DOI:
10.1039/C0SC00613K
(Edge Article)
Chem. Sci., 2011,
2, 807-810
Enantioselective silver-catalyzed propargylation of imines†
Received
6th December 2010
, Accepted 5th January 2011
First published on 28th January 2011
Abstract
A catalytic enantioselective method for the propargylation of aldimines is described. A silver catalyst ligated by a bidentate phoshpine is utilized to provide good yields and high ee's for a variety of substrates. Homopropargylic sulfonamide products undergo facile silver-mediated cyclization to generate 2-pyrrolines, useful intermediates for alkaloid synthesis.
Introduction
Addition of nucleophilic reagents to carbonyl derivatives has provided a wealth of methods for the formation of C–C bonds with control of absolute stereochemistry. Addition of functionalized nucleophiles provides further synthetic versatility, as subsequent derivatization allows for preparation of complex synthetic intermediates. Propargylation reactions of carbonyl derivatives provide products with orthogonal functional groups for subsequent elaboration. Enantioselective propargylation reactions of aldehydes and ketones and highly diastereoselective propargylation reactions of sulfinyl imines have been reported.1,2,3 In this communication we report the first catalytic enantioselective propargylation reactions of imines (eqn (1)). |  | (1) |
Recently our laboratory has developed a palladium catalyzed annulation reaction that is initiated by conjugate allenylation of benzylidine malononitriles.4 We postulated that a silver complex would catalyze selective reactions of different electrophiles, including imines. We envisioned that the transformation could proceed through the catalytic cycle in Fig. 1. Transmetallation of a silver catalyst with an allenyl boronic acid pinacol ester (Bpin) initially provides a propargylic silver species I.5 This intermediate then equilibrates to form its allenyl silver counterpart II. We hypothesized that the relative nucleophilicities of these complexes could be modulated by ligand tuning.6 For example, the appropriate ligand would accelerate reaction of the allenylsilver complex II, providing the propargylated product with high selectivity.
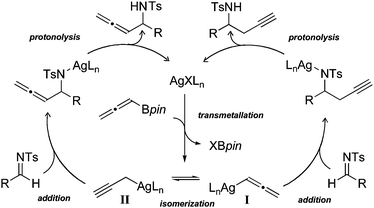 |
| Fig. 1 Proposed catalytic cycle. | |
Results and discussion
Initial studies
We began our studies by examining AgF as a catalyst in combination with bidentate phosphine ligands. In the absence of exogenous ligand, silver fluoride provided a 1
:
1 mixture of allenylation and propargylation products (Table 1, entry 1). As expected, the introduction of bidentate phosphine ligands influenced the product distribution, and generally favored formation of propargylation product 3a (Table 1, entries 2–4). Walphos-1 and MeDuPhos provided promising starting points for the development of an enantioselective transformation (Table 1, entry 4 and 5). The selectivity of the Walphos-1 ligated catalyst improved significantly at −20 °C, providing homopropargylic amine as the major product and 92% ee. This dramatic increase in selectivity is consistent with a competitive racemic pathway that is suppressed at reduced temperature. We hypothesized that incomplete ligation of silver by the phosphine would leave catalytically active AgF in solution at room temperature. At −20 °C, however, AgF would be insoluble and only phosphine-ligated silver complexes would be present in solution. Consistent with this hypothesis, pre-stirring AgF with Walphos-1 in methanol to affect complex formation7 noticeably improved the yield as well as the selectivity of the transformation (Table 1, entries 9, 10).
Table 1 Enantioselective propargylation of aldimines
Entry |
Ligand |
Temperature |
Yielda2a(%) |
ee 2a(%) |
Yielda3a(%) |
ee 3a(%) |
Isolated yield after column chromatography.
Catalyst pre-formed in methanol.
Reaction time = 3 h.
Reaction time = 6 h.
|
1 |
none |
RT |
34 |
nd |
32 |
nd |
2 |
Et-Ferrotane |
RT |
trace |
nd |
28 |
7 |
3 |
Chiraphos |
RT |
22 |
0 |
79 |
4 |
4 |
Me-Duphos |
RT |
9 |
2 |
54 |
29 |
5 |
Walphos W001-1 |
RT |
38 |
0 |
32 |
35 |
6 |
Me-Duphos |
−20 °C |
28 |
nd |
63 |
39 |
7 |
Walphos W001-1 |
−20 °C |
trace |
nd |
47 |
92 |
8 |
Walphos W002 - 1 |
−20 °C |
trace |
nd |
45 |
11 |
9b,c |
Walphos W001-1 |
RT |
trace |
nd |
85 |
88 |
10b,d |
Walphos W001-1 |
−20 °C |
trace |
nd |
90 |
97 |
The electronic environment at the metal center heavily influences the stereoselectivity of this transformation. In our initial ligand screening, Walphos-1, which contains electron-withdrawing trifluoromethyl subsitutents on one phosphine, provided the desired product in 92% ee (Table 1, entry 7).‡ In contrast, Walphos-2, which does not include electron-withdrawing groups, afforded the product in a modest 11% ee (Table 1, entry 8).
Synthetic scope
A variety of substrates underwent highly enantioselective propargylation under the optimized reaction conditions (Table 2).§, The presence of either electron-donating (entry 6) or electron-withdrawing groups on the aryl substituent (entry 3) was tolerated by the catalyst. Naphthaldehyde derived imine 1f performed well despite its greater steric bulk (entry 7). Heteroatom substitution was also tolerated providing the furyl product 3g in 93% yield and 97% ee (entry 7). Although the reaction of conjugated aldimine 1h was slower and product 3h was obtained in modest yield, the ee was still very good (entry 9). Alkyl imine 1i, with a fully substituted carbon in the α position, demonstrates the boundaries of the scope, as it afforded product 1i in modest yield and with 74% ee. The increased steric bulk of this substrate next to the electrophilic site could be responsible for the decreased rate and enantioselectivity of the transformation. Substitution of the sulfonyl group was also possible, as nosyl imine 2 afforded product 4 in good yield and high ee (entry 5). The decreased yield of this transformation is most likely caused by poor solubility of the substrate in THF. Despite the lower yield, nosyl-protected homopropargyl amines are useful products due to the relative ease of sulfonamide deprotection.
Entry |
R1 |
Ar |
Substrate |
Product |
Yield (%) |
ee (%) |
An additional 2 equiv of allenyl Bpin was added after 4 h. Reaction time = 16 h.
Reaction performed in DMF instead of THF. Reaction time = 16 h.
|
1 |
Ts |
|
1a, R2 = H |
3a
|
91 |
96 |
2 |
Ts |
1b, R2 = 4-Br |
3b
|
73 |
98 |
3 |
Ts |
1c, R2 = 4-CF3 |
3c
|
96 |
96 |
4 |
Ts |
1d, R2 = 2-Br |
3d
|
74 |
95 |
5a |
Ns |
4, R2 = H |
5
|
70 |
92 |
6b |
Ts |
|
1e
|
3e
|
99 |
90 |
7 |
Ts |
|
1f
|
3f
|
81 |
93 |
8 |
Ts |
|
1g
|
3g
|
93 |
97 |
9a |
Ts |
|
1h
|
3h
|
47 |
89 |
10 |
Ts |
|
1i
|
3i
|
40 |
74 |
By virtue of incorporation of orthogonal functional groups, the homopropargylic sulfonamide products generated in this reaction are useful building blocks for synthesis. For example, related compounds have been used as precursors for pyridoindoles.3 Furthermore, homopropargylic amines can be derivatized to furnish 2-pyrrolines, enamines that have proven utility in synthesis of bioactive pyrrolidines and alkaloids.8 Intramolecular silver-mediated hydroamination transforms 3a to 2-pyrroline 6 in excellent yield (eqn (2)).9 While the formation of Markovnikov products is generally preferred in hydroamination reactions of alkynes, the ring strain present in four-membered rings forces the homopropargyl amine to undergo a 5-endo-digcyclization resulting in anti-Markovnikov addition.10,11 As anticipated, the cyclization reaction does not effect the newly formed stereogenic center and provides enantioenriched 2-pyrroline.
| 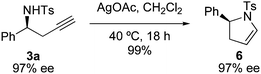 | (2) |
Conclusion
We have developed an enantioselective silver-catalyzed propargylation reaction of aldimines. This transformation is tolerant of substitution on the aromatic ring of the substrate with little erosion of enantioselectivity. Products undergo facile silver-mediated cyclization reactions to provide 2-pyrrolines. Efforts towards expansion of the substrate scope and elucidation of mechanism are underway.
Acknowledgements
This work was supported by an NSF CAREER Award (CHE-0847273) and GAANN fellowship for HMW. Mr. Benjamin Kohn is acknowledged for preliminary studies of related reactions. We thank Solvias and Frontier Scientific for donations of ligands and allenylboronic ester, respectively.
Notes and references
- For catalytic asymmetric aldehyde propargylation, see:
(a) G. E. Keck, D. Krishnamurthy and X. Chen, Tetrahedron Lett., 1994, 35, 8323 CrossRef CAS;
(b) C.-M. Yu, S.-K. Yoon, K. Baek and J.-Y. Lee, Angew. Chem., Int. Ed., 1998, 37, 2392 CrossRef CAS;
(c) D. A. Evans, Z. K. Sweeney, T. Rovis and J. Tedrow, J. Am. Chem. Soc., 2001, 123, 12095 CrossRef CAS;
(d) S. E. Denmark and T. Wynn, J. Am. Chem. Soc., 2001, 123, 6199 CrossRef CAS;
(e) G. Xia and H. Yamamoto, J. Am. Chem. Soc., 2007, 129, 496 CrossRef CAS;
(f) D. R. Fandrick, K. R. Fandrick, J. T. Reeves, Z. Tan, W. Tang, A. G. Capacci, S. Rodriguez, J. J. Song, H. Lee, N. K. Yee and C. H. Senanayake, J. Am. Chem. Soc., 2010, 132, 7600 CrossRef CAS.
- For catalytic asymmetric propargylation of ketones, see: S.-L. Shi, L.-W. Xu, K. Oisaki, M. Kanai and M. Shibasaki, J. Am. Chem. Soc., 2010, 132, 6638 Search PubMed.
- For diastereoselective imine propargylation, see: D. R. Fandrick, C. S. Johnson, K. R. Fandrick, J. T. Reeves, Z. Tan, H. Lee, J. J. Song, N. K. Yee and C. H. Senanayake, Org. Lett., 2010, 12, 748 Search PubMed.
-
B. L. Kohn and E. R. Jarvo, 2010, Manuscript in preparation.
- For examples of transmetallation and/or isomerization of allenylmetal complexes, see:
(a) J. Marshall, J. Org. Chem., 2007, 72, 8153 CrossRef CAS;
(b) J. Marshall, Chem. Rev., 1996, 96, 31 CrossRef CAS;
(c) E. J. Corey, C. M. Yu and D.-H. Lee, J. Am. Chem. Soc., 1990, 112, 878 CrossRef CAS;
(d) Y. Tamaru, S. Goto, A. Tanaka, M. Shimizu and M. Kimura, Angew. Chem., Int. Ed. Engl., 1996, 35, 878 CrossRef CAS;
(e) Reference 1b ;
(f) D. R. Fandrick, K. R. Fandrick, J. T. Reeves, Z. Tan, C. S. Johnson, H. Lee, J. J. Song, N. K. Yee and C. H. Senanayake, Org. Lett., 2010, 12, 88 CrossRef CAS;
(g)
T. Harada and A. Oku in Oranozinc Reagents: A Practical Approach, P. Knochel and P. Jones, ed.; Oxford Univ. Press: Oxford, 1999; 101 Search PubMed.
- J. Halpern, Science, 1982, 217, 401 CrossRef CAS.
- M. Wadamoto and H. Yamamoto, J. Am. Chem. Soc., 2005, 127, 14556 CrossRef CAS.
- For select examples, see:
(a) V. H. Rawal, C. Michoud and R. F. Monestel, J. Am. Chem. Soc., 1993, 115, 3030 CrossRef CAS;
(b) U. Gross, M. Nieger and S. Bräse, Org. Lett., 2009, 11, 4740 CrossRef CAS;
(c) T. D. Michel, M. J. Kier, A. M. Kearney and C. D. Vanderwal, Org. Lett., 2010, 12, 3093 CrossRef;
(d) U. Chiacchio, L. Borrello, L. Crispino, A. Rescifina, P. Merino, B. Macchi, E. Balestrieri, A. Mastino, A. Piperno and G. Romeo, J. Med. Chem., 2009, 52, 4054 CrossRef CAS;
(e) P. A. Wender and D. Strand, J. Am. Chem. Soc., 2009, 131, 7528 CrossRef CAS.
- R. Martin, B. M. Jäger, S. Richter, R. Fedorov, D. Manstein, H. O. Gutzeit and H.-J. Knölker, Angew. Chem., Int. Ed., 2009, 48, 8042 CrossRef CAS.
-
(a) G. A. Molander and H. Hasegawa, Heterocycles, 2004, 64, 467 CAS;
(b) T. E. Müller, K. C. Hultzsch, M. Yus, F. Foubelo and M. Tada, Chem. Rev., 2008, 108, 3795 CrossRef.
- For examples of 5-endo-dighydroamination reactions of free amines, see:
(a) T. E. Müller, M. Grosche, E. Herdtweck, A.-K. Pleier, E. Walter and Y.-K. Yan, Organometallics, 2000, 19, 170 CrossRef;
(b) S. Burling, L. D. Field, B. A. Messerle and P. Turner, Organometallics, 2004, 23, 1714 CrossRef CAS.
- For a discussion of non-linear effects, see: D. Guillaneus, S.-H. Zhao, O. Samuel, D. Rainford and H. B. Kagan, J. Am. Chem. Soc., 1994, 116, 9430 Search PubMed.
Footnotes |
† Electronic supplementary information (ESI) available: Experimental procedures and spectroscopic and analytical data for all new compounds. See DOI: 10.1039/c0sc00613k |
‡ This observation could be consistent with a mechanism wherein two silver catalysts, with distinct reaction roles, are involved. For example, one catalyst could generate a nucleophilic propargylsilver complex and a second could serve as a Lewis acid to activate the imine. To probe for the involvement of two catalysts in the catalytic cycle, the presence of a nonlinear effect was investigated. A modest negative non-linear effect was observed (see ESI† for details), consistent with more than one silver complex being involved in the transition state of this transformation.12 |
§ Absolute configuration of 3a was assigned based on reduction and comparison of optical rotation to values reported in the literature. The absolute configuration of the remaining products is inferred. See ESI† for details. |
|
This journal is © The Royal Society of Chemistry 2011 |
Click here to see how this site uses Cookies. View our privacy policy here.