DOI:
10.1039/C1SC00175B
(Edge Article)
Chem. Sci., 2011,
2, 1835-1838
Enamine/carbene cascade catalysis in the diastereo- and enantioselective synthesis of functionalized cyclopentanones†
Received
22nd March 2011
, Accepted 23rd May 2011
First published on 16th June 2011
Abstract
Herein we report an enantioselective synthesis of complex cyclopentanones using aliphatic aldehydes and activated enones. With the combination of a chiral secondary amine and a chiral triazolium catalyst, high diastereoselectivity and excellent enantioselectivity can be achieved. We present evidence of a clear cooperative effect when these two catalysts are present simultaneously in the system.
Introduction
The incorporation of multiple catalytic cycles in a single procedure allows for complex compounds to be easily accessed, a concept that has been termed cascade catalysis.1–3 By eliminating the need for isolation and purification of intermediates, both time and resources are saved. This is especially important if these intermediates prove unstable upon isolation. While attractive, cascade catalysis provides unique challenges. With the possibility of multiple catalysts present simultaneously, the need for reaction selectivity is important. A solution to this problem is to use catalysts of orthogonal reactivity. Our group has previously reported that secondary amine and N-heterocyclic carbene (NHC) catalysts initiate cascade reactions of enals and β-dicarbonyls to form α-hydroxycyclopentanones (Fig. 1a).4 This reaction proceeds via iminium activation of the enal to induce a conjugate addition by the dicarbonyl followed by an intramolecular benzoin. Importantly the two catalysts work cooperatively, providing higher yields and enantioselectivities compared to a two-step process. Herein, we report the secondary amine/NHC catalyzed cascade reaction of aliphatic aldehydes and activated Michael acceptors to form complex cyclopentanones with a complementary substitution pattern.
Whereas our previous work employed iminium catalysis, we sought to explore the use of enamine catalysis in combination with NHC's in a cascade reaction. Ma has reported a highly diastereo- and enantioselective Michael addition of aliphatic aldehydes into activated enones with the use of a secondary amine catalyst (3).5 We speculated that the aldehyde intermediate formed could undergo an intramolecular benzoin reaction when exposed to an N-heterocyclic carbene catalyst (Fig. 1b). This would provide a cyclopentanone product in what may be considered a formal [3 + 2] cycloaddition. This approach provides complimentary and inaccessible substitution patterns from our previous work.
Results and discussion
Reaction development
The reaction conditions developed by the Ma group were repeated using the Jørgensen–Hayashi catalyst 37 in methanol at room temperature. After observing the consumption of starting material, achiral triazolium salt 58 and sodium acetate were added to catalyze the benzoin cyclization. These conditions provide no desired product (Table 1, entry 1). When methanol is replaced with chloroform, the desired product is formed in 29% yield and 96% ee but with a 3
:
1
:
<1
:
<1 dr (Table 1, entry 2).9 Employing a one-step protocol with all reagents present from the outset results in an increase in yield to 35% (entry 3). Increasing the temperature from 23 °C to 60 °C improves the yield to 89% while maintaining high enantioselectivity (96% ee) and a 5
:
1
:
<1
:
<1 diastereomeric ratio. The diastereoselectivity is further improved to 290
:
15
:
6
:
1 when the chiral aminoindanol based triazolium 610,11 is used (entry 5). The use of the antipode of this catalyst, 6′, results in lower diastereoselectivity (4
:
1
:
<1
:
<1). This is likely a result of a match/mismatch relationship.
|
Solvent
|
Triazolium salt |
T (°C) |
Yield (%) |
ee (%)b |
drb |
See supporting information for general procedure.†
Enantioselectivity and diastereoselectivity were determined by GC.
20 mol % Triazolium salt was added after full consumption of 2a.
20 mol % Triazolium salt was added at the beginning of the reaction.
|
1c |
MeOH
|
|
23 |
0 |
— |
— |
2c |
CHCl3 |
5
|
23 |
29 |
96 |
3 : 1 : <1 : <1 |
3d |
CHCl3 |
5
|
23 |
35 |
95 |
2 : 1 : <1 : <1 |
4d |
CHCl3 |
5
|
60 |
89 |
96 |
5 : 1 : <1 : <1 |
5d |
CHCl3 |
|
60 |
87 |
95 |
19 : 1 : <1 : <1 |
6d |
CHCl3 |
|
60 |
59 |
93 |
4 : 1 : <1 : <1 |
Synthetic scope
With suitable conditions established, a variety of substrates were screened to explore the scope of this new cascade. Aliphatic aldehydes provide the desired products in good yield and high enantio- and diastereoselectivity (Chart 1, 4b–c). Isovaleraldehyde competitively forms the Stetter product12 in a 1
:
1 ratio with cyclopentanone 4d under standard conditions. This side product can be avoided when the introduction of the triazolium is delayed until after complete formation of the intermediate. Larger aldehydes are also viable but routinely require prolonged reaction times (Chart 1, 4f–g).
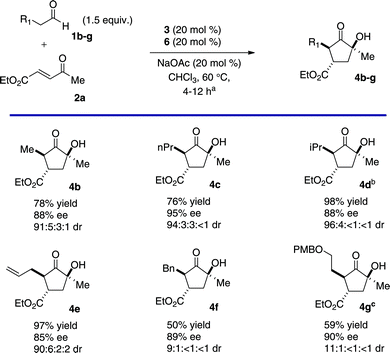 |
| Chart 1
Aldehyde Scopea (aSee Supporting Information†. bCatalyst 6 was added after consumption of starting material. cDiastereomeric ratio determined by 1H NMR.) | |
Variation in the enone component was then explored (Chart 2). Esters and tertiary amides are viable under these conditions (Chart 2, 4h–k).13 Substitution at the ketone position is also tolerated. N-Alkyl ketones provide products in excellent yields while maintaining high stereoselectivity. The isopropyl ketone failed to cyclize under standard conditions. By substituting the bulky triazolium 6 with the smaller achiral catalyst 5, the intramolecular benzoin was accomplished albeit with a curiously low enantioselectivity (4o). Phenyl ketone can also be employed, with diminished diastereoselectivity (4n). Diketones may also be used to form products (4p–q) with good selectivity despite diminished yield.
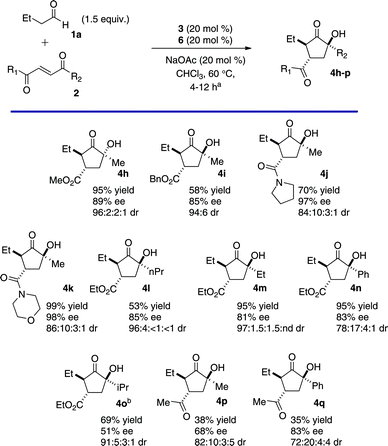 |
| Chart 2
Keto-ester Scopea (aSee Supporting Information†. bCatalyst 5 was used in place of 6.) | |
With high complexity already built into the cyclopentanone products, functionalization should allow rapid access to even more elaborate products. The addition of sodium triacetoxyborohydride at the end of the reaction permits a diastereoselective reduction of the ketone to 1,2-diol 7. This action effectively permits the formation of a fourth stereocenter in one pot (eqn (1)).
| 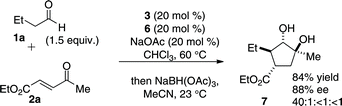 | (1) |
Mechanistic insights
We then explored if there is an advantage between this one-step protocol versus a two-step reaction. Aldehyde 8 was prepared by exposing butyraldehyde and enone 2a to catalyst 3, catalytic acetic acid, and chloroform at room temperature (Scheme 1). After purification, the aldehyde intermediate was isolated in moderate yield with a 2
:
1 diastereomeric ratio.
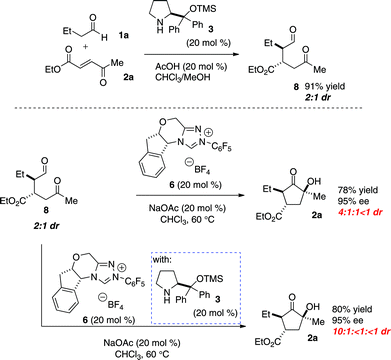 |
| Scheme 1 Two-Pot Reactions | |
Exposure of this intermediate to benzoin conditions provides the cyclized product in comparable yield and enantioselectivity, but with a low diastereomeric ratio (4
:
1
:
1
:
<1). This diastereoselectivity can be improved when the benzoin cyclization is performed with the addition of chiral amine catalyst 3 (10
:
1
:
<1
:
<1 dr).
In our previous work, it was discovered that the amine catalyst is responsible for a retro-Michael reaction, which eroded enantioselectivity in the two-pot reaction. Crossover experiments indicate that this pathway is non-operative in this system.14 Instead, we propose that the secondary amine catalyst is capable of epimerizing the α-position of the intermediate aldehyde to form an equilibrium between 8 and 8′ (Fig. 2). The chiral triazolium 6 prefers cyclization with only one of these diastereomers, and this adduct continues on to the final product. The amine catalyst thus aids in converting the less reactive diastereomer into its epimer.15 This hypothesis explains how the amine catalyst can convert aldehyde 8 with low diastereoselectivity to a diastereomerically enriched product.
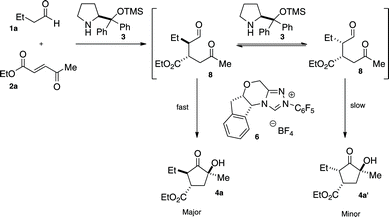 |
| Fig. 2 Proposed Mechanism | |
To support this mechanism, the benzoin cyclization was monitored over time by NMR spectroscopy. When intermediate aldehyde 8 is exposed to catalyst 6, we see complete consumption of one diastereomer in preference over the other (Fig. 3). When amine catalyst 3 is included in this reaction, consumption of both diastereomers occurs over the course of the reaction, consistent with the hypothesis that amine catalyst 3 serves to interconvert the two diastereomers of 8.16,17
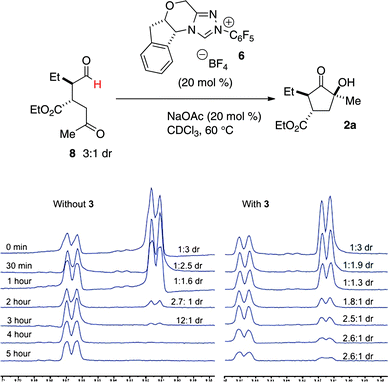 |
| Fig. 3
NMR experiments | |
Conclusion
In summary, a one-pot stereoeselective Michael–Benzoin cascade reaction has been developed for the synthesis of complex cyclopentanones. The presence of both the secondary amine and triazolium catalysts is essential for excellent results, indicating a cooperative relationship between the catalysts. This provides a unique and useful method to form complicated cyclopentanes from simple starting materials.
Acknowledgements
We thank NIGMS for generous support of this research (GM72586). K. E. O. thanks NIH (GM80442-S1 and GM096749) for funding. T.R. thanks Amgen and Roche for unrestricted support. We thank Donald Gauthier and Greg Hughes (Merck) for a generous gift of aminoindanol and Kevin M. Oberg and Derek M. Dalton (CSU) for solving the crystal structure of 9.
Notes and references
- For recent reviews:
(a) C. Grondal, M. Jeanty and D. Enders, Nat. Chem., 2010, 2, 167 CrossRef CAS;
(b) J. Zhou, Chem.–Asian J., 2010, 5, 422 CrossRef CAS;
(c) Y. Xinhong and W. Wang, Org. Biomol. Chem., 2008, 6, 2037 RSC;
(d) D. Enders, C. Grondal and M. R. M. Hüttl, Angew. Chem., Int. Ed., 2007, 46, 1570 CrossRef CAS;
(e) A. M. Walji and D. W. C. MacMillan, Synlett, 2007, 1477 CAS;
(f) J.-C. Wasilke, S. J. Obrey, R. T. Baker and G. C. Bazan, Chem. Rev., 2005, 105, 1001 CrossRef CAS.
- For some recent examples of multicatalytic systems:
(a) A. Quintard, A. Alexakis and C. Mazet, Angew. Chem. Int. Ed, 2011, 50, 2354 CAS;
(b) B. M. Trost and X. Luan, J. Am. Chem. Soc., 2011, 133, 1706 Search PubMed;
(c) D. E. A. Raup, B. Cardinal-David, D. Holte and K. A. Scheidt, Nat. Chem., 2010, 2, 766 CrossRef CAS;
(d) C. Yu, Y. Zhang, S. Zhang, J. He and W. Wang, Tetrahedron Lett., 2010, 51, 1742 Search PubMed;
(e) B.-C. Hong, N. S. Dange, C.-S. Hsu and J.-H. Liao, Org. Lett., 2010, 12, 4812 CrossRef CAS;
(f) Y. Huang, A. M. Walji, C. H. Larsen and D. W. C. MacMillan, J. Am. Chem. Soc., 2009, 127, 15051 Search PubMed;
(g) B. Simmons, A. M. Walji and D. W. C. MacMillan, Angew. Chem., Int. Ed., 2009, 48, 4349 CrossRef CAS;
(h) H. Jiang, P. Elsner, K. L. Jensen, A. Falcicchio, V. Marcos and K. A. Jørgensen, Angew. Chem., Int. Ed., 2009, 48, 6844 CrossRef CAS;
(i) S. Belot, K. A. Vogt, C. Besnard, N. Krause and A. Alexakis, Angew. Chem., Int. Ed., 2009, 48, 8923 CrossRef CAS;
(j) Y. Wang, R.-G. Han, Y.-L. Zhou, S. Yang, P.-F. Xu and D. J. Dixon, Angew. Chem., Int. Ed., 2009, 48, 9834 CrossRef CAS;
(k) T. A. Cernak and T. H. Lambert, J. Am. Chem. Soc., 2009, 131, 3124 CrossRef CAS;
(l) B. D. Kelly, J. M. Allen, R. E. Tundel and T. H. Lambert, Org. Lett., 2009, 11, 1381 CrossRef CAS;
(m) D. A. Nicewicz and D. W. C. MacMillan, Science, 2008, 322, 77 CrossRef CAS;
(n) Y. Chi, S. T. Scroggins and J. M. J. Fréchet, J. Am. Chem. Soc., 2008, 130, 6322 CrossRef CAS;
(o) L.-Q. Lu, Y.-J. Cao, X.-P. Liu, J. An, C.-J. Yao, Z.-H. Ming and W.-J. Xiao, J. Am. Chem. Soc., 2008, 130, 6946 CrossRef CAS;
(p) J. Zhou and B. List, J. Am. Chem. Soc., 2007, 129, 7498 CrossRef CAS;
(q) W. Wang, H. Li, J. Wang and L. Zu, J. Am. Chem. Soc., 2006, 128, 10354 CrossRef CAS.
- For select recent examples of cascade reactions with a single catalyst:
(a) C. Liu, X. Zhang, R. Wang and W. Wang, Org. Lett., 2010, 12, 4948 CrossRef CAS;
(b) N. T. Jui, E. C. Y. Lee and D. W. C. MacMillan, J. Am. Chem. Soc., 2010, 132, 10015 CrossRef CAS;
(c) P. G. McGarraugh and S. E. Brenner, Org. Lett., 2009, 11, 5654 Search PubMed;
(d) L. Zu, S. Zhang, H. Xie and W. Wang, Org. Lett., 2009, 11, 1627 CrossRef CAS;
(e) G. Bencivenni, L.-Y. Wu, A. Mazzanti, B. Giannichi, F. Pesciaoli, M.-P. Song, G. Bartoli and P. Melciorre, Angew. Chem., Int. Ed., 2009, 48, 7200 CrossRef CAS;
(f) J. Wang, H. Li, H. Xie, L. Zu, X. Shen and W. Wang, Angew. Chem., Int. Ed., 2007, 46, 9050 CrossRef CAS;
(g) H. Xie, L. Zu, H. Li, J. Wang and W. Wang, J. Am. Chem. Soc., 2007, 129, 10886 CrossRef CAS;
(h) J. Zhou and B. List, J. Am. Chem. Soc., 2007, 129, 7498 CrossRef CAS;
(i) D. Enders, M. R. M. Hüttl, C. Grondal and G. Raabe, Nature, 2006, 441, 861 CrossRef CAS;
(j) Y. Huang, A. M. Walji, C. H. Larsen and D. W. C. MacMillan, J. Am. Chem. Soc., 2005, 127, 15051 CrossRef CAS.
-
(a) S. P. Lathrop and T. Rovis, J. Am. Chem. Soc., 2009, 131, 13628 CrossRef CAS. For other contributions to cascade catalysis from our laboratories, see:
(b) H. U. Vora and T. Rovis, J. Am. Chem. Soc., 2007, 129, 13796 CrossRef CAS;
(c) C. M. Filloux, S. P. Lathrop and T. Rovis, Proc. Natl. Acad. Sci. U. S. A., 2010, 107, 20666 CrossRef CAS.
- J. Wang, A. Ma and D. Ma, Org. Lett., 2008, 10, 5425 CrossRef CAS.
- The “IM” and “EN” convention to denote iminium and enamine catalysis was first introduced by MacMillan and coworkers and subsequently adopted by others. See:
(a) Y. Huang, A. M. Walji, C. H. Larsen and D. W. C. MacMillan, J. Am. Chem. Soc., 2005, 127, 15051 CrossRef CAS;
(b) also see ref. 1a..
-
(a) M. Marigo, T. C. Wabnitz, D. Fielenbach and K. A. Jørgensen, Angew. Chem., Int. Ed., 2005, 44, 794 CrossRef CAS;
(b) Y. Hayashi, H. Gotoh, T. Hayashi and M. Shoji, Angew. Chem., Int. Ed., 2005, 44, 4212 CrossRef CAS.
- M. S. Kerr, J. Read de Alaniz and T. Rovis, J. Org. Chem., 2005, 70, 5725 CrossRef CAS.
- Absolute configuration determined by Single Crystal X-ray diffraction of the chiral salt (see ESI†):
.
-
(a) H. U. Vora, S. P. Lathrop, N. T. Reynolds, M. S. Kerr, J. Read de Alaniz and T. Rovis, Org. Synth., 2010, 87, 350 Search PubMed;
(b) M. S. Kerr, J. Read de Alaniz and T. Rovis, J. Am. Chem. Soc., 2002, 124, 10298 CrossRef CAS.
-
(a) Y. Hachisu, J. W. Bode and K. Suzuki, J. Am. Chem. Soc., 2003, 125, 8432 CrossRef CAS;
(b) H. Takikawa, Y. Hachisu, J. W. Bode and K. Suzuki, Angew. Chem., Int. Ed., 2006, 45, 3492 CrossRef CAS;
(c) D. Enders, O. Niemeier and T. Balensiefer, Angew. Chem., Int. Ed., 2006, 45, 1463 CrossRef CAS.
- Stetter Product formed:
.
-
Secondary amides and thioesters do not provide desired products.
- See Supporting Information for crossover experiment results†.
- The second step of this reaction could then be considered analogous to a dynamic kinetic resolution, which has been invoked in several other organocatalyzed transformations:
(a) A. Lee, A. Michrowska, S. Sulzer-Mosse and B. List, Angew. Chem., Int. Ed., 2011, 50, 1707 Search PubMed;
(b) S. Hoffman, M. Nicoletti and B. List, J. Am. Chem. Soc., 2006, 128, 13074 CrossRef CAS;
(c) D. E. Ward, V. Jheengut and O. T. Akinnusi, Org. Lett., 2005, 7, 1181 CrossRef CAS.
- See Supporting Information for spectra from NMR experiments†.
- In addition, when intermediate 8 is exposed to catalyst 3 in deuterated methanol at elevated temperature, complete deuteration is observed at the α-position
.
Footnote |
† Electronic supplementary information (ESI) available: Experimental procedures, spectra, and crystal structures are provided. See DOI: 10.1039/c1sc00175b |
|
This journal is © The Royal Society of Chemistry 2011 |
Click here to see how this site uses Cookies. View our privacy policy here.