DOI:
10.1039/C1SC00124H
(Edge Article)
Chem. Sci., 2011,
2, 1842-1849
Intramolecular frustrated N/B lewis pairs by enamine hydroboration†
Received
2nd March 2011
, Accepted 27th May 2011
First published on 16th June 2011
Abstract
A series of enamines undergoes anti-Markovnikov hydroboration with Piers' borane HB(C6F5)2 to yield C2-bridged frustrated N/B Lewis pairs, featuring weak N⋯B interaction. Some examples show typical frustrated Lewis pair reactions with dihydrogen or with 1-alkynes. One such N/B FLP serves as an active catalyst for the hydrogenation of enamines. Many of the new compounds were characterized by X-ray diffraction.
Introduction
Frustrated Lewis Pairs (FLP) are systems of co-existing active Lewis acids and bases. Mostly, their mutual annihilation is prevented by the presence of sufficient steric hindrance caused by bulky substituents of both the Lewis acid and the Lewis base component of the pair.1 Meanwhile a number of combinations of the strong boron Lewis acid B(C6F5)3 with various phosphorus,2 nitrogen3,4 or even carbon centered5 bulky Lewis bases have been described.6 From the onset of FLP chemistry, intramolecular non-quenched Lewis acid/base combinations have received special attention.7
We had described the intramolecular phosphine/borane system 1 which so far is one of the most active FLP systems for cooperative heterolytic dihydrogen cleavage.8,9 The hydrogen activation system 1/2 (see Chart 1) is an active metal-free catalyst for the hydrogenation of enamines or imines (other FLP systems even catalytically hydrogenate silyl enol ethers10). The frustrated Lewis pair 1 was shown to have a weak Lewis acid–Lewis base interaction.11,12
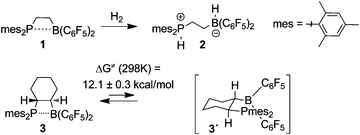 |
| Chart 1 Examples of frustrated P/B Lewis pairs. | |
We had shown that the derivative 3 features a four-membered heterocyclic ring structure in the crystal that contains a long (and probably weak) P–B bond. From the dynamic 19F-NMR spectra of 3 we determined a Gibbs activation energy of ΔG≠ (298 K) = 12.1 ± 0.3 kcal mol−1 for P–B bond dissociation in the intramolecular FLP 3 (see Chart 1).13
Since amines represent an important class of Lewis bases, we have now prepared a series of related C2-bridged intramolecular N/B frustrated Lewis pairs and compared their interesting features with those of the established intramolecular P/B Lewis pairs.
Results and discussions
Synthesis and structural characterization
We prepared the new C2-bridged amine/borane frustrated Lewis pairs (5) by means of enamine routes. As a typical example 1-diethylaminostyrene (4a)14 was reacted with “Piers' borane” [HB(C6F5)2]15 by anti-Markovnikov orientated hydroboration to yield the N/B Lewis pair 5a (70%). Compound 5a was characterized spectroscopically, by C,H,N elemental analysis and by X-ray diffraction (see Fig. 1 and Table 1).
Table 1 Selected structural data of the frustrated N/B Lewis pairs 5a, c and d (bond lengths in Å, angles in °)
Compound: |
5a
|
5c
|
5d
|
N–B |
1.719(3) |
1.723(2) |
1.824(6) |
N–C1 |
1.564(3) |
1.563(2) |
1.508(6) |
C1–C2 |
1.516(3) |
1.567(3) |
1.514(5) |
C2–B |
1.627(3) |
1.682(3) |
1.634(7) |
|
C1–N–B |
84.8(1) |
88.1(1) |
82.3(3) |
C2–B–N |
84.3(2) |
85.5(1) |
81.2(3) |
N–C1–C2 |
93.7(2) |
95.3(1) |
96.5(3) |
C1–C2–B |
89.6(2) |
89.4(1) |
88.8(3) |
C31–B–C41 |
109.3(2) |
102.9(1) |
109.6(3) |
N–C1–C2–B |
−22.0(2) |
10.3(1) |
−28.2(3) |
C1–C2–B–N |
20.0(1) |
−9.3(1) |
23.1(3) |
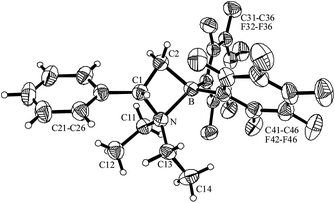 |
| Fig. 1 A view of the molecular structure of compound 5a. | |
Compound 5a features a central NC2B four-membered heterocyclic ring system. The N–B linkage is rather long at 1.719(3) Å.16 The B–C2 bond (1.627(3) Å) is significantly shorter than the adjacent B–C31 (1.640(3) Å and B–C41 (1.641(3) Å) linkages (angle C31–B–C41: 109.3(2)°). The central four-membered ring is slightly puckered (angle between the C2–C1–N and C2–B–N planes: 29.5°).
In solution, compound 5a shows a 11B NMR signal in the borate region at δ 2.1. It features the 1H/13C NMR signals of diastereotopic ethyl groups at nitrogen [13C: δ 50.2, 9.0/40.7, 8.3]. The CH2 group of each N–C2H5 unit also shows diastereotopic splitting in the 1H NMR spectrum due to the chirality center at C1 of the bridge (for details see the Supporting Information†). The [N]–CHPh–CH2–[B] bridge shows a typical pattern of 1H NMR resonances at δ 2.20/1.80 (2JHH = 13.5 Hz) and 4.55 (3JHH = 13.5 and 7.1 Hz) [13C: δ 17.9 (br, C2), 75.0 (C1)]. The pair of C6F5 substituents at boron are also diastereotopic (470 MHz, 298 K, in benzene-d6). They show Δδ19F(m,p) differences (see Scheme 1) in a range between typical values of tri- and tetracoordinated boron.17
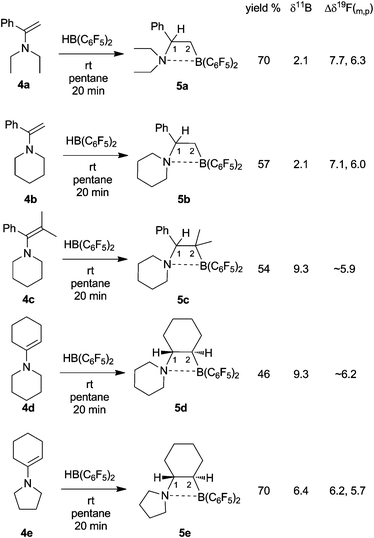 |
| Scheme 1 Synthesis and selected physical data of the frustrated N/B Lewis pairs 5a-e | |
The analogous HB(C6F5)2 hydroboration reaction of the piperidino substituted styrene derivative 4b gave the N/B Lewis pair 5b. It shows similar spectroscopic features as 5a. Due to the chirality center (C1 of the bridging –CHPh–CH2–unit) the hydrogens of the CH2 pairs of the piperidino moiety have become diastereotopic, giving rise to a total of nine separate signals in the 1H NMR and five resonances in the 13C NMR spectrum.
Hydroboration of the tetrasubstituted enamine carbon–carbon double bond of 4c gave compound 5c which was characterized by X-ray diffraction. The structural features of 5c are similar to those of 5a (see Table 1). The N–B bond length in the central four-membered heterocyclic subunit of 5c was found at 1.723(2) Å (for a view of the structure and further details see the Supporting Information). In solution compound 5c shows the NMR signals of a pair of diastereotopic methyl substituents at C2 of the bridge [1H: δ 1.33, 0.95 (each 3H), 13C: δ 33.2, 27.3].
Hydroboration of piperidino-cyclohexene (4d) with HB(C6F5)2 gave the FLP system 5d. The X-ray crystal structure analysis revealed the 1,2-trans-attachment of both the –N(C5H10) and the –B(C6F5)2 substituents at the chair-shaped cyclohexane backbone. The chiral (racemic) N/B frustrated Lewis pair 5d also shows a direct N-B interaction, although the specific attachment at the cyclohexylene carbocycle appears to introduce some strain: the N-B bond in 5d (1.824(6) Å) is by about 0.1 Å longer than the respective bonds in the related non-annulated compounds 5a and 5c (see Table 1 and Fig. 2).
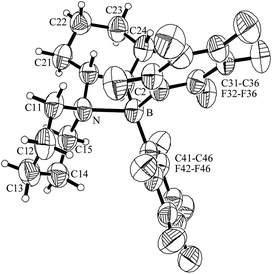 |
| Fig. 2 A view of the molecular structure of the FLP system 5d. | |
Eventually, we reacted the closely related enamine starting material pyrrolidino-cyclohexene (4e) with HB(C6F5)2 to obtain 5e, which was isolated in 70% yield.
Dynamic features
We were able to determine the Gibbs activation energies ΔG≠ (T)diss of the N–B dissociation process for the chiral examples 5b and 5d of this new class of compounds from their dynamic NMR spectra.
The chiral four-membered heterocyclic Lewis pair 5b shows the 19F NMR spectra of a pair of diastereotopic C6F5 groups at boron. Ring opening of 5b by N–B bond dissociation would give the open isomer 5b′ (see Scheme 2) which contains a non-stereogenic trigonal-planar boron center.13 Consequently, its C6F5 groups are homotopic in the NMR experiment. Therefore, rapid equilibration between the closed global minimum isomer 5b with the not directly observable open local minimum structure 5b′ of higher energy content on the NMR time scale would reveal itself by temperature dependent coalescence of the signals of respective pairs of diastereotopic groups.
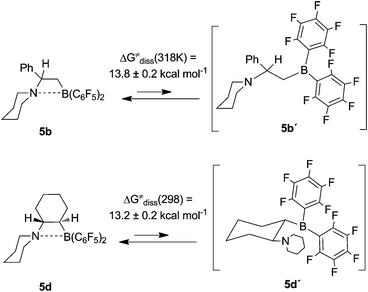 |
| Scheme 2 Dynamic behaviour of the N/B FLPs 5. | |
This is actually observed: At low temperature the 19F NMR spectrum of compound 5b (564 MHz, 283 K) shows well separated sets of ortho-, para- and meta-signals of the pair of C6F5 substituents at boron. Increasing the monitoring temperature rapidly results in a pairwise coalescence of the ortho-, para- and meta- 19F NMR signals to eventually result in the observation of a single averaged set (see Fig. 3 and the Supporting Information†). From these dynamic 19F NMR spectra we estimated the Gibbs activation energy of the N-B dissociation process in compound 5b as ΔG≠(318 K) = 13.8 ± 0.2 kcal mol−1 (based on the p-19F resonance; for details see the Supporting Information†).
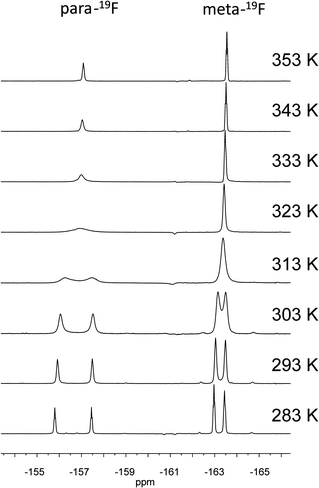 |
| Fig. 3 Dynamic 19F NMR spectra of compound 5b, only the p- and m-C6F5 resonances are depicted. | |
The analogous investigation was carried out with the cyclohexylene-bridged N/B Lewis pair 5d. From the coalescence of the respective pairs of 19F NMR resonances we estimated the Gibbs activation barrier of the N-B dissociation process at ΔG≠ (298 K) = 13.2 ± 0.2 kcal mol−1 (based on the p-19F resonance; for details see the Supporting Information†). We note that this value is slightly lower for 5d than for 5b which probably reflects the increased strain of the four-membered heterocycle in the former due to the ring annulation. This coincides with the observed rather long N–B distance in 5d (see above).
Chemical properties and reactions
The reaction of the FLPs 5a and 5b with pyridine led to the formation of their respective Lewis acid/Lewis base adducts 6a and 6b, respectively (Scheme 3).18 In the latter case, adduct formation is evidenced by a characteristic changing of the 1H NMR ABX pattern of the [N]–CHPh–CH2–[B] signals of the C2-bridge, the 19F NMR chemical shifts and the chemical shift of the 11B NMR resonance [5b: δ 2.1; 6b: δ −0.6] (for further details see the Experimental Section and the Supporting Information†).
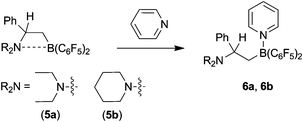 |
| Scheme 3 Reaction of the N/B FLPs 5 with pyridine. | |
Compound 5d undergoes a typical FLP reaction with terminal alkynes.19 Thus, treatment of in situ generated 5d with 1-pentyne takes place rapidly at room temperature in pentane solution to yield the zwitterionic product 7b as a red solid (59% yield, see Scheme 4). The product 7b shows a 1H NMR N–H resonance (of 1H rel. intensity) at δ 9.42 and a 11B NMR signal at δ −17.8. It features the 19F NMR signals of a pair of diastereotopic C6F5 substituents at boron.
The compound 7b was characterized by X-ray diffraction (see Fig. 4 and Table 2). It shows a non-constrained B/N-substituted cyclohexane chair. The [–B(C6F5)2–C
C–C3H7] borate substituent and the [–NH(C5H10)] ammonium group are trans-1,2-attached at the ring. Both the borate B and the ammonium N centers are pseudo-tetrahedrally coordinated. The nitrogen atom has a proton attached to it and the remaining acetylide anion in the zwitterionic product 7b is found bonded to boron (see Fig. 4).
Table 2 Selected structural parameters of the zwitterionic FLP/acetylene reaction products 7 and 8 (bond lengths in Å, angles in °)
Compound: |
7a
|
7b
|
7c
|
8
|
R |
Ph |
n-C3H7 |
n(CH2)3C CH |
Ph |
N⋯B |
3.162(3) |
3.158(2) |
3.160(4) |
3.233(4) |
N–C1 |
1.524(3) |
1.528(2) |
1.531(4) |
1.538(3) |
C1–C2 |
1.539(3) |
1.538(2) |
1.531(4) |
1.536(4) |
C2–B |
1.658(3) |
1.666(2) |
1.659(5) |
1.646(4) |
B–C3 |
1.601(3) |
1.602(2) |
1.608(5) |
1.594(5) |
C3–C4 |
1.205(3) |
1.199(2) |
1.201(4) |
1.216(4) |
|
C2–B–C3 |
114.7(2) |
114.3(1) |
112.9(3) |
109.9(2) |
C1–C2–B |
117.0(2) |
116.4(1) |
116.7(2) |
114.8(2) |
N–C1–C2 |
111.4(2) |
111.3(1) |
112.5(2) |
108.9(2) |
N–C1–C2–B |
−56.7(2) |
−57.2(2) |
−54.9(4) |
−72.4(3) |
C1–C2–B–C3 |
57.1(3) |
54.1(2) |
60.7(4) |
59.1(3) |
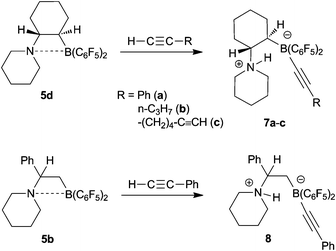 |
| Scheme 4 Reactions of the N/B FLPs 5 with 1-alkynes | |
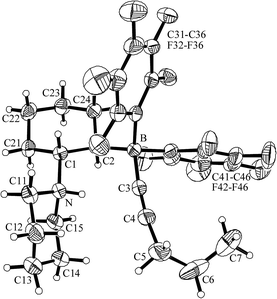 |
| Fig. 4 Molecular structure of compound 7b. | |
The reaction of 5d with phenylacetylene takes an analogous course: deprotonation of the C–H acidic C(sp)–H acetylene terminus of the alkyne by the amine Lewis base component takes place and the remaining phenylacetylide moiety is then accommodated by the adjacent boron Lewis acid functionality19,20 to give the zwitterionic cyclohexylene-bridged ammonium–alkynyl borate product 7a (isolated in 64% yield).
Compound 7a was characterized spectroscopically, by C,H,N elemental analysis and by X-ray crystal structure analysis (depicted in the Supporting Information,† see also Table 2). Similarly, the reaction of 5d with 1,7-octadiyne gave the mono-C-H activation product 7c, that was also characterized by X-ray diffraction. Its essential structure features are similar to those of 7a and 7b (for details see Table 2 and the Supporting Information†).21
We have also reacted the frustrated Lewis pair 5b with phenylacetylene and obtained the zwitterionic product 8 (58% isolated). It shows a N–H ammonium 1H NMR resonance at δ 8.92 and a 11B NMR signal at δ −19.2, typical of a borate anion system. The X-ray crystal structure analysis (Fig. 5) shows a slightly opened gauche arrangement of the C1–N (1.538(3) Å) and C2–B (1.646(4) Å) vectors at the connecting C1–C2 unit (θ N–C1–C2–B: −72.4(3)°). The ammonium N–H is directed towards the linear B-acetylide unit (angles B–C3–C4: 175.6(3)°, C3–C4–C5: 176.9(4)°).
The frustrated P/B Lewis pairs 1 and 3 very efficiently split dihydrogen heterolytically1,11,12,13 under mild conditions (see above). In principle, the new frustrated N/B Lewis pairs react similar, although there are a number of differences in detail. We exposed the N/B Lewis pairs 5a–e to 2.5 bars of dihydrogen at room temperature, usually in pentane solution. All the positive reactions were subsequently confirmed by the analogous treatment of the systems 5 with deuterium (D2) to actually involve heterolytic splitting of the H–H, respectively D–D bond.
Under these typical “ambient” conditions the systems 5a and 5c did not react with hydrogen. In contrast, the apparently less sterically hindered frustrated N/B Lewis pair 5b reacted rapidly with H2 at these standard conditions to yield the zwitterionic ammonium/hydridoborate product 9b. It was isolated as a colourless solid in 58% yield. The reactivity of the 5b system towards H2 is quite similar to that of related intramolecular frustrated P/B Lewis pairs. The product 9b (see Scheme 5) was characterized spectroscopically [11B NMR: δ −22.8 (d, 1JBH ∼ 80 Hz); 1H NMR: δ 7.46 (br, 1H, N–H), 3.08 (br 1
:
1
:
1
:
1 q, 1H, 1JBH ∼ 80 Hz, B–H) and by X-ray diffraction (see Fig. 6 and Table 3).
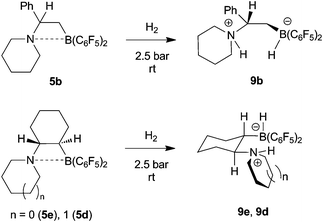 |
| Scheme 5 Reaction of some N/B FLPs with dihydrogen | |
Table 3 Selected structural data of the zwitterionic H2 activation products 9 (bond lengths in Å, angles in °)
Compound: |
9b
|
9d
|
9e
|
N–C1 |
1.539(3) |
1.523(2) |
1.520(3) |
C1–C2 |
1.524(3) |
1.537(2) |
1.536(3) |
C2–B |
1.638(3) |
1.652(2) |
1.652(3) |
N⋯B |
3.039(3) |
3.011(2) |
2.992(3) |
N–H⋯H–B |
1.90 |
1.77 |
1.80 |
|
C1–N–C11 |
115.0(2) |
114.8(1) |
116.6(2) |
C1–N–C15/(14) |
113.4(2) |
114.6(1) |
116.6(2) |
C2–B–C31 |
114.0(2) |
110.9(1) |
111.0(2) |
C2–B–C41 |
111.6(2) |
115.3(1) |
117.5(2) |
N–C1–C2 |
108.0(2) |
109.8(1) |
109.0(2) |
C1–C2–B |
113.1(2) |
114.7(1) |
112.8(2) |
N–C1–C2–B |
−60.7(2) |
50.0(2) |
54.0(2) |
The X-ray crystal structure analysis of compound 9b shows the presence of an ammonium unit (C11–N–C15: 110.6(2)°) and a hydridoborate moiety (C31–B–C41 107.5(2)°) attached at the (–CHPh–CH2)-bridge. This central unit attains a gauche conformation of the C1–N and C2–B vectors (θ N–C1–C2–B: −60.7(2)°). The N–H and B–H vectors are oriented towards each other.
The reaction of the cyclohexylene-bridged N/B Lewis pair 5d with dihydrogen proceeded analogously to yield the zwitterion 9d [50% isolated; 11B NMR: δ −21.7 (d, 1JBH ∼ 78 Hz), 1H NMR: δ 7.58 (N–H)]. Compound 9d was also characterized by X-ray diffraction (see Fig. 7 and Table 3). The analogous reaction of the pyrrolidine derived N/B Lewis pair (5e) with dihydrogen gave the H2-splitting product 9e. It was also characterized by an X-ray crystal structure analysis. Its structural features are similar to those of 9d (see the Supporting Information† for a view of the structure of 9e and further structural details).
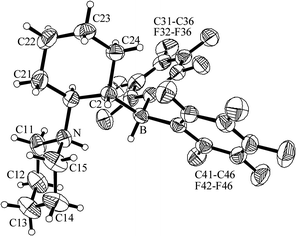 |
| Fig. 7 Molecular structure of compound 9d. | |
The hydrogen activation system 5b/9b is a metal-free catalyst for the hydrogenation of enamines. First we hydrogenated the enamines 4b and 4d at “ambient” conditions (i.e. 2.5 bar H2 pressure, at r.t. in toluene, 20h). For these experiments we used a rather large amount of the 9b catalyst and isolated the corresponding tert. amines in 83% (10b) and 53% (10d), respectively (see Scheme 6). The catalyst performance was better at 60 bar H2 pressure. Here, reasonable product conversions were obtained with 5 mol% of the 9b catalyst at room temperature.
The ethylene-bridged intramolecular frustrated P/B Lewis pair 1 and its congeners react rapidly with aldehydes by 1,2-addition to yield the respective six-membered heterocyclic products.19,22,23 We have reacted the cyclohexylene-bridged intramolecular frustrated N/B Lewis pair 5d with benzaldehyde and observed the formation of a different product type. From the reaction mixture we isolated the product 12a as a white crystalline solid. The X-ray crystal structure analysis revealed the formation of a new zwitterionic product type (Fig. 8). It contains an iminium ion functionality inside of the former piperidine ring (N1–C15: 1.277(3) Å, angle N–C15–C14: 124.4(2) Å). Apparently a hydride was abstracted in the α-position to nitrogen and transferred to the benzaldehyde reagent. Consequently, we find its reduced –O–CH2–Ph benzyl form attached at the intramolecular –B(C6F5)2 moiety of 12a (B–O3: 1.471(2) Å, O3–C4: 1.416(2) Å, angles C2–B–O3: 108.5(2)°, B–O3–C4: 119.6(1)°, O3–C4–C5: 108.7(2)°).
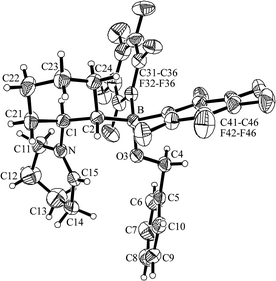 |
| Fig. 8 A projection of the molecular structure of compound 12a. | |
The NMR spectra confirmed the formation of the product 12a by an intramolecular disproportion reaction. There are several possible mechanistic pathways to explain this result. One is depicted in Scheme 7 which takes into account that strongly electrophilic boranes of the type R–B(C6F5)2 can abstract hydride anion from the α-CH position of amines to give iminium salts.16,18,24 We observed the 1H/13C NMR feature of the newly formed –CH
N+R– functionality of 12a at δ 8.45/173.9. The 11B NMR resonance of 12a is found at δ 1.6. The 19F NMR spectrum reveals the presence of a pair of diastereotopic C6F5 groups at boron and we observe the 1H NMR features of the newly formed benzyl –CH2[Ph] group at δ 4.14/3.83 [AB, 2JHH = 10.8 Hz; 13C: 67.8].
The reaction of 5d with benzophenone proceeded analogously to give the related trapping product 12b (for details including the X-ray crystal structure analysis see the Supporting Information†).
Conclusions
The series of enamines 4 used in this study cleanly undergo anti-Markovnikov hydroboration with Piers' borane HB(C6F5)2 to yield the intramolecular frustrated N/B Lewis pairs 5. The systems 5 feature a weak intramolecular N⋯B interaction. The chiral examples of 5 show activation barriers of N–B bond dissociation of ca. 13.5 kcal mol−1, which is slightly higher than it was observed for the corresponding intramolecular frustrated P/B Lewis pairs.13,25 Nevertheless, ring opening of the systems 5 is facile and, consequently, many of these compounds show typical frustrated Lewis pair behaviour, although they have a higher tendency of allowing competing reactions pathways to be followed. When the examples of 5 are not too bulky their nitrogen Lewis base components deprotonate C–H acidic acetylenes with formation of 7 and 8. Sterically non-encumbered examples of 5 rapidly cleave dihydrogen heterolytically to yield the respective ammonium/hydridoborate zwitterions (9). One of these systems (9b) is a reasonable metal-free catalyst for enamine hydrogenation. We conclude that the C2-bridged N/B Lewis pairs 5 in many respect resemble their corresponding C2-connected P/B pairs, but that their stability and frustrated Lewis pair reactivity seems to be somewhat lower. Nevertheless, some of the N/B examples 5 are as good FLPs as the extensively studied analogous P/B pairs. Since the N/B pairs should become easily available in a great structural variability by the introduced and described hydroboration route of readily available enamines we expect the chemistry of such hydrocarbylene-bridged N/B systems to become a very useful addition to frustrated Lewis pair chemistry.
Experimental
As a typical example we here describe the preparation of the N/B-FLP 5b and its reaction with dihydrogen to give the zwitterion 9b.
Preparation of 5b
N-(1-phenylethen-1-yl)piperidine (4b) (100 mg, 0.535 mmol) was added to a suspension of bis(pentafluorophenyl)borane (185 mg, 0.535 mmol) in pentane (10 mL). The reaction mixture was stirred for 20 min at room temperature. Then the precipitate was collected, washed with pentane (3 mL) and dried in vacuo to obtain 5b as a white solid (160 mg, 57%). Anal. Calc. C25H18BF10N: C 56.31, H 3.40, N 2.63; found: C 56.74, H 3.76, N 2.60. 1H NMR (600 MHz, 298 K, benzene-d6) δ = 7.06(1H)/7.03(2H)/7.02(2H) (Ph), 4.48 (dd, 3JHH = 13.2 Hz, 3JHH = 6.7 Hz, 1H, 1-H), 2.98/2.44, 2.85/2.59, 1.19/0.94, 0.77 (2H), 0.47/0.08 (each m, each 1H, C5H10N), 2.15 (dd, 2JHH = 13.6 Hz, 3JHH = 6.7 Hz, 1H, 2-H), 1.97 (dd, 2JHH = 13.6 Hz, 3JHH = 13.2 Hz, 1H, 2′-H). 13C{1H} NMR (151 MHz, 298 K, benzene-d6) δ = 136.6/130.7/129.6/128.7 (Ph), 75.7 (C1), 57.4/47.2/22.3/21.8/21.3 (C5H10N), 18.5 (C2) [C6F5 not listed]. 11B{1H} NMR (192 MHz, 298 K, toluene-d8) δ = 2.1. 19F NMR (564 MHz, 298 K, toluene-d8) δ = −127.9 (2F, o-C6F5A), −129.4 (2F, o-C6F5B), −156.0 (1F, p-C6F5B), −157.5 (1F, p-C6F5A), −163.1 (2F, m-C6F5B), −163.5 (2F, m-C6F5A).
Preparation of 9b
The reaction of compound 5b [generated in situ by using 4b (57 mg, 0.305 mmol) and HB(C6F5)2 (105 mg, 0.305 mmol) in pentane (5 mL)] with dihydrogen (2.5 bar) was completed after 2h stirring at room temperature. The solvent was removed and the residue was washed with pentane (3 × 5ml) to get 9b as a white solid (93 mg, 58%). 1H NMR (600 MHz, 298 K, benzene-d6) δ = 7.46 (br, 1H, N–H), 7.07 (1H)/7.00 (2H)/6.73 (2H) (Ph), 3.52 (d, 3JHH = 13.6 Hz, 1H, 1-H), 3.08 (br, 1
:
1
:
1
:
1 q, 1JBH ∼ 80 Hz, 1H, B-H), 2.66/1.13, 2.21/1.66, 1.03/0.80, 0.89/0.76, 0.77/−0.12 (each m, each 1H, C5H10N), 1.85/1.75 (each m, each 1H, 2-H). 13C{1H} NMR (151 MHz, 298 K, benzene-d6) δ = 133.5/130.3/129.9/128.8 (Ph), 73.7 (C1), 53.3/45.7/23.6/23.3/21.4 (C5H10N), 22.4 (br, C2), [C6F5 not listed]. 11B NMR (192 MHz, 298 K, benzene-d6) δ = −22.8 (d, 1JBH ∼ 80 Hz). 19F NMR (564 MHz, 298 K, benzene-d6) δ = −132.6 (m, 2F, o-C6F5A), −133.7 (m, 2F, o-C6F5B), −160.6 (m, 1F, p-C6F5B), −161.8 (m, 1F, p-C6F5A), −164.1 (m, 2F, m-C6F5B), −165.6 (m, 2F, m-C6F5A).
Acknowledgements
Financial support by the Deutsche Forschungsgemeinschaft is gratefully acknowledged.
Notes and references
- D. W. Stephan and G. Erker, Angew. Chem., 2010, 122, 50; D. W. Stephan and G. Erker, Angew. Chem., Int. Ed., 2010, 49, 46 CAS.
- D. Chen, Y. Wang and J. Klankermayer, Angew. Chem., 2010, 122, 9665 CrossRef; D. Chen, Y. Wang and J. Klankermayer, Angew. Chem., Int. Ed., 2010, 49, 9475 CrossRef CAS; M. Ullrich, A. J. Lough and D. W. Stephan, Organometallics, 2010, 29, 3647 CrossRef CAS; R. C. Neu, E. Y. Ouyang, S. J. Geier, X. Zhao, A. Ramos and D. W. Stephan, Dalton Trans., 2010, 39, 4285 RSC; C. Jiang, O. Blacque and H. Berke, Organometallics, 2009, 28, 5233 CrossRef CAS; A. Ramos, A. J. Lough and D. W. Stephan, Chem. Commun., 2009, 1119 Search PubMed; M. Ullrich, A. Lough and D. W. Stephan, J. Am. Chem. Soc., 2009, 131, 52 CrossRef CAS; D. P. Huber, G. Kehr, K. Bergander, R. Fröhlich, G. Erker, S. Tanino, Y. Ohki and K. Tatsumi, Organometallics, 2008, 27, 5279 CrossRef CAS; S. J Geier, T. M. Gilbert and D. W. Stephan, J. Am. Chem. Soc., 2008, 130, 12632 CrossRef CAS; P. A. Chase, G. C. Welch, T. Jurca and D. W. Stephan, Angew. Chem., 2007, 119, 8196 CrossRef; P. A. Chase, G. C. Welch, T. Jurca and D. W. Stephan, Angew. Chem., Int. Ed., 2007, 46, 8050 CrossRef CAS; G. C. Welch and D. W. Stephan, J. Am. Chem. Soc., 2007, 129, 1880 CrossRef CAS; G. C. Welch, L. Cabrera, P. A. Chase, E. Hollink, J. D. Masuda, P. Wei and D. W. Stephan, Dalton Trans., 2007, 3407 RSC.
- C. Jiang, O. Blacque, T. Fox and H. Berke, Organometallics, 2011, 30, 2117 CrossRef CAS; E. Theuergarten, D. Schüns, J. Grunenberg, C. G. Daniliuc, P. G. Jones and M. Tamm, Chem. Commun., 2010, 46, 8561 RSC; G. Erós, H. Mehdi, I. Pápai, T. A. Rakob, P. Király, G. Tárkányi and T. Soós, Angew. Chem., 2010, 122, 6709 CrossRef; G. Erós, H. Mehdi, I. Pápai, T. A. Rakob, P. Király, G. Tárkányi and T. Soós, Angew. Chem., Int. Ed., 2010, 49, 6559 CrossRef CAS; S. J. Geier, A. L. Gille, T. M. Gilbert and D. W. Stephan, Inorg. Chem., 2009, 48, 10466 CrossRef CAS; S. J. Geier and D. W. Stephan, J. Am. Chem. Soc., 2009, 131, 3476 CrossRef CAS; C. Jiang, O. Blacque and H. Berke, Chem. Commun., 2009, 5518 RSC; K. V. Axenov, G. Kehr, R. Fröhlich and G. Erker, Organometallics, 2009, 28, 5148 CrossRef CAS; V. Sumerin, F. Schulz, M. Nieger, M. Atsumi, C. Wang, M. Leskelä, P. Pyykkö, T. Repo and B. Rieger, J. Organomet. Chem., 2009, 694, 2654 CrossRef CAS; V. Sumerin, F. Schulz, M. Atsumi, C. Wang, M. Nieger, M. Leskelä, T. Repo, P. Pyykkö and B. Rieger, J. Am. Chem. Soc., 2008, 130, 14117 CrossRef CAS; V. Sumerin, F. Schulz, M. Nieger, M. Leskelä, T. Repo and B. Rieger, Angew. Chem., 2008, 120, 6090 CrossRef; V. Sumerin, F. Schulz, M. Nieger, M. Leskelä, T. Repo and B. Rieger, Angew. Chem., Int. Ed., 2008, 47, 5861 CrossRef.
- In some cases a nitrogen containing substrate in catalytic metal-free FLP hydrogenation can serve as the Lewis base: S. J. Geier, P. A. Chase and D. W. Stephan, Chem. Commun., 2010, 46, 4884 Search PubMed; K. V. Axenov, G. Kehr, R. Fröhlich and G. Erker, J. Am. Chem. Soc., 2009, 131, 3454 RSC; P. A. Chase, T. Jurca and D. W. Stephan, Chem. Commun., 2008, 1701 CrossRef CAS; D. Chen and J. Klankermayer, Chem. Commun., 2008, 2130 RSC.
- P. A. Chase, A. Gille, T. M. Gilbert and D. W. Stephan, Dalton Trans., 2009, 7179 RSC; D. Holschumacher, C. Taouss, T. Bannenberg, C. G. Hrib, C. G. Daniliuc, P. G. Jones and M. Tamm, Dalton Trans., 2009, 6927 RSC; P. A. Chase and D. W. Stephan, Angew. Chem., 2008, 120, 7543 CrossRef; P. A. Chase and D. W. Stephan, Angew. Chem., Int. Ed., 2008, 47, 7433 CrossRef CAS; D. Holschumacher, T. Bannenberg, C. G. Hrib, P. G. Jones and M. Tamm, Angew. Chem., 2008, 120, 7538 CrossRef; D. Holschumacher, T. Bannenberg, C. G. Hrib, P. G. Jones and M. Tamm, Angew. Chem., Int. Ed., 2008, 47, 7428 CrossRef CAS; G. D. Frey, V. Lavallo, B. Donnadieu, W. W. Schoeller and G. Bertrand, Science, 2007, 316, 439 CrossRef CAS.
- J. Spielmann, F. Buch and S. Harder, Angew. Chem., 2008, 120, 9576 CrossRef; J. Spielmann, F. Buch and S. Harder, Angew. Chem., Int. Ed., 2008, 47, 9434 CrossRef CAS; R. Noyori and T. Ohkuma, Angew. Chem., 2001, 113, 40 CrossRef; R. Noyori and T. Ohkuma, Angew. Chem., Int. Ed., 2001, 40, 40 CrossRef CAS; R. Noyori, M. Yamakawa and S. Hashiguchi, J. Org. Chem., 2001, 66, 7931 CrossRef CAS; T. Ohkuma, M. Koizumi, H. Ikehira, T. Yokozawa and R. Noyori, Org. Lett., 2000, 2, 659 CrossRef CAS;
T. Ohkuma and R. Noyori, Hydrogenation of Carbonyl Groups, Springer, Berlin, 1999 Search PubMed; C. Walling and L. Bollyky, J. Am. Chem. Soc., 1964, 86, 3750 Search PubMed; C. Walling and L. Bollyky, J. Am. Chem. Soc., 1961, 83, 2968 CrossRef CAS.
- D. W. Stephan, Chem. Commun., 2010, 46, 8526 RSC; D. W. Stephan, Dalton Trans., 2009, 3129 RSC; D. W. Stephan, Org. Biomol. Chem., 2008, 6, 1535 RSC; G. C. Welch, R. R. S. Juan, J. D. Masuda and D. W. Stephan, Science, 2006, 314, 1124 CrossRef CAS.
- P. Spies, G. Erker, G. Kehr, K. Bergander, R. Fröhlich, S. Grimme and D. W. Stephan, Chem. Commun., 2007, 5072 RSC.
- S. Schwendemann, T. A. Tumay, K. V. Axenov, I. Peuser, G. Kehr, R. Fröhlich and G. Erker, Organometallics, 2010, 29, 1067 CrossRef CAS; P. Spies, S. Schwendemann, S. Lange, G. Kehr, R. Fröhlich and G. Erker, Angew. Chem., 2008, 120, 7654 CrossRef; P. Spies, S. Schwendemann, S. Lange, G. Kehr, R. Fröhlich and G. Erker, Angew. Chem., Int. Ed., 2008, 47, 7543 CrossRef CAS.
- H. Wang, R. Fröhlich, G. Kehr and G. Erker, Chem. Commun., 2008, 5966 RSC.
- S. Grimme, H. Kruse, L. Goerigk and G. Erker, Angew. Chem., 2010, 122, 1444; S. Grimme, H. Kruse, L. Goerigk and G. Erker, Angew. Chem., Int. Ed., 2010, 49, 1402 CAS.
- For other theoretical FLP studies, see: e.g. B. Schirmer and S. Grimme, Chem. Commun., 2010, 46, 7942 Search PubMed; S. Gao, W. Wu and Y. Mo, J. Phys. Chem. A, 2009, 113, 8108 RSC; T. A. Rokob, A. Hamza and I. Pápai, J. Am. Chem. Soc., 2009, 131, 10701 CrossRef CAS; T. A. Rokob, A. Hamza, A. Stirling and I. Pápai, J. Am. Chem. Soc., 2009, 131, 2029 CrossRef CAS; T. Privalov, Chem.–Eur. J., 2009, 15, 1825 CrossRef; T. A. Rokob, A. Hamza, A. Stirling, T. Soós and I. Pápai, Angew. Chem., 2008, 120, 2469 CrossRef CAS; T. A. Rokob, A. Hamza, A. Stirling, T. Soós and I. Pápai, Angew. Chem., Int. Ed., 2008, 47, 2435 CrossRef; T. Privalov, Dalton Trans., 2009, 1321 CrossRef CAS; Y. Guo and S. Li, Inorg. Chem., 2008, 47, 6212 RSC; A. Stirling, A. Hamza, T. A. Rokob and I. Pápai, Chem. Commun., 2008, 3148 CrossRef CAS.
- K. Axenov, C. M. Mömming, G. Kehr, R. Fröhlich and G. Erker, Chem.–Eur. J., 2010, 16, 14069 CrossRef CAS.
- J. Paleček and O. Paleta, Synthesis, 2004, 4, 521; R. Carlson and A. Nilsson, Acta Chem. Scand., Ser. B, 1984, 38, 49; W. White and H. Winegarten, J. Org. Chem., 1967, 32, 213 CrossRef CAS.
- D. J. Parks, W. E. Piers and G. P. A. Yap, Organometallics, 1998, 17, 5492 CrossRef CAS; W. E. Piers and T. Chivers, Chem. Soc. Rev., 1997, 26, 345 RSC; D. J. Parks, R. E.v H. Spence and W. E. Piers, Angew. Chem., 1995, 107, 895; D. J. Parks, R. E.v H. Spence and W. E. Piers, Angew. Chem., Int. Ed. Engl., 1995, 34, 809 CrossRef CAS.
- For a comparison: B(C6F5)3–NH(C5H10) B–N: 1.628(2) Å; B(C6F5)3–N (C2H5)3 B–N: 1.633(4) Å. See: F. Focante, P. Mercandelli, A. Sironi and L. Resconi, Coord. Chem. Rev., 2006, 250, 170 Search PubMed; A. J. Mountford, D. L. Hughes and S. J. Lancaster, Chem. Commun., 2003, 2148 CrossRef CAS.
- T. Beringhelli, D. Donghi, D. Maggioni and G. D'Alfonso, Coord. Chem. Rev., 2008, 252, 2292 CrossRef CAS.
- G. Erker, Dalton Trans., 2005, 1883 RSC; W. E. Piers, Adv. Organomet. Chem., 2004, 52, 1.
- See for a comparison: C. M. Mömming, S. Frömel, G. Kehr, R. Fröhlich, S. Grimme and G. Erker, J. Am. Chem. Soc., 2009, 131, 12280 Search PubMed.
- For other reaction modes of alkynes with FLPs see: e.g. M. A. Dureen, C. C. Brown and D. W. Stephan, Organometallics, 2010, 29, 6594 Search PubMed; C. Chen, G. Kehr, R. Fröhlich and G. Erker, J. Am. Chem. Soc., 2010, 132, 13594 CrossRef CAS; C. Chen, F. Eweiner, B. Wibbeling, R. Fröhlich, S. Senda, Y. Ohki, K. Tatsumi, S. Grimme, G. Kehr and G. Erker, Chem.–Asian J., 2010, 5, 2199 CrossRef CAS; T. Voss, C. Chen, G. Kehr, E. Nauha, G. Erker and D. W. Stephan, Chem.–Eur. J., 2010, 16, 3005 CrossRef CAS; S. Geier, M. A. Dureen, E. Y. Ouyang and D. W. Stephan, Chem. Eur. J., 2010, 16, 988 CrossRef CAS; C. Jiang, O. Blacque and H. Berke, Organometallics, 2010, 29, 125 CAS; M. A. Dureen and D. W. Stephan, J. Am. Chem. Soc., 2009, 131, 8396 CrossRef CAS.
- For FLP reactions with non-conjugated diynes see: e.g. C. Chen, R. Fröhlich, G. Kehr and G. Erker, Chem. Commun., 2010, 46, 3580 Search PubMed.
- C. M. Mömming, G. Kehr, B. Wibbeling, R. Fröhlich and G. Erker, Dalton Trans., 2010, 39, 7556 RSC.
- C. M. Mömming, G. Kehr, B. Wibbeling, R. Fröhlich, B. Schirmer, S. Grimme and G. Erker, Angew. Chem., 2010, 122, 2464; C. M. Mömming, G. Kehr, B. Wibbeling, R. Fröhlich, B. Schirmer, S. Grimme and G. Erker, Angew. Chem., Int. Ed., 2010, 49, 2414.
- P. Liptau, M. Neumann, G. Erker, G. Kehr, R. Fröhlich and S. Grimme, Organometallics, 2004, 22, 21 CrossRef; S. Guidotti, I. Camurati, F. Focante, L. Angellini, G. Moscardi, L. Resconi, R. Leardini, D. Nanni, P. Mercandelli, A. Sironi, T. Beringhelli and D. Maggioni, J. Org. Chem., 2003, 68, 5445 CrossRef CAS; N. Millot, C. C. Santini, B. Fenet and J.-M. Basset, Eur. J. Inorg. Chem., 2002, 3328 CrossRef CAS.
- P. Spies, G. Kehr, K. Bergander, B. Wibbeling, R. Fröhlich and G. Erker, Dalton Trans., 2009, 1534 RSC.
Footnotes |
† Electronic supplementary information (ESI) available: Synthetic details and characterization of the new compounds. CCDC reference numbers 815781–815791. For ESI and crystallographic data in CIF or other electronic format see DOI: 10.1039/c1sc00124h |
‡ X-ray crystal structure analyses. |
|
This journal is © The Royal Society of Chemistry 2011 |