DOI:
10.1039/C1FO10209E
(Paper)
Food Funct., 2012,
3, 49-57
Inhibitory effects of garcinol and pterostilbene on cell proliferation and adipogenesis in 3T3-L1 cells
Received
23rd September 2011
, Accepted 27th October 2011
First published on 18th November 2011
Abstract
The aim of this work was to study the effects of garcinol and pterostilbene on cell proliferation and adipogenesis in 3T3-L1 cells. The results showed that garcinol and pterostilbene decreased the cell population growth and caused cell cycle arrest at the G2/M phase in 3T3-L1 preadipocytes. During adipocyte differentiation, both garcinol and pterostilbene had inhibitory effects on fat droplet formation and triacylglycerol accumulation. The data indicated that garcinol and pterostilbene could inhibit the glycerol-3-phosphate dehydrogenase (GPDH) activity by 97.8 and 61.5%, respectively, as compared to the control. Both garcinol and pterostilbene significantly attenuated the protein expressions of PPARγ and C/EBPα during 3T3-L1 adipocyte differentiation. Moreover, garcinol and pterostilbene caused an inhibition of lipid accumulation in the 3T3-L1 adipocyte differentiation phase. Garcinol and pterostilbene also significantly up-regulated the gene expression of adiponectin as well as down-regulated the gene expressions of leptin, resistin, and fatty acid synthase (FAS) in 3T3-L1 adipocyte differentiation. In 3T3-L1 adipocytes, garcinol significantly down-regulated the protein expressions of PPARγ and FAS as well as up-regulated the protein expressions of adipose triglyceride lipase (ATGL) and adiponectin. Garcinol also significantly up-regulated the gene expression of adiponectin as well as down-regulated the gene expressions of leptin and FAS. These results suggest that garcinol and pterostilbene have anti-adipogenic effects on preadipocytes and adipocytes.
Introduction
Obesity has become a global health problem due to its association with lifestyle-related diseases such as hyperlipidemia, hypertension, arteriosclerosis, type 2 diabetes mellitus, gall bladder disease, cancer, respiratory complications, and osteoarthritis.1,2 Wellen & Hotamisligil3 indicated that the excessive growth of white adipose tissue (WAT) is a potent risk factor for type 2 diabetes and cardiovascular disease. Adipocytokines are adipocyte-derived hormones, such as adiponectin and leptin, which modulate hepatic and peripheral lipid and glucose metabolism.4,5 The level of adiponectin in plasma is negatively correlated with body mass index (BMI), blood glucose, insulin, and triacylglycerols and positively correlated with the amount of high-density lipoprotein (HDL) cholesterol.6–8 The amount of leptin secreted in the adipose tissue is positively correlated with the lipid content and adipocyte size.9 The goal of obesity treatment is to reach and maintain a healthy body weight. In general, lifestyle changes, dieting, physical exercise and pharmacotherapy are the mainstays of treatment for obesity. However, some pharmacotherapies (such as sibutramine) have been withdrawn from the market based on US FDA warnings of serious side effects. Therefore, developments of potential natural compounds with slight or no side effects are very important for antiobesity agents.
Garcinol is a polyprenylated benzophenone derivative, obtained from Guttiferae (such as Garcinia indica, Garcinia cambogia, and Garcinia huillkensis) in tropical regions. The rind of the fruiting body of Garcinia indica has traditionally been used as culinary spice for foods. The rind of Garcinia plants also contains isogarcinol, hydroxycitric acid, hydroxycitric acid lactone, organic acid, polyphenols, carbohydrates, anthocyanin, and pigments.10 The phytochemistry and biology of Garcinia plants have been investigated for various uses, including treatment of inflammatory and infectious diseases as well as antioxidant, anti-carcinogenic, and anti-ulcer properties.11–13Pterostilbene is a naturally occurring compound and similar to the resveratrol found in grapes, wines, and berries.14 Recent studies have exhibited that pterostilbene possesses antioxidant, anti-inflammatory, and anti-cancer properties.15–17 However, few of these studies have focused on the anti-obesity effects of garcinol and pterostilbene on inhibition of adipogenesis in 3T3-L1 adipocytes.
The objective of this study was to investigate the effects of garcinol and pterostilbene on cell proliferation and adipogenesis in 3T3-L1 cells. In this study, 3T3-L1 preadipocytes, 3T3-L1 differentiation-phase adipocytes, and mature 3T3-L1 adipocytes were used to investigate antiproliferation and adipogenesis in vitro.
Materials and methods
Materials
Garcinol and pterostilbene were provided by Professor Chi-Tang Ho (Rutgers University, New Jersey, USA). MTT dye [3-(4,5-dimethylthiazol-2-yl)-2,5-diphenyltetrazolium bromide], propidium iodide (PI), oil red O, Triton®X-100, dexamethasone (DEX), 3-isobutyl-1-methylxanthine (IBMX), and insulin (INS) were obtained from Sigma (St. Louis, MO, USA). Dulbecco's modified Eagle's medium (DMEM), bovine calf serum, and antibiotic mixture (penicillin-streptomycin) were purchased from the Invitrogen Co. (Carlsbad, CA, USA). All other chemicals are reagent grade.
Cell culture
3T3-L1
preadipocytes (BCRC 60159) were purchased from the Bioresource Collection and Research Center (BCRC, Food Industry Research and Development Institute, Hsinchu, Taiwan, ROC). 3T3-L1 preadipocytes were planted into 10 cm dish and maintained in DMEM supplemented with 10% bovine calf serum, 1.5 g L−1sodium bicarbonate and 100 U mL−1penicillin-streptomycin, at 37 °C in a humidified 5% CO2 incubator. Adipocytic differentiation was induced by adipogenic agents (0.5 mM IBMX, 1 μM DEX and 1 μM INS) that were added to culture medium for eight days. Afterwards, the medium was placed to normal culture medium and was freshly replaced every 48 h. The cells were harvested at ten days from the initiation of the differentiation.
Analysis of cell viability
Cell viability was determined by MTT assay.18 Briefly, 3T3-L1 preadipocytes were seeded into 24-well plates. After 24 h of incubation, the cells were treated with the compound and incubated for further 0–72 h. Culture media were then removed and replaced with fresh media containing 0.5 mg mL−1 MTT and incubated for 2 h at 37 °C. The violet crystal was dissolved by DMSO and the optical density was measured at 570 nm using a BMG LABTECH FLUOstar (GmbH, Offenburg, Germany). The relative cell viability (percent) related to control wells containing a cell culture medium without samples was calculated as A570 (sample)/A570 (control) × 100. The final concentration of DMSO in the culture system was less than 0.1%, which had no significant effect on the cell growth.
The cells were plated into 10 cm culture dish at a density of 1 × 106cells/dish. The cancer cells stimulated with 10 μM of sample for 0–72 h were assayed for cell cycle progression and/or apoptosis by the PI staining method, as previously described.19 Briefly, cells were harvested, washed with PBS twice and fixed in 80% ethanol at 4 °C for 30 min, followed by incubation with 100 μg mL−1RNase for 30 min at 37 °C. The cells were stained with 40 μg mL−1PI for 15 min at room temperature and subjected to flow cytometric analysis of the DNA content using FACScan flow cytometry (Becton-Dickinson Immunocytometry Systems, San Jose, CA, USA). Approximately 1 × 104 counts were made for each sample. The percentage of the distribution of cell cycle phase and apoptosis were calculated by CELL Quest software.
Oil red O staining of 3T3-L1 adipocytes
Intracellular
lipid accumulation was measured using oil red O. The oil red O working solution was prepared as described by Ramirez-Zacarias et al.203T3-L1 adipocytes were harvested at ten days after the initiation of differentiation. 3T3-L1 cells were differentiated for ten days with or without garcinol (0–80 μM) and pterostilbene (0–150 μM). Cells were washed twice with phosphate buffered saline (PBS, pH 7.4) and then fixed with 10% neutral formalin for at least 20 min at room temperature. After the 10% neutral formalin was removed, 100% propylene glycol was added to each well for 3 min. Cells were decolorized with 60% propylene glycol before staining for 1 h with the oil red O working solution and then washed exhaustively with water. The morphological characteristics of lipid accumulation in cells were examined by microscopy (Olympus, Tokyo, Japan).
Triacylglycerol content
3T3-L1
adipocytes were harvested at ten days after the initiation of differentiation. Cells were collected and lysed in lysis buffer (1% Triton X-100 in PBS). The total triacylglycerol content in cells was determined using a commercial triacylglycerol assay kit (DiaSys Diagnostic Systems GmbH, Holzheim, Germany). The protein concentration was determined by using a BioRad DC protein assay kit (Bio-Rad Laboratories, Hercules, CA, USA).
Glycerol-3-phosphate dehydrogenase activity
3T3-L1
adipocytes were harvested at ten days after the initiation of differentiation. Cells were washed twice carefully with ice-cold PBS on 3T3-L1 adipocytes, and lysed in 25 mM Tris/1 mM EDTA, pH 7.5 for the measurement of glycerol-3-phosphate dehydrogenase (GPDH) specific activity. GPDH activity was determined using a commercial GPDH activity assay kit (B-Bridge, Mountain view, CA, USA). Protein concentration was determined by the BioRad DC protein assay kit (Bio-Rad Laboratories, Hercules, CA, USA) using bovine serum albumin as a standard.
Western blot assay
3T3-L1
adipocytes were harvested at ten days after the initiation of differentiation. Cells were collected and lysed in ice-cold lysis buffer [20 mM tris-HCl (pH 7.4), 2 mM EDTA, 500 μM sodium orthovanadate, 1% Triton X-100, 0.1% SDS, 10 mM NaF, 10 μg mL−1 leupeptin, and 1 mM PMSF]. The protein concentration was estimated with the Bio-Rad DC protein assay (Bio-Rad Laboratories, Hercules, CA, USA) using bovine serum albumin as a standard. Total protein (50–60 μg) was separated by sodium dodecyl sulfate-polyacrylamide gel electrophoresis (SDS-PAGE) using a 12% polyacrylamide gel. The proteins in the gel were transferred to a PVDF membrane. The membrane was blocked with 5% skimmed milk in PBST (0.05% v/v Tween-20 in PBS, pH 7.2) for 1 h. Membranes were incubated with primary antibody at 4 °C overnight and then with secondary antibody for 1 h. Membranes were washed in PBST for 10 min three times between each step. The signal was detected using the ChemiLucent ECL Detection System (Millipore, Billerica, MA, USA). The relative expression of proteins was quantified densitometrically using the software LabWorks 4.5 and calculated according to the reference bands of β-actin.
RNA
extraction and real-time RT-PCR
3T3-L1
adipocytes were harvested at ten days after the initiation of differentiation. Real-time RT-PCR was performed to determine the level of gene expression in cells. Total RNA from cells was isolated using the TRIzol method (Life Technologies, Rockville, MD, USA) according to the manufacturer's protocol. The cDNA was synthesized from the total RNA by reverse transcription PCR using a high-capacity cDNA reverse transcription kit (Applied Biosystems, Foster City, CA, USA) according to the manufacturer's instructions. The following primer pairs were used: leptin, 5′-CATCTGCTGGCCTTCTCCAA-3′ (forward) and 5′-ATCCAGGCTCTCTGGCTTCTG-3′ (reverse); adiponectin, 5′- GGAGATGCAGGTCTTCTTGG-3′ (forward) and 5′-TCCTGATACTGGTCGTAGGTGAA-3′ (reverse); FAS, 5′-TGGGTTCTAGCCAGCAGAGT-3′ (forward) and 5′-TACCACCAGAGACCGTTATGC-3′ (reverse); resistin, 5′-AGACTGCTGTGCCTTCTGGG-3′ (forward) and 5′-CCCTCCTTTTCCTTTTCTTCCTTG-3′ (reverse); GAPDH, 5′-TCAACGGCACAGTCAAGG-3′ (forward) and 5′-ACTCCACGACATACTCAGC-3′ (reverse). Relative real-time RT-PCR for detection of gene expression levels was carried out using an ABI 7300 real-time PCR system (Applied Biosystems, Foster City, CA, USA). The reaction mixture (total volume 25 μL) contained 1 × smart quant green master mix, 4.5 mM MgCl2, 100 nM forward primer, 100 nM reverse primer, cDNA and DEPC-H2O, as well as commercial reagents (Protech Technology, Taipei, Taiwan). The thermal profile was established according to the manufacturer's protocol: 95 °C for 10 min for enzyme activation, followed by denaturing at 95 °C for 15 s and annealing and elongation at 60 °C for 1 min, with a total of 40 cycles. Relative levels of gene expression were quantified using the ΔΔCt method, which results in a ratio of target gene expression relative to equally expressed housekeeping genes.
Statistical analysis
Each experiment was performed in triplicate. The results are expressed as mean ± SD. Statistical analysis was performed using SAS software. Analysis of variance was performed using ANOVA procedures. Significant differences (p < 0.05) between means were determined by Duncan's multiple range tests.
Results
Effects of garcinol and pterostilbene on cell viability and cell cycle in 3T3-L1 preadipocytes
To assess the inhibitory effects of garcinol and pterostilbene on the growth of 3T3-L1 preadipocytes, the cells were cultured for 24 and 72 h with or without test compounds (0–40 μM), and population growth was determined by MTT assay. The results showed that addition of garcinol or pterostilbene to the growth medium decreased the cell population growth of 3T3-L1 preadipocytes in a time- and dose-dependent manner (Fig. 1). Inhibition of cell population growth might be mediated through cell cycle arrest or cell cytotoxic effect. Cell cycle analysis of 3T3-L1 preadipocytes was performed by flow cytometry after being treated with 0–10 μM garcinol or pterostilbene for 24–72 h. As shown in Fig. 2, garcinol resulted in significant (p < 0.05) increase in cell population in the G2/M phase from 17.8% (24 h) to 30.5% (72 h) when the cells were treated with 10 μM garcinol. In pterostilbene treated cells, a significant (p < 0.05) increase in the number of cells in the G2/M phase from 19.1% (24 h) to 45.4% (72 h).
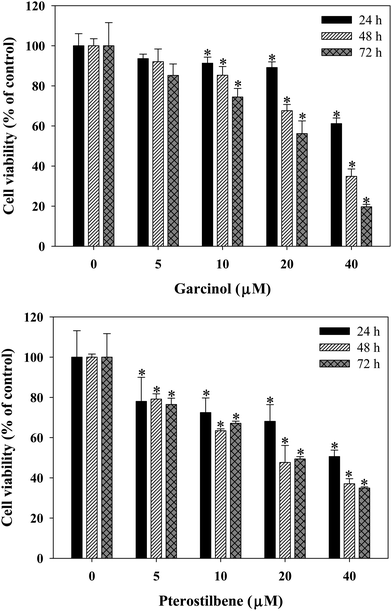 |
| Fig. 1 Effects of garcinol and pterostilbene on the cell viability of 3T3-L1 preadipocytes. Cells were treated with 0–40 μM of garcinol or pterostilbene for 0–72 h. Reported values are means ± SD (n = 3). *Significantly different from control (p < 0.05). | |
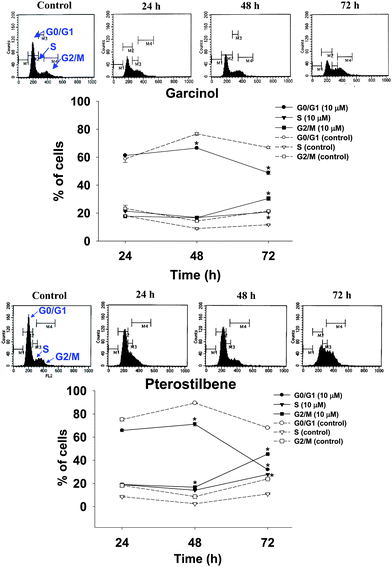 |
| Fig. 2 Effects of garcinol and pterostilbene on the cell cycle of 3T3-L1 preadipocytes. Cells were treated with 10 μM of garcinol or pterostilbene for 0–72 h. Reported values are means ± SD (n = 3). *Significantly different from control (p < 0.05). | |
Effects of garcinol and pterostilbene on intracellular triacylglycerol and GPDH activity in adipocyte differentiation of 3T3-L1 cells
Effects of garcinol and pterostilbene on the oil red O-stained material (OROSM), intracellular triacylglycerol and GPDH activity during 3T3-L1 adipocyte differentiation are shown in Fig. 3. Oil red O staining indicated that the treatment with 10 μM of garcinol or pterostilbene inhibited lipid accumulation in adipocyte differentiation of 3T3-L1 cells (Fig. 3A). Results also demonstrated that garcinol and pterostilbene at 10 μM caused the inhibition of intracellular triacylglycerol (Fig. 3B) and the inhibition of GDPH activity in the adipocyte differentiation of 3T3-L1 cellsFig. 3C.
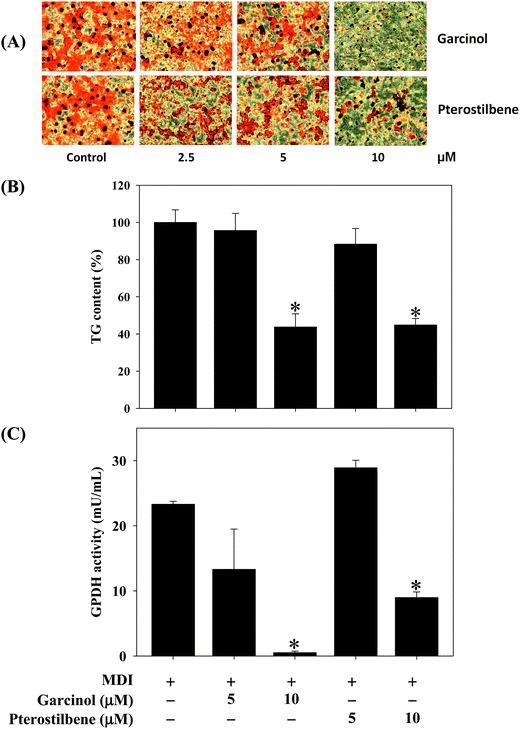 |
| Fig. 3 Effects of garcinol and pterostilbene on (A) OROSM, (B) intracellular triacylglycerol, and (C) GPDH activity during 3T3-L1 adipocyte differentiation. 3T3-L1 cells were differentiated for 10 days with or without garcinol and pterostilbene at 5 and 10 μM. Reported values are means ± SD (n = 3). *Significantly different from MDI (p < 0.05). MDI, methylisobutylxanthine/dexamethasone/insulin differentiate medium. | |
Effects of garcinol and pterostilbene on adipogenic protein and gene expressions in adipocyte differentiation of 3T3-L1 cells
Effects of garcinol and pterostilbene on protein expressions of CCAAT/enhancer binding protein (C/EBPα) and peroxisome proliferator-activated receptor (PPARγ) during 3T3-L1 adipocyte differentiation are shown in Fig. 4. Garcinol (0–10 μM) resulted in a significant decreased in C/EBPα and PPARγ during 3T3-L1 adipocyte differentiation. Moreover, treatment of 3T3-L1 adipocyte differentiation with 5 μM pterostilbene significantly decreased the protein expressions of C/EBPα and PPARγ. The effects of garcinol and pterostilbene on mRNA expressions of adiponectin, leptin, resistin and fatty acid synthase (FAS) during 3T3-L1 adipocyte differentiation are shown in Fig. 5. Treatment of 3T3-L1 adipocyte differentiation with garcinol (10 μM) or pterostilbene (10 μM) significantly increased the mRNA expression of adiponectin. Moreover, garcinol (1.25 μM) and pterostilbene (5 μM and 10 μM) significantly reduced leptin mRNA expression in 3T3-L1 adipocyte differentiation. Additionally, the mRNA expressions of resistin and FAS were remarkably decreased when garcinol (10 μM) or pterostilbene (5 μM and 10 μM) were added to 3T3-L1 adipocytes undergoing differentiation.
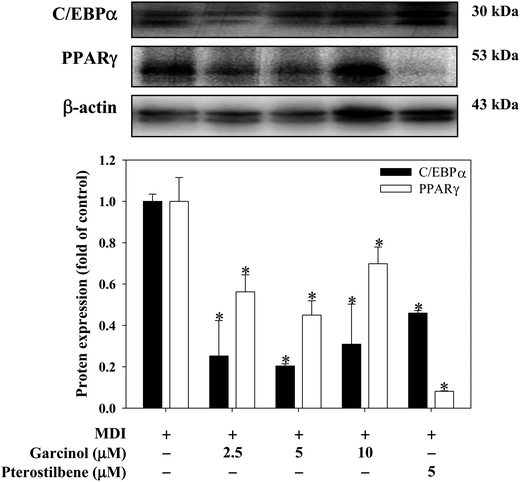 |
| Fig. 4 Effects of garcinol and pterostilbene on protein expressions of PPARγ and C/EBPα during 3T3-L1 adipocyte differentiation. 3T3-L1 cells were differentiated for 10 days with or without garcinol and pterostilbene. Reported values are means ± SD (n = 3). *Significantly different from MDI (p < 0.05). | |
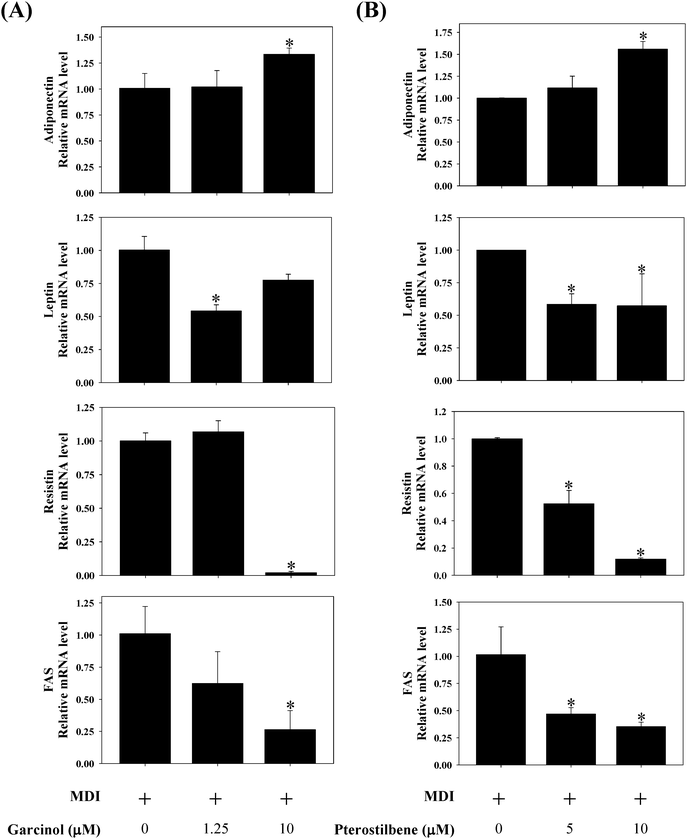 |
| Fig. 5 Effects of (A) garcinol and (B) pterostilbene on mRNA expressions of adiponectin, leptin, resistin, and FAS during 3T3-L1 adipocyte differentiation. 3T3-L1 cells were differentiated for 10 days with or without garcinol and pterostilbene. Reported values are means ± SD (n = 3). *Significantly different from control (p < 0.05). | |
Effects of garcinol and pterostilbene on cell viability and intracellular triacylglycerol in 3T3-L1 adipocytes
Effects of garcinol and pterostilbene on cell viability and lipid accumulation in 3T3-L1 adipocytes are shown in Fig. 6. The cell viability showed that cell numbers in 3T3-L1 adipocytes was not influenced by the treatment with garcinol (0–60 μM, 48–72 h) or pterostilbene (0–200 μM, 48–72 h) (Fig. 6A). The results demonstrated that garcinol (0–80 μM, 72 h) and pterostilbene (0–150 μM, 72 h) caused an inhibition of lipid accumulation in the 3T3-L1 adipocytes (Fig. 6B).
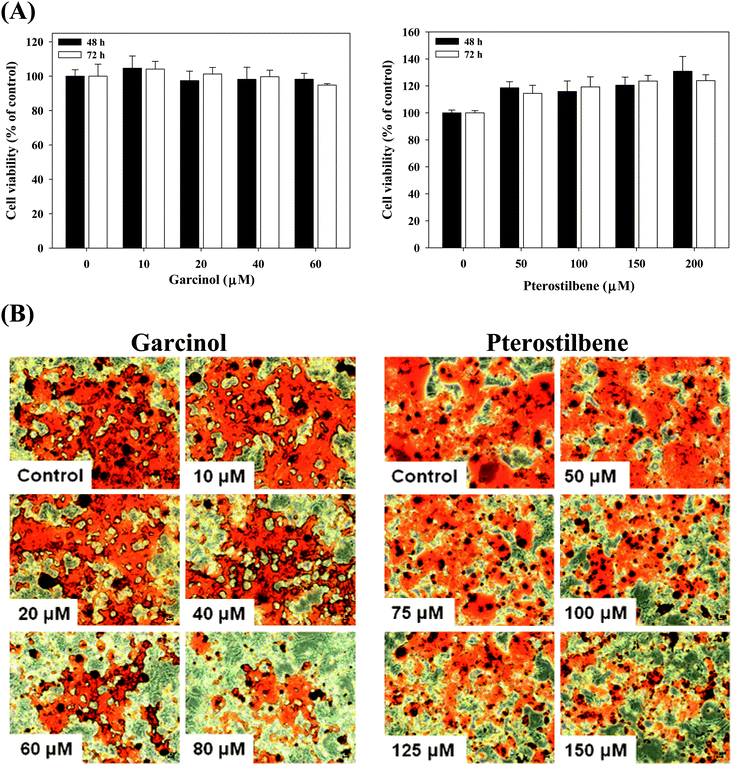 |
| Fig. 6 Effects of garcinol and pterostilbene on cell viability (A) and lipid accumulation (B) in 3T3-L1 adipocytes. 3T3-L1 adipocytes were harvested at 10 days after initiation of differentiation and then treated with garcinol or pterostilbene. Reported values are means ± SD (n = 3). | |
Effect of garcinol on adipogenic protein and gene expressions in 3T3-L1 adipocytes
Effect of garcinol on protein expressions of C/EBPα, PPARγ, FAS, adipose triglyceride lipase (ATGL), and adiponectin in 3T3-L1 adipocytes are shown in Fig. 7. Treatment of 3T3-L1 adipocytes with garcinol (0–80 μM, 24 h) significantly decreased the protein expressions of PPARγ and FAS. Additionally, the protein expressions of ATGL and adiponectin were remarkably increased when garcinol (80 μM, 24 h) was added to 3T3-L1 adipocytes. However, garcinol did not affect the C/EBPα protein expression of 3T3-L1 adipocytes. Effect of garcinol on mRNA expressions of adiponectin, leptin, and FAS in 3T3-L1 adipocytes is shown in Fig. 8. Treatment of 3T3-L1 adipocytes with garcinol (5 μM, 3 h) significantly increased the mRNA expression of adiponectin. The mRNA expressions of leptin and FAS were decreased after treatment of 3T3-L1 adipocytes with garcinol at 2.5 μM and 5 μM (3 h) and 5 μM (3 h), respectively.
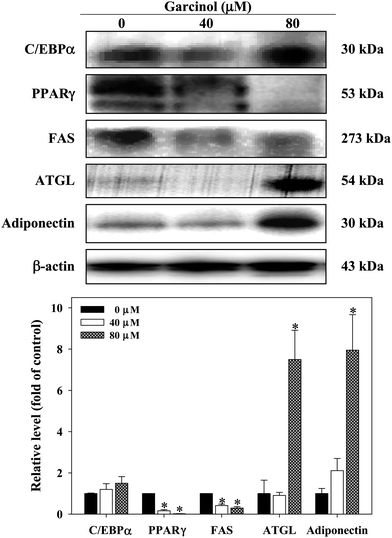 |
| Fig. 7 Effect of garcinol on protein expression of C/EBPα, PPARγ, FAS, ATGL, and adiponectin in 3T3-L1 adipocytes. 3T3-L1 adipocytes were harvested at 10 days after initiation of differentiation. The cells were treated with 0–80 μM of garcinol for 24 h. Reported values are means ± SD (n = 3). *Significantly different from control (p < 0.05). | |
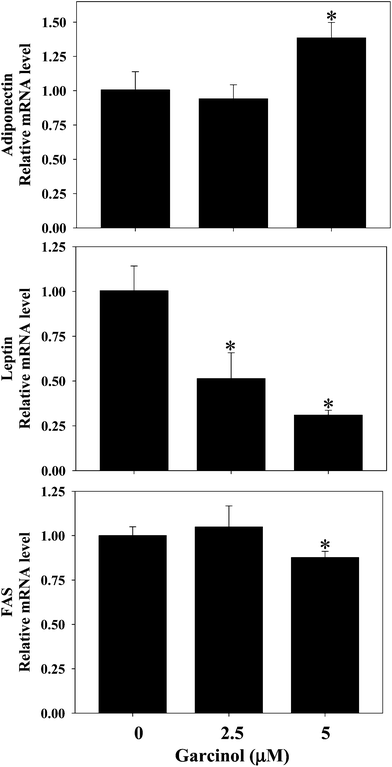 |
| Fig. 8 Effect of garcinol on mRNA expressions of adiponectin, leptin, and FAS in 3T3-L1 adipocytes. 3T3-L1 adipocytes were harvested at 10 days after initiation of differentiation. The cells were treated with 0–5 μM of garcinol for 3 h. Reported values are means ± SD (n = 3). *Significantly different from control (p < 0.05). | |
Discussion
Obesity has become a global health problem due to its association with various diseases, such as type 2 diabetes, cardiovascular disease and cancer.1 The prevalence of obesity and obesity-related disease has led to major research interests in the inhibition of adipogenesis.21 In the present study, we focused on the effects of garcinol and pterostilbene on cell proliferation and adipogenesis in 3T3-L1 preadipocytes, adipocytes undergoing differentiation, and mature adipocytes. Wang and Jones22 indicated that the decreased differentiation and proliferation of preadipocytes and decreased lipogenesis are mechanisms of proposed antiobesity. Our previous studies have shown that gallic acid and quercetin inhibit cell growth and induce apoptosis in 3T3-L1 preadipocytes.23–25 In the present study, the addition of garcinol and pterostilbene to the growth medium decreased the cell population growth of 3T3-L1 preadipocytes (Fig. 1). The flow cytometry assay indicated that the treatment of 3T3-L1 preadipocytes with garcinol and pterostilbene caused cell cycle arrested at G2/M phase (Fig. 2). Popovich et al.26 also indicated that the triterpenoid extract from bitter melon (Momordica charantia) caused cell cycle arrested at the G2/M phase in 3T3-L1 cells. Aguilar et al.27 have established a link between the cell cycle and the regulation of adipogenesis viaPPARγ interaction.
In 3T3-L1 adipocyte differentiation, our data indicated that garcinol and pterostilbene could inhibition of intracellular triacylglycerol and GPDH activity (Fig. 3). The cytosolic enzyme GPDH appears to have an important role in the conversion of glycerol into triacylglycerol.28Adipocyte is now known to produce and secrete PPARγ and C/EBPα, which have roles in the early stage of adipocyte differentiation.29 Our results indicated that garcinol and pterostilbene significantly down-regulated the protein expressions of C/EBPα and PPARγ in 3T3-L1 adipocyte differentiation (Fig. 4). Garcinol and pterostilbene also significantly up-regulated the gene expression of adiponectin as well as down-regulated the gene expressions of leptin, resistin and FAS in 3T3-L1 adipocyte differentiation (Fig. 5). A role for PPARγ in the regulation of numerous genes in the mature adipocyte, including insulin sensitivity and glucose homeostasis, has previously been reported .30 Suzuki et al.31 indicated that bilberry extracts (containing 25% anthocyanidins) significantly inhibit 3T3-L1 adipocyte differentiation through the insulin pathway.
In 3T3-L1 adipocytes, treatment of cells with garcinol and pterostilbene did not decrease adipocyte number (Fig. 6A), however, the exposure of 3T3-L1 adipocytes to garcinol and pterostilbene resulted in lower levels of lipid accumulation (Fig. 6B). Ambati et al.32 indicated that ajoene (natural garlic compound) had inhibitory effects on fat droplet formation and triacylglycerol accumulation in 3T3-L1 adipocytes. In the molecular mechanism, garcinol significantly down-regulated the protein expressions of PPARγ and FAS and also up-regulated the protein expressions of ATGL and adiponectin (Fig. 7). Garcinol also significantly up-regulated the gene expression of adiponectin as well as down-regulated the gene expressions of leptin and FAS (Fig. 8). Yamauchi et al.33 indicated that adiponectin is thought to play a crucial role in the suppression of obesity and type 2 diabetes. Our previous studies have shown that o-coumaric acid and rutin inhibit the expressions of PPARγ, C/EBPγ and leptin and then up-regulate expression of adiponectin at protein level in 3T3-L1 adipocytes.34 Lee et al.35 indicated shikonin (5,6-dihydroxyflavone-7-glucuronic acid, is an important component of Lithospermum erythrorhizon Sieb. et Zucc) inhibits fat accumulation in 3T3-L1 adipocytes through the down-regulation of SREBP1C expression and subsequently the expressions of PPARγ and C/EBPα.
Conclusions
In conclusion, these results indicate that garcinol and pterostilbene can inhibit cell proliferation and adipogenesis in 3T3-L1 preadipocytes, adipocytes undergoing differentiation and mature adipocytes. Garcinol and pterostilbene were found to inhibit the adipogenic protein/gene expressions in 3T3-L1 adipocytes undergoing differentiation and mature adipocytes. These results suggest that garcinol and pterostilbene have anti-adipogenic effect on preadipocytes, differentiating adipocytes and mature adipocytes.
Abbreviations
ATGL
| adipose triglyceride lipase; |
C/EBPα | CCAAT/enhancer binding protein; |
FAS |
fatty acid synthase; |
GPDH
|
glycerol-3-phosphate dehydrogenase; |
OROSM
| oil red O stained material. |
PPARγ
|
peroxisome proliferator-activated receptor. |
Acknowledgements
This research work was supported by the National Science Council, NSC 99-2622-B-005-004-CC2,Taiwan, Republic of China.
References
- P. G. Kopelman, Nature, 2000, 404, 635–643 Search PubMed.
- G. Medina-Gomez and A. Vidal-Puig, Nat. Med., 2005, 11, 602–603 Search PubMed.
- K. E. Wellen and G. S. Hotamisligil, J. Clin. Invest., 2005, 115, 1111–1119 Search PubMed.
- C. S. Mantzoros, Ann. Intern. Med., 1999, 130, 671–680 Search PubMed.
- A. H. Berg, T. P. Combs and P. E. Scherer, Trends Endocrinol. Metab., 2002, 13, 84–89 Search PubMed.
- K. Hotta, T. Funahashi, Y. Arita, M. Takahashi, M. Matsuda, Y. Okamoto, H. Iwahashi, H. Kuriyama, N. Ouchi, K. Maeda, M. Nishida, S. Kihara, N. Sakai, T. Nakajima, K. Hasegawa, M. Muraguchi, Y. Ohmoto, T. Nakamura, S. Yamashita, T. Hanafusa and Y. Matsuzawa, Arterioscler., Thromb., Vasc. Biol., 2000, 20, 1595–1599 Search PubMed.
- A. Xu, Y. Wang, H. Keshaw, L. Y. Xu, K. S. L. Lam and G. J. S. Cooper, J. Clin. Invest., 2003, 112, 91–100 Search PubMed.
- J. P. Whitehead, A. A. Richards, I. J. Hickman, G. A. Macdonald and J. B. Prins, Diabetes, Obes. Metab., 2006, 8, 264–280 Search PubMed.
- N. Valerio, M. Melania, C. Paolo, D. Vincenzo, D. Rita, P. Fiorella, C. Roberto, G. Donatella and M. Matilde, Eur. J. Endocrinol., 2006, 155, 735–743 Search PubMed.
- S. Padhye, A. Ahmad, N. Oswal and F. H. Sarkar, J. Hematol. Oncol., 2009, 2, 38 Search PubMed.
- N. Krishnamurthy, Y. S. Lewis and B. Ravindranath, Tetrahedron Lett., 1981, 22, 793–736 Search PubMed.
- C. H. Liao, S. Sang, Y. C. Liang, C. T. Ho and J. K. Lin, Mol. Carcinog., 2004, 41, 140–149 CrossRef CAS.
- A. Koeberle, H. Northoff and O. Werz, Biochem. Pharmacol., 2009, 77, 1513–1521 Search PubMed.
- A. M. Rimando, W. Kaltm, J. B. Magee, J. Dewey and J. R. Ballington, J. Agric. Food Chem., 2004, 52, 4713–4719 CrossRef CAS.
- M. H. Pan, Y. H. Chang, V. Badmaev, K. Nagabhushanam and C. T. Ho, J. Agric. Food Chem., 2007, 55, 7777–7785 CrossRef CAS.
- M. H. Pan, Y. H. Chang, M. L. Tsai, C. S. Lai, S. Y. Ho, V. Badmaev and C. T. Ho, J. Agric. Food Chem., 2008, 56, 7502–7509 Search PubMed.
- Y. S. Chiou, M. L. Tsai, Y. J. Wang, A. C. Cheng, W. M. Lai, V. Badmaev, C. T. Ho and M. H. Pan, J. Agric. Food Chem., 2010, 58, 8833–8841 Search PubMed.
- T. Mosmann, J. Immunol. Methods, 1983, 65, 55–63 CrossRef CAS.
- E. Takada, H. Toyota, J. Suzuki and J. Mizuguchi, J. Immunol., 2001, 166, 1641–1649 Search PubMed.
- J. L. Ramirez-Zacarias, F. Castro-Munozledo and W. Kuri-Harcuch, Histochemistry, 1992, 97, 493–497 Search PubMed.
- S. W. Ing and M. A. Belury, Nutr. Rev., 2011, 69, 123–131 Search PubMed.
- Y. W. Wang and P. J. Jones, Int. J. Obes., 2004, 28, 941–955 Search PubMed.
- C. L. Hsu, S. L. Huang and G. C. Yen, J. Agric. Food Chem., 2006, 54, 4191–4197 Search PubMed.
- C. L. Hsu and G. C. Yen, Mol. Nutr. Food Res., 2006, 50, 1072–1079 Search PubMed.
- C. L. Hsu, W. H. Lo and G. C. Yen, J. Agric. Food Chem., 2007, 55, 7359–7365 Search PubMed.
- D. G. Popovich, L. Li and W. Zhang, Food Chem. Toxicol., 2010, 48, 1619–1626 Search PubMed.
- V. Aguilar, J. S. Annicotte, X. Escote, J. Vendrell, D. Langin and L. Fajas, Endocrinology, 2010, 151, 5247–5254 Search PubMed.
- L. S. Wise and H. Green, J. Biol. Chem., 1979, 254, 273–275 Search PubMed.
- E. D. Rosen, C. J. Walkey, P. Puigserver and B. M. Spiegelman, Genes Dev., 2000, 14, 1293–1307 Search PubMed.
- J. Berger and D. E. Moller, The mechanisms of action of PPARs, Annu. Rev. Med., 2002, 53, 409–435 CrossRef CAS.
- R. Suzuki, M. Tanaka, M. Takanashi, A. Hussain, B. Yuan, H. Toyoda and M. Kuroda, Nutr. Metab., 2011, 8, 14 Search PubMed.
- S. Ambati, J. Y. Yang, S. Rayalam, H. J. Park, M. A. Della-Fera and C. A. Baile, Phytother. Res., 2009, 23, 513–518 Search PubMed.
- T. Yamauchi, J. Kamon, H. Waki, Y. Terauchi, N. Kubota, K. Hara, Y. Mori, T. Ide, K. Murakami, N. Tsuboyama-Kasaoka, O. Ezaki, Y. Akanuma, O. Gavrilova, C. Vinson, M. L. Reitman, H. Kagechika, K. Shudo, M. Yoda, Y. Nakano, K. Tobe, R. Nagai, S. Kimura, M. Tomita, P. Froguel and T. Kadowaki, Nat. Med., 2001, 7, 941–946 Search PubMed.
- C. L. Hsu and G. C. Yen, J. Agric. Food Chem., 2007, 55, 8404–8410 Search PubMed.
- H. Lee, R. Kang and Y. Yoon, Phytother. Res., 2010, 24, 344–351 Search PubMed.
|
This journal is © The Royal Society of Chemistry 2012 |