DOI:
10.1039/C1RA00377A
(Communication)
RSC Adv., 2011,
1, 758-760
Flash synthesis of TAC-101 and its analogues from 1,3,5-tribromobenzene using integrated flow microreactor systems†
Received
28th June 2011
, Accepted 10th August 2011
First published on 7th September 2011
Abstract
Sequential introduction of three electrophiles into 1,3,5-tribromobenzene based on Br/Li exchange reaction was achieved using an integrated flow microreactor system consisting of six micromixers and six microtube reactors. This method enables flash synthesis of TAC-101 and its analogues within 13 s in good yields on a preparative scale.
Chemical synthesis of biologically active complex molecules serves not only as powerful tools for biological and medicinal studies but also as methods for producing useful drugs in pharmaceutical industry.1 However, synthesis of complex molecules is often labour-intensive and time-consuming. To meet future demands, the speed and efficiency of chemical synthesis should be greatly enhanced. For this purpose, the integration of chemical reactions has received significant research interest.2 Organic synthesis has been often performed by stepwise formation of the individual bonds in a target molecule. However, it would be much more efficient if one could form several bonds in one sequence without isolating the intermediates.3 One-pot sequential reaction is an effective method for carrying out several transformations and forming several bonds in a single vessel.4 The reaction sequence can be also changed, in principle, by changing the order of the addition of reaction components. However, it is rather difficult to use a short-lived intermediate for the next sequence, because the minimal interval of the addition of reaction components in practice is often much longer than the lifetimes of such intermediates. In contrast, extensive efforts have been recently devoted to developing a new method for the integration of reactions, a continuous flow method, in which a sequence of reactions is conducted in a flow mode by adding components at different places.5 Especially, continuous flow microreactor systems6,7 enable the use of a short-lived intermediate because the residence time can be greatly reduced to the order of milliseconds, keeping the flexibility of the addition order of reaction components (flash chemistry)8,9. Moreover, continuous flow microreactor synthesis can be used not only for preparative scale laboratory synthesis but also for production of chemical substances on industrial scales.10
Retinoids including vitamin A function as ligands for two classes of nuclear hormone receptors such as the retinoic acid receptors (RARs) and the retinoid X receptors (RXRs), and regulate the morphogenesis, development, growth, and differentiation of cells.11 TAC-101 (4-[3,5-bis(trimethylsilyl)benzamido]benzoic acid) is a synthetic retinoid with selective binding affinity for RAR-α binding. Shudo et al. have reported a synthetic process for the preparation of TAC-101 in five steps from 1,3,5-tribromobenzene as a starting material. However, total yield is very low (3.4%).12 Recently, Tamanoi and Nishihara reported that the short-step synthesis of TAC-101 analogues can be achieved by Rh-catalyzed arylation of hydrosilane as a key step from 3,5-diiodobenzoic acid as a starting material (55% total yield).13 It is important to note that these methods cannot be applied, in principle, to the synthesis of compounds bearing two different silyl groups. Therefore, more efficient, versatile, and practical synthesis of TAC-101 and its analogues has been strongly needed.
We describe herein that the continuous flow microreactor method serves as a highly efficient, versatile, and practicable synthesis of TAC-101 and its analogues. The retrosynthetic strategy of the flow microreactor synthesis is shown in Fig. 1. As a key consideration, we intended to integrate three sets of Br/Li exchange reaction followed by reaction with an electrophile in space starting from 1,3,5-tribromobenzene. The present strategy allows the introduction of two different silyl groups to synthesize a variety of TAC-101 analogues.
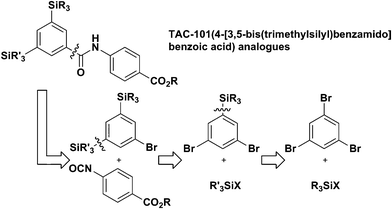 |
| Fig. 1 Retrosynthetic strategy for TAC-101 analogues. | |
First, we focused on the Br/Li exchange reaction of 1,3,5-tribromobenzene to generate 3,5-dibromophenyllithium, which is the first step of the synthesis. It was reported that the generation and reactions of 3,5-dibromophenyllithium by the Br/Li exchange reaction of 1,3,5-tribromobenzene in THF in a conventional batch macro reactor (flask) gave rise to the formation of various by-products at −78 °C.14 In fact, Br/Li exchange reaction of 1,3,5-tribromobenzene with n-BuLi followed by the reaction with methanol at −78 °C in a 20 mL flask gave the desired product in only moderate yield (76%) and small amount of bromobenzene was produced as a main by-product (5%) (See the Supplementary Information for details†).
Thus, generation of 3,5-dibromophenyllithium by Br/Li exchange reaction of 1,3,5-tribromobenzene followed by reaction with trimethylsilyl triflate was carried out using a flow microreactor system composed of two T-shaped micromixers (M1 and M2) and two microtube reactors (R1 and R2) shown in Fig. 2a. The desired product, 1,3-dibromo-5-(trimethylsilyl)benzene was obtained in high yield (88%) at 0 °C at the residence time (tR1) of 0.22 s. Further increase in tR1 caused the decrease in the yield as shown in Fig. 2b. Moreover, under the optimized conditions (0 °C, tR1 = 0.22 s, tR2 = 2.2 s), the reaction with other silylating reagents such as triethylsilyl triflate, chlorodimethylhydrosilane, and chlorodimethylphenylsilane gave the corresponding mono-silylated dibromobenzenes in good yields (Fig. 2c).
With selective generation of 3,5-dibromophenyllithium followed by monosilylation in hand, we next examined the sequential introduction of two trimethylsilyl groups by repeating the sequence consisting of the Br/Li exchange reaction and the subsequent silylation using an integrated flow microreactor system composed of four micromixers (M1, M2, M3 and M4) and four microtube reactors (R1, R2, R3 and R4) shown in Fig. 3a. At 0 °C, the yield reached the maximum at tR3 = 0.14 s, and further increase in tR3 caused a decrease in the yield (Fig. 3b). Under the optimized conditions (0 °C, tR1 = 0.22 s, tR2 = 2.2 s, tR3 = 0.14 s), the sequential introduction of two different silyl groups was achieved with various combinations of silylating reagents without the isolating a monosilylated dibromobenzene as shown in Fig. 3c.
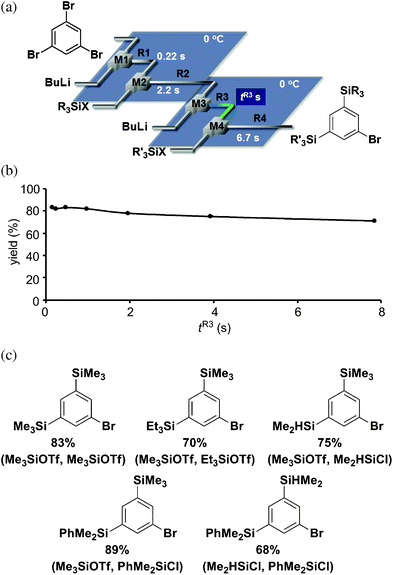 |
| Fig. 3 (a) An integrated flow microreactor system for sequential introduction of two silyl groups to 1,3,5-tribromobenzene. (b) Effects of residence time in R3 at 0 °C on the yield of 1-bromo-3,5-bis(trimethylsilyl)benzene using trimethylsilyl triflate as a silylating reagent. (c) The structures and the yields of 1-bromo-3,5-disilylbenzenes. Silylating reagents are shown in parenthesis (left: first silylating reagent, right: second silylating reagent). | |
Finally, we examined the sequential introduction of two silyl groups followed by introduction of amide functionality using an integrated flow microreactor system composed of six micromixers (M1, M2, M3, M4, M5 and M6) and six microtube reactors (R1, R2, R3, R4, R5 and R6) shown in Fig. 4a. The desired product, methyl 4-[3,5-bis(trimethylsilyl)benzamido]benzoate was obtained in a high yield at the residence time of 1.5 s in R5 as shown in Fig. 4b. Various TAC-101 methyl ester analogues having two different silyl groups could be synthesized from 1,3,5-tribromobenzene in one flow within 12.2 s in high yields shown in Fig. 4c. In addition, the productivity ranged from 132–194 mg per minute depending on the nature of the silyl groups. The reaction could be continuously carried out for at least 5 min and the yields were stable during the course of the operation, although elongated operation was difficult because of the limitation of the syringe capacity for syringe pumping, Finally, the hydrolysis with NaOH in ethanol gave the corresponding carboxylic acids in 91–99% yields.
![(a) An integrated flow microreactor system for synthesis of TAC-101 analogues. (b) Effects of residence time in R5 at 0 °C on the yield of methyl 4-[3,5-bis(trimethylsilyl)benzamido]benzoate. (c) The structures and the yields of TAC-101 analogues. Silylating reagents are shown in parenthesis (left: first silylating reagent, right: second silylating reagent).](/image/article/2011/RA/c1ra00377a/c1ra00377a-f4.gif) |
| Fig. 4 (a) An integrated flow microreactor system for synthesis of TAC-101 analogues. (b) Effects of residence time in R5 at 0 °C on the yield of methyl 4-[3,5-bis(trimethylsilyl)benzamido]benzoate. (c) The structures and the yields of TAC-101 analogues. Silylating reagents are shown in parenthesis (left: first silylating reagent, right: second silylating reagent). | |
In conclusion, we have developed an effective method for the synthesis of TAC-101 analogues by integration of three sequences consisting of the Br/Li exchange reaction and the subsequent reaction with an electrophile. The present results demonstrate that flash chemistry using integrated flow microreactor systems is quite effective for multi-step synthesis of biologically interesting molecules on a preparative scale.
References
-
K. C. Nicolaou and T. Montagnon, Molecules that Changed the World, Wiley-VCH, Weinheim, 2008 Search PubMed.
-
(a) L. F. Tietze, Chem. Rev., 1996, 96, 115 CrossRef CAS;
(b) I. Ryu and N. Sonoda, Chem. Rev., 1996, 96, 177 CrossRef CAS;
(c) P. J. Parsons, C. S. Penkett and A. J. Shell, Chem. Rev., 1996, 96, 195 CrossRef CAS;
(d) J. Louie, C. W. Bielawski and R. H. Grubbs, J. Am. Chem. Soc., 2001, 123, 11312 CrossRef CAS;
(e) D. Enders, M. R. M. Hüttl, C. Grondal and G. Raabe, Nature, 2006, 441, 861 CrossRef CAS;
(f) G. Babu, A. Orita and J. Otera, Chem. Lett., 2008, 37, 1296 CrossRef CAS;
(g) M. Shimizu, K. Shimono and T. Hiyama, Chem. Lett., 2006, 35, 838 CrossRef CAS;
(h) H. Fuwa, T. Tako, M. Ebine and M. Sasaki, Chem. Lett., 2008, 37, 904 CrossRef CAS;
(i) S. Ikeda, M. Shibuya, N. Kanoh and Y. Iwabuchi, Chem. Lett., 2008, 37, 962 CrossRef CAS;
(j) T. A. Cernak and T. H. Lambert, J. Am. Chem. Soc., 2009, 131, 3124 CrossRef CAS;
(k) D. J. Hardee and T. H. Lambert, J. Am. Chem. Soc., 2009, 131, 7536 CrossRef CAS;
(l) A. Dömling and I. Ugi, Angew. Chem., Int. Ed., 2000, 39, 3168 CrossRef;
(m) H. Bienaymé, C. Hulme, O. Oddon and P. Schmitt, Chem.–Eur. J., 2000, 6, 3321 CrossRef;
(n) G. Balme, E. Bossharth and N. Monteiro, Eur. J. Org. Chem., 2003, 4101 CrossRef CAS;
(o) W. Stacy and M. G. Orga, J. Comb. Chem., 2007, 9, 14 CrossRef;
(p) I. Ugi, Pure Appl. Chem., 2001, 73, 187 CrossRef CAS.
- P. A. Wender, V. A. Verma, T. J. Paxton and T. H. Pillow, Acc. Chem. Res., 2008, 41, 40 CrossRef CAS.
-
(a) A. Orita, J. Yaruva and J. Otera, Angew. Chem., Int. Ed., 1999, 38, 2267 CrossRef;
(b) A. Orita, N. Yoshioka, P. Struwe, A. Braier, A. Beckmann and J. Otera, Chem.–Eur. J., 1999, 5, 1355 CrossRef CAS;
(c) P. A. Clarke, S. Santos and W. H. C. Martin, Green Chem., 2007, 9, 438 RSC;
(d) T. Nokami, H. Tsuyama, A. Shibuya, T. Nakatsutsumi and J. Yoshida, Chem. Lett., 2008, 37, 942 CrossRef CAS;
(e) Y. Numata, J. Kawashima, T. Hara and Y. Tajima, Chem. Lett., 2008, 37, 1018 CrossRef CAS;
(f) K. Yamaguchi, M. Kotani, K. Kamata and N. Mizuno, Chem. Lett., 2008, 37, 1258 CrossRef CAS.
-
(a) S. Suga, D. Yamada and J. Yoshida, Chem. Lett., 2010, 39, 404 CrossRef CAS;
(b) J. Yoshida, K. Saito, T. Nokami and A. Nagaki, Synlett, 2011, 9, 1189 CrossRef.
-
(a) P. D. I. Fletcher, S. J. Haswell, E. Pombo-Villar, B. H. Warrington, P. Watts, S. Y. F. Wong and X. Zhang, Tetrahedron, 2002, 58, 4735 CrossRef CAS;
(b) K. Jähnisch, V. Hessel, H. Löwe and M. Baerns, Angew. Chem., Int. Ed., 2004, 43, 406 CrossRef;
(c) G. N. Doku, W. Verboom, D. N. Reinhoudt and A. van den Berg, Tetrahedron, 2005, 61, 2733 CrossRef CAS;
(d) P. Watts and S. J. Haswell, Chem. Soc. Rev., 2005, 34, 235 RSC;
(e) J. Yoshida, A. Nagaki, T. Iwasaki and S. Suga, Chem. Eng. Technol., 2005, 3, 259 CrossRef;
(f) J. Kobayashi, Y. Mori and S. Kobayashi, Chem.–Asian J., 2006, 1, 22 CrossRef CAS;
(g) T. Fukuyama, M. T. Rahman, M. Sato and I. Ryu, Synlett, 2008, 2, 151 Search PubMed;
(h) J. Yoshida, A. Nagaki and T. Yamada, Chem.–Eur. J., 2008, 14, 7450 CrossRef CAS;
(i) J. P. McMullen and K. F. Jensen, Annu. Rev. Anal. Chem., 2010, 3, 19 CrossRef CAS;
(j) J. Yoshida, H. Kim and A. Nagaki, ChemSusChem., 2011, 4, 331 CrossRef CAS.
-
(a) S. Suga, M. Okajima, K. Fujiwara and J. Yoshida, J. Am. Chem. Soc., 2001, 123, 7941 CrossRef CAS;
(b) A. Nagaki, K. Kawamura, S. Suga, T. Ando, M. Sawamoto and J. Yoshida, J. Am. Chem. Soc., 2004, 126, 14702 CrossRef CAS;
(c) A. Nagaki, M. Togai, S. Suga, N. Aoki, K. Mae and J. Yoshida, J. Am. Chem. Soc., 2005, 127, 11666 CrossRef CAS;
(d) T. Kawaguchi, H. Miyata, K. Ataka, K. Mae and J. Yoshida, Angew. Chem., Int. Ed., 2005, 44, 2413 CrossRef CAS;
(e) P. He, P. Watts, F. Marken and S. J. Haswell, Angew. Chem., Int. Ed., 2006, 45, 4146 CrossRef CAS;
(f) K. Tanaka, S. Motomatsu, K. Koyama, S. Tanaka and K. Fukase, Org. Lett., 2007, 9, 299 CrossRef CAS;
(g) H. R. Sahoo, J. G. Kralj and K. F. Jensen, Angew. Chem., Int. Ed., 2007, 46, 5704 CrossRef CAS;
(h) C. H. Hornung, M. R. Mackley, I. R. Baxendale and S. V. Ley, Org. Process Res. Dev., 2007, 11, 399 CrossRef CAS;
(i) T. Fukuyama, M. Kobayashi, M. T. Rahman, N. Kamata and I. Ryu, Org. Lett., 2008, 10, 533 CrossRef CAS;
(j) A. Nagaki, E. Takizawa and J. Yoshida, J. Am. Chem. Soc., 2009, 131, 1654 CrossRef CAS;
(k) A. Nagaki, E. Takizawa and J. Yoshida, Chem.–Eur. J., 2010, 16, 14149 CrossRef CAS.
-
(a)
J. Yoshida, Flash Chemistry: Fast Organic Synthesis in Microsystems, Wiley-Blackwell, 2008 Search PubMed;
(b) J. Yoshida, Chem. Commun., 2005, 4509 RSC;
(c) J. Yoshida, A. Nagaki and T. Yamada, Chem.–Eur. J., 2008, 14, 7450 CrossRef CAS;
(d) J. Yoshida, Chem. Rec., 2010, 10, 332 CrossRef CAS.
-
(a) A. Nagaki, Y. Tomida, H. Usutani, H. Kim, N. Takabayashi, T. Nokami, H. Okamoto and J. Yoshida, Chem.–Asian J., 2007, 2, 1513 CrossRef CAS;
(b) H. Usutani, Y. Tomida, A. Nagaki, H. Okamoto, T. Nokami and J. Yoshida, J. Am. Chem. Soc., 2007, 129, 3046 CrossRef CAS;
(c) A. Nagaki, N. Takabayashi, Y. Tomida and J. Yoshida, Org. Lett., 2008, 18, 3937 CrossRef;
(d) A. Nagaki, H. Kim and J. Yoshida, Angew. Chem., Int. Ed., 2008, 47, 7833 CrossRef CAS;
(e) A. Nagaki, N. Takabayashi, Y. Tomida and J. Yoshida, Beilstein J. Org. Chem., 2009, 5, No16 CrossRef;
(f) Y. Tomida, A. Nagaki and J. Yoshida, Org. Lett., 2009, 11, 3614 CrossRef CAS;
(g) A. Nagaki, H. Kim and J. Yoshida, Angew. Chem., Int. Ed., 2009, 48, 8063 CrossRef CAS;
(h) A. Nagaki, H. Kim, C. Matsuo and J. Yoshida, Org. Biomol. Chem., 2010, 8, 1212 RSC;
(i) A. Nagaki, H. Kim, Y. Moriwaki, C. Matsuo and J. Yoshida, Chem.–Eur. J., 2010, 16, 11167 CrossRef CAS;
(j) Y. Tomida, A. Nagaki and J. Yoshida, J. Am. Chem. Soc., 2011, 133, 3744 CrossRef CAS;
(k) H. Kim, A. Nagaki and J. Yoshida, Nat. Commun., 2011, 2, 264 CrossRef;
(l) T. Asai, A. Takata, Y. Ushiogi, Y. Iinuma, A. Nagaki and J. Yoshida, Chem. Lett., 2011, 40, 393 CrossRef CAS;
(m) A. Nagaki, S. Yamada, M. Doi, Y. Tomida, N. Takabayashi and J. Yoshida, Green Chem., 2011, 13, 1110 RSC.
-
(a) H. Wakami and J. Yoshida, Org. Process Res. Dev., 2005, 9, 787 CrossRef CAS;
(b) T. Iwasaki, N. Kawano and J. Yoshida, Org. Process Res. Dev., 2006, 10, 1126 CrossRef CAS;
(c) Y. Ushiogi, T. Hase, Y. Iinuma, A. Takata and J. Yoshida, Chem. Commun., 2007, 2947 RSC.
- H. Kagechika and K. Shudo, J. Med. Chem., 2005, 48, 5875 CrossRef CAS.
-
(a) T. Yamakawa, H. Kagechika, E. Kawachi, Y. Hashimoto and K. Shudo, J. Med. Chem., 1990, 33, 1430 CrossRef CAS;
(b) N. A. Rizvi, J. L. Marshall, E. Ness, M. J. Hawkins, C. Kessler, H. Jacobs, W. D. Brenckman, Jr., J. S. Lee, W. Petros, W. K. Hong and J. M. Kurie, J. Clin. Oncol., 2002, 20, 3522 CrossRef CAS.
- Y. Yamanoi and H. Nishihara, J. Org. Chem., 2008, 73, 6671 CrossRef CAS.
- L. S. Chen, G. J. Chen and C. Tamborski, J. Organomet. Chem., 1981, 215, 281 CrossRef CAS.
Footnote |
† Electronic Supplementary Information (ESI) available: Experimental section. See DOI: 10.1039/c1ra00377a/ |
|
This journal is © The Royal Society of Chemistry 2011 |
Click here to see how this site uses Cookies. View our privacy policy here.