
It is noteworthy that family-based treatments show positive relationships between parent and child weight losses. Epstein et al.48 showed a relationship between parent and child zBMI change over 6 and 12 months (rs = 0.69, 0.77, ps < 0.001), and concordance for the number of Taq1 A1 alleles predicted the similarity in parent and child weight loss at 6 (P = 0.003) and 12 (P = 0.025) months. These results show concordance of the Taq1 A1 allele of the DRD2 between parents and children may be one mechanism for the similar response to family-based treatments within families.
Moreover certain D2 dopamine receptor Taq1A genotypes (A1/A1, A1/A2) have been associated with obesity and substance abuse. Barnard et al.49 hypothesized that their presence would be associated with reduced efficacy of dietary interventions in individuals with type 2 diabetes. In fact, the A1 allele was highly prevalent, occurring in 47% of white participants (n = 49), which was significantly higher than the 29% prevalence previously reported in non-diabetic whites (P = 0.01). As discussed earlier, ethnicity is an important factor in dietary intake potentially related to dopaminergic genotype. The A1 allele was found in 55% of black participants (n = 44). Black participants with A1 (+) genotypes had significantly greater mean body weight (11.2 kg heavier, P = 0.05) and greater intake of fat (P = 0.002), saturated fat (P = 0.01), and cholesterol (P = 0.02) compared with A2/A2 A1 (−) individuals. Among whites in the vegan group, A1 (+) individuals reduced fat intake (P = 0.04) significantly less than did A1 (−) individuals.
Interestingly, Simonen et al.50 studied frequencies of a polymorphism in exon 6 of the DRD2 gene for the T and C alleles in females, using a sports index to evaluate physical activity. The TT homozygote in White women had 29–38% lower sports index (F = 4.09, P = .023) and 27–33% lower work index (F = 6.23, P = .004) than the CC homozygotes and CT heterozygotes. The results suggest that DNA sequence variation in the DRD2 gene is associated with physical activity levels among white women. In a related study, Mathes et al.51 showed significantly greater dopamine concentrations in dorsal striatum and nucleus accumbens of running wheel (HR) mice compared to M16 (selected for obesity) and ICR (not selected for obesity) mice. Microarray analysis showed significant gene expression differences between HR and M16 compared to ICR mice in both brain areas, with changes revealed throughout the dopamine pathway including D1 and D2 receptors, associated G-proteins (e.g., Golf), and adenylate cyclase (e.g., Adcy5). The results suggest that similar modifications within the dopamine system may contribute to the expression of opposite phenotypes in mice, demonstrating that alterations within central reward pathways can contribute to both obesity and excessive exercise which may be a competing phenotype.
In terms of energy expenditure as discussed earlier, Epstein et al.52 observed that food reinforcement was greater in obese than in non-obese individuals, especially in obese individuals with the Taq1 A1 allele. Energy intake was greater for individuals high in food reinforcement and greatest in those high in food reinforcement with the Taq1 A1 allele. These data show that individual differences in food reinforcement may be important for obesity and that the DRD2 genotype may interact with food reinforcement to influence energy intake. These results are not surprising and are in agreement with Stice et al.53 Weaker activation of the frontal operculum, lateral orbitofrontal cortex, and striatum in response to imagined intake of palatable foodsversus imagined intake of unpalatable foods or water, predicted future increases in body mass for those with the DRD2Tac IA A1 allele or the DRD4-7R allele. Data also suggest that for those lacking these alleles, greater responsivity of these food reward regions predicted future increases in body mass. Accordingly, this novel prospective functional MRI (fMRI) study indicates that responsivity of reward circuitry to food increases risk for future weight gain, but that genes that impact dopamine signaling capacity moderate the predictive effects, suggesting two qualitatively distinct pathways to unhealthy weight gain based on genetic risk. Finally, Heber and Carpenter54 suggested, upon review of the literature, that there is increasing evidence that the same brain reward circuits involved in perpetuating drug abuse are involved in the hedonic urges and food cravings observed clinically in overweight and obese subjects. A polymorphism of the D2 dopamine receptor which renders it less sensitive to dopamine stimulation has been proposed to promote self-stimulatory behavior such as consuming alcohol, abusing drugs, or binging on foods. It is important to determine how this polymorphism may interact with other well-known candidate genes for obesity, including polymorphisms of the leptin receptor gene and the opiomelanocortin gene. The complex interaction of poly-genes and even control of glucose induced dopamine release regulated by second messengers provide a novel mechanism. In fact, Fulton et al.55 recently demonstrated the ability of D2-AR activation to increase Kv1.2 currents in co-transfected cells and its reliance on Gβγ subunit signaling along with the physical coupling of D2-AR and Kv1.2-containing channels in striatal tissue.
Conclusions
In summary, we propose that while obesity is a polygenic disorder, our findings indicate a putative important role of the dopamine D2 receptor gene in morbid obesity, especially in high risk populations.17,35 We therefore propose that these and other findings will have important therapeutic implications, but at this stage the present results should remain as a preliminary observation. While further confirming the work of Comings and associates16 who found significant association of the DRD2A1 allele and body mass index accounting for only 2.8 percent of the variance, our surprisingly high value of contribution to the variance 45.5% percent body fat may support the use of more stringent control selection in subsequent studies and warrants further rigorous research.
References
- G. J. Wang, N. D. Volkow, J. Logan, N. R. Pappers, C. T. Wong, W. Zhu and N. Netusil, Brain dopamine and obesity, Lancet, 2001, 357, 354–57 CrossRef CAS.
- H. H. Males, M. C. Neale and L. J. Eaves, Genetic and environmental factors in relative body weight and human adiposity, Behav. Genet., 1997, 27, 325–351 CrossRef.
- K. M. Rose, B. Newman, E. J. Mayes-Davis and J. V. Selby, Genetic and behavioral determinants of waist-hop ratio and waist circumference in women twins, Obesity Res., 1998, 6, 382–392 Search PubMed.
- D. G. Carey, T. V. Ngugen, L. V. Campbell, D. J. Chisholm and P. Kelly, Genetic influences on central abdominal fat: a twin study, Int. J. Obes. Relat Metab Disord, 1996, 20, 722–726 CAS.
- T. Rice, E. W. Daw, J. Gagnon, C. Bouchard, A. S. Leon, J. S. Skinner, J. H. Wilmore and D. C. Rao, Familial resemblance for body composition measures: the HERITAGE Family Study, Obes. Res., 1997, 5, 557–562 Search PubMed.
- K. Schousboe, P. M. Visscher, B. Erbas, K. O. Kyvik, J. L. Hopper, J. E. Henriksen and B. L. Heitmann, Twin study of genetic and environmental influences on adult body size, shape, and composition, Int. J. Obes., 2004, 28, 39–48 CrossRef CAS.
- T. L. Nelson, D. T. Brandon, S. A. Wiggins and K. E. Whitfield, Genetic and environmental influences on body-fat measures among African–American twins, Obesity, 2002, 10, 733–739 CrossRef.
- Y. C. Chagnon, J. H. Wilmore, I. B. Borecki, J. Gagnon, L. Perusse, M. Chagnon, G. R. Collier, A. S. Leon, J. S. Skinner, D. C. Rao and C. Bouchard, Associations between the Lepton Receptor Gene and adiposity in middle-aged Caucasian males from the HERITAGE Family Study, J. Clin. Endocrinol. Metab., 2000, 85, 29–34 CrossRef CAS.
- L. M. LeMura and M. T. Maziekas, Factors that alter body fat, body mass, and fat-free mass in pediatric obesity, Med. Sci. Sports Exercise, 2002, 34, 487–496 Search PubMed.
- L. Perusse, Y. C. Chagnon, S. J. Weisnagel, T. Rankinen, E. Snyder, J. Sands and C. Bouchard, The human obesity gene map: the 2000 update, Obesity, 2001, 9(2), 135–69 CrossRef CAS.
- D. Evans, A. M. Wolf, U. Nellessen, S. Ahle, B. Kortner, H. W. Kuhlmann and U. Beisegel, Association between polymorphisms in candidate genes and morbid obesity, Int. J. Obes., 2001, 25(suppl 1), S19–S21 CrossRef CAS.
- C. Garenc, L. Perusse, Y. C. Chagnon, T. Rankinen, J. Gagnon, I. B. Borecku, A. S. Leon, J. S. Skinner, J. H. Wilmore, D. C. Rao and C. Bouchard, The hormone-sensitive lipase gene and body composition: the HERITAGE Family Study, Int. J. Obes., 2002, 26, 220–227 CrossRef CAS.
- K. Clement, C. Dina, A. Basdevant, N. Chastang, V. Pelloux, N. Lahlou, M. Berlan, D. Langin, B. Guy-Grand and P. Froguel, A sib-pair analysis study of 15 candidate genes in French families with morbid obesity: indication for linkage with islet 1 locus on chromosome 5q, Diabetes, 1999, 48, 398–402 CrossRef CAS.
- J. M. Fernandez-Real, C. Gutierrez, W. Ricart, R. Casamitjana, M. Fernandez-Castaner, J. Vendrell and J. Solar, The TNF-alpha gene Nco1 Polymorphism influences the relationship among resistance, percent body fat, and increased serum lepton levels, Diabetes, 1997, 46, 1468–1472 CrossRef CAS.
- J. Frey, M. Neuhauser-Berthold, S. A. Elis, S. A. Duncker, F. Rose, W. F. Blum, H. Remschmidt, F. Geller and J. Hebebrand, Lower serum lepton levels in female students of the nutritional science with eating disorders, Eur J Nutr, 2003, 42, 142–148 CAS.
- D. E. Comings, R. Gade, J. P. MacMurray, D. Muhlman, P. Johnson, R. Verde and W. R. Peters, Genetic variants of the human obesity (OB) gene: association with body mass index in young women, psychiatric symptoms and interaction with the dopamine D2 receptor (DRD2) gene, Molecular Psychiatry, 1996, 1, 325–335 Search PubMed.
-
K. Walder, R. A. Norman, R. L. Hanson, P. Schrauwen, M. Neverova, C. P. Jenkinson, J. Easlick, C. H. Waerden, C. Pecqueur, S. Raimbault, D. Ricqtier, M. H. Silver, A. R. Shuldiner, G. Solanes, B. B. Lowell, W. K. Chung, R. L. Leibel, R. Pratley and E. Ravussin, Association between uncoupling protein polymorphisms (UCP2–UCP3) and energy metabolism/obesity in Pima Indians, Human Molecular Genetics, 7, pp. 1431–1435 Search PubMed.
- K. Blum, J. G. Cull, E. R. Braverman and D. E. Comings, Reward Deficiency Syndrome, American Sci, 1996, 84, 132–145 Search PubMed.
- K. Blum and E. R. Braverman, Reward Deficiency Syndrome: a biogenetic model for the diagnosis and treatment of compulsive, addictive, and compulsive behaviors, J. Psychoact. Drugs, 2000, 32, 1–112 Search PubMed.
- K. Blum, E. P. Noble, P. J. Sheridan, A. Montgomery, T. Ritchie, P. Jagadeeswaran, H. Nogami, A. H. Briggs and J. B. Cahn, Allelic association of human D2 receptor gene in alcoholism, J Am Med Assn, 1990, 263, 578–580.
-
K. Xu, D. Lichterman, A. Roy, M. Roy, et al., Patterns of linkage disequilibrium on multiple populations support a role of a single haplotype block of the D2 dopamine receptor gene in substance abuse, American College of Neuropharmacology 42nd annual meeting, December 7–11th, San Juan, Puerto Rico, 2003, pp 70 (poster session abstract) Search PubMed.
-
E. L. Gardner, Brain Reward Mechanisms, in: Brain Reward Mechanisms in Substance Abuse: A comprehensive Textbook, J. H. Lowenson, P. Ruiz, R. B. Millman and J. G. Langford, ed., Williams & Wilkins, Baltimore, Maryland, 3rd edn, 1997 Search PubMed.
- E. P. Noble, D2 Dopamine Receptor Gene in Psychiatric and Neurological Disorders and its Phenotypes, Am. J. Med. Genet., 2003, 116B, 103–125 CrossRef.
- P. A. Tataranni and E. Ravussin, Use of dual-energy X-ray absorptiometry in obese individuals, Am J Clin Nutr, 1995, 62, 730–734 CAS.
- D. E. Comings, S. Wu, C. Chiu, R. H. Ring, R. Gade, C. Ahn, J. P. MacMurray, G. Dietz and D. Muhleman, Polygenic inhertance of Tourette Syndrome, stuttering, attention-deficit-hyperactivity, conduct and oppositional defiant disorder. The addictive and subtractive effect of the three dopaminergic genes-DRD2, DBH and DAT1, Am. J. Med. Genet., 1996, 67, 264–288 CrossRef CAS.
- D. E. Comings, R. Gade-Andavilu, N. Gonzalez, S. Wu, D. Muhlman, H. Blake, F. Chiu, K. Farewell, S. Darakjy, R. Baker, G. Dietz, G. Saucier and J. P. MacMurray, Multivariate analysis of associations of 42 genes in ADHD, ODD and conduct disorder, Clin. Genet., 2000, 58, 31–40 CAS.
-
S. Siegel, Nonparametric statistics for the behavioral sciences, McGraw Hill Book Co., New York, 1956, pp 104–111 Search PubMed.
- D. E. Comings, S. D. Flanagan, G. Dietz, D. Muhlman, E. Knell and R. Gysin, The dopamine D2 receptor (DRD2) as a major gene in obesity and height, Biochem. Med. Metab. Biol., 1993, 50, 176–185 CrossRef CAS.
- E. P. Noble, R. E. Noble, T. Ritchie, D. K. Grandy and R. S. Sparkes, D2 dopamine receptor gene and obesity, Int. J. Eating Disord., 1994, 15, 205–17 Search PubMed.
- K. Blum, E. R. Braverman, R. C. Wood, J. Gill, C. Li, T. J. H. Chen, M. Taub, A. R. Montgomery, J. G. Cull and J. P. Sheridan, Increased prevalence of the Taq1 A1 allele of the dopamine D2 receptor gene (DRD2) in obesity with comorbid substance use disorder, Pharmacogenetics, 1996, 6, 297–305 CrossRef CAS.
- M. R. Spitz, M. A. Detry and P. Pillow, et al., Variant alleles of the D2 dopamine receptor gene and obesity, Nutr. Res., 2000, 20, 371–380 CrossRef CAS.
- G. N. Thomas, B. Tomlinson and A. J. H. Critchley, Modulation of blood pressure and obesity with the dopamine D2 receptor gene Taq1 polymorphism, Hypertension, 2000, 36, 177–182 CAS.
- R. Rosemond, T. Rankinen and M. Chagnon,
et al., Polymorphism in exon 6 of the dopamine D2 receptor gene (DRD2) is associated with elevated blood pressure and personality disorders in men, J. Hum. Hypertens., 2001, 15, 553–558.
- G. N. Thomas, J. A. J. H. Critchley, B. Tomlinson, C. S. Cockram and J. C. N. Chan, Relationships between the Taq1 polymorphism of the dopamine D2 receptor and blood pressure in hyperglycemic and normoglycemic Chinese subjects, Clin. Endocrinol., 2001, 55, 605–611 CrossRef CAS.
- C. P. Jenkinson, R. Hanson, K. Cray, C. Wiedrich, C. Bogardus and L. Baiser, Association of dopamine D2 receptor polymorphisms Ser311Cys and Taq1 with obesity or type 2 diabetes mellitus in Pima Indians, Int. J. Obes., 2000, 24, 1233–8 CrossRef CAS.
- P. A. Tataranni, L. Baier and C. Jenkinson,
et al., Ser311Cys mutation in the human dopamine D2 gene is associated with reduced energy expenditure, Diabetes, 2001, 50, 901–904 CrossRef CAS.
- C. M. Adler, I. Elman, N. Weisenfield, L. Kestler, D. Pickar and A. Breier, Effects of acute metabolic stress on striatal dopamine release in healthy volunteers, Neuropsychopharmacology, 2000, 22, 545–50 CrossRef CAS.
- J. M. Trugman and C. L. James, Dopamine agonist and antagonist effects on regional cerebral glucose utilization in rats with intact dopaminergic innervation, Brain Res., 1993, 607(1–2), 270–4 CrossRef CAS.
- K. Shiroyama, K. Moriwaki and O. Yuge, The direct effect of dopamine on glucose release from primary cultured rat hepatocytes, In Vivo, 1998, 12(5), 527–9 Search PubMed.
- E. P. Noble, K. Blum, T. Ritchie, A. Montgomery and P. J. Sheridan, Allelic association of the D2 dopamine receptor gene with receptor-binding characteristics in alcoholism, Archives of General Psychiatry, 1991, 48(7), 648–54 Search PubMed.
- K. Neiswanger, S. Y. Hill and R. B. Kaplan, Association between alcoholism and the Taq1 A RFLP of the dopamine D2 receptor gene in samples of female and male alcoholics, Am. J. Med. Genet., 1995, 60, 267–271 CrossRef CAS.
- S. Y. Hill, Alternative strategies for uncovering genes contributing to alcoholism risk: unpredictable findings in a genetic wonderland, Alcohol, 1998, 16, 53–59 CrossRef CAS.
- S. Y. Hill, N. Zezza, G. Wipprecht, J. Locke and K. Neiswanger, Personality traits and dopamine receptors(D2 and D4): Linkage studies in families of alcoholics, Am. J. Med. Genet., 1999, 88, 634–641 CrossRef CAS.
- F. Yasuno, T. Suhara, M. Yamamoto, M. Inoue, Y. Okubo and K. Suzuki, Relation among dopamine D2 receptor binding, obesity and personality in normal human subjects, Neurosci. Lett., 2001, 300(1), 59–61 CrossRef CAS.
- B. F. Fuemmeler, T. D. Agurs-Collins, F. J. McClernon, S. H. Kollins, M. E. Kail, A. W. Bergen and A. E. Ashley-Koch, Genes implicated in serotonergic and dopaminergic functioning predict BMI categories, Obesity, 2008 Feb, 16(2), 348–55 Search PubMed.
- K. Blum, E. R. Braverman, J. G. Cull, T. J. Chen, J. Gill, R. Wood, A. Eisenberg, M. Sherman, K. R. Davis, D. Matthews, L. Fischer, N. Schnautz, W. Walsh, A. A. Pontius, M. Zedar, G. Kaars and D. E. Comings, Association of polymorphisms of dopamine D2 receptor (DRD2) and dopamine transporter (DAT1) genes with schizoid/avoidant behaviors (SAB), Mol. Psychiatry, 1997, 2(3), 239–46 CrossRef CAS.
- B. M. King, The rise, fall, and resurrection of the ventromedial hypothalamus in the regulation of feeding behavior and body weight, Physiol. Behav., 2006, 28;87(2), 221–44 CrossRef.
- L. H. Epstein, K. K. Dearing and R. W. Erbe, Parent-child concordance of Taq1 A1 allele predicts similarity of parent-child weight loss in behavioral family-based treatment programs, Appetite, 2010, 55(2), 363–6 CrossRef.
- N. D. Barnard, E. P. Noble, T. Ritchie, J. Cohen, D. J. Jenkins, G. Turner-McGrievy, L. Gloede, A. A. Green and H. Ferdowsian, D2 dopamine receptor Taq1A polymorphism, body weight, and dietary intake in type 2 diabetes, Nutrition, 2009, 25(1), 58–65 CrossRef CAS.
- R. L. Simonen, T. Rankinen, L. Pérusse, A. S. Leon, J. S. Skinner, J. H. Wilmore, D. C. Rao and C. Bouchard, A dopamine D2 receptor gene polymorphism and physical activity in two family studies, Physiol. Behav., 2003, 78(4–5), 751–7 CrossRef CAS.
- W. F. Mathes, D. L. Nehrenberg, R. Gordon, K. Hua, T. Garland Jr and D. Pomp, Dopaminergic dysregulation in mice selectively bred for excessive exercise or obesity, Behav. Brain Res., 2010, 11;210(2), 155–63 CrossRef.
- L. H. Epstein, J. L. Temple, B. J. Neaderhiser, R. J. Salis, R. W. Erbe and J. J. Leddy, Food reinforcement, the dopamine D2 receptor genotype, and energy intake in obese and non-obese humans, Behav. Neurosci., 2007, 121(5), 877–86 CrossRef CAS.
- E. Stice, S. Yokum, C. Bohon, N. Marti and A. Smolen, Reward circuitry responsivity to food predicts future increases in body mass: moderating effects of DRD2 and DRD4, NeuroImage, 2010, 1;50(4), 1618–25 CrossRef.
- D. Heber and C. L. Carpenter, Addictive Genes and the Relationship to Obesity and Inflammation, Mol. Neurobiol., 2011, 44(2), 160–165 Search PubMed.
- S. Fulton, D. Thibault, J. A. Mendez, N. Lahaie, E. Tirotta, E. Borrelli, M. Bouvier, B. L. Tempel and L. E. Trudeau, Contribution of Kv1.2 voltage-gated potassium channel to D2 autoreceptor regulation of axonal dopamine overflow, J. Biol. Chem., 2011, 18;286(11), 9360–72 CrossRef.
Footnote |
† Conflict of interest: Kenneth Blum and B. William Downs own stock in LifeGen, Inc. worldwide distributors of genetic testing. John Giordano is a LifeGen, Inc. Partner. |
|
This journal is © The Royal Society of Chemistry 2012 |