DOI:
10.1039/C1PY00294E
(Paper)
Polym. Chem., 2011,
2, 2257-2260
Tribenzotriquinacene-based polymers of intrinsic microporosity†
Received
28th June 2011
, Accepted 17th July 2011
First published on 11th August 2011
Abstract
Bowl-shaped tribenzotriquinacene (TBTQ) derivatives containing hydroxyl groups at their peripheral sites are successful monomers for the synthesis of polymers of intrinsic microporosity (PIMs) with examples of both network and non-network PIMs demonstrated. Nitrogen adsorption measurements reveal apparent surface areas in the range of 560–670 m2 g−1 for the TBTQ-PIMs. A network structure and i-propyl, rather than methyl substitution at the apical site of the TBTQ unit, appear to favour porosity.
Introduction
Microporous materials are solids that contain interconnected pores of molecular dimensions (i.e. <2 nm).1 Examples include zeolites, activated carbons and metal–organic frameworks (MOFs).2 They have high internal surface areas that can be exploited for a wide range of applications, including gas storage, gas separation and heterogeneous catalysis. Over recent years there has been increasing interest in the synthesis of polymeric microporous materials built from purely organic components such as covalent organic frameworks,3,4 hypercrosslinked polymers5 and conjugated microporous polymers.6 One approach to microporous organic materials involves the synthesis of polymers of intrinsic microporosity (PIMs) that possess rigid and contorted macromolecular structures which cannot fill space efficiently, leaving molecular-sized interconnected voids.7,8 The rigidity is enforced by the polymer being composed of fused rings and the contorted structure arises from the incorporation of non-linear ‘sites of contortion’ such as a spiro-centres9,10 or triptycene units.11 PIMs demonstrate great diversity of structure due to the variety of monomers that can be used for their synthesis. In addition, they can be prepared as either insoluble network polymers with high surface areas of interest as hydrogen storage materials,12 adsorbents,13 heterogeneous catalysts14 or as soluble non-network polymers whose solvent processability can be exploited for the fabrication of sensors,15,16 or highly permeable gas separation membranes.17,18
One of the structural components used previously for making PIMs is cyclotricatechylene (CTC; Fig. 1a).19 The resulting network polymer from the reaction between CTC and 2,3,5,6-tetrafluoroterephthalonitrile demonstrated an apparent BET surface area of 830 m2 g−1 with the microporosity presumably arising from the bowl-shape of the CTC units.20 Due to the potential for providing pre-formed concave binding sites of a fixed size and shape for specific adsorption, we are interested in making further examples of PIMs with similar bowl-shaped components. Hence, our attention was drawn to the tribenzotriquinacene (TBTQ) unit designed and prepared previously by Kuck and co-workers,21 which has found utility as concave binding moieties for fullerenes22,23 and for crystal engineering.24 Despite Kuck's anticipation that these CTC analogues would be a useful building block for organic networks,21 to date, no TBTQ-based polymers have been prepared. In the context of PIMs, it was anticipated that the fused-ring structure of the TBTQ unit should ensure greater polymer rigidity as compared to CTC and that the substitution of small alkyl groups (e.g.methyl or i-propyl) at the apical carbon would hinder columnar stacking of the bowl-shaped units in the solid state and enhance microporosity. In addition, the synthesis of a suitable tetrahydroxylated derivative of CTC that could act as a precursor to soluble non-network PIMs appears a very difficult challenge due to its one-step synthesis,19 whereas, Kuck's step-wise assembly of TBTQ should allow access to suitable non-symmetrical derivatives.21,25 Here we describe the synthesis of symmetrical and non-symmetrical TBTQ monomers 1–4, containing peripheral hydroxyl substituents, and their subsequent polymerisation with 2,3,5,6-tetrafluoroterephthalonitrile to give TBTQ-PIMs1–4 (Fig. 1b&c).
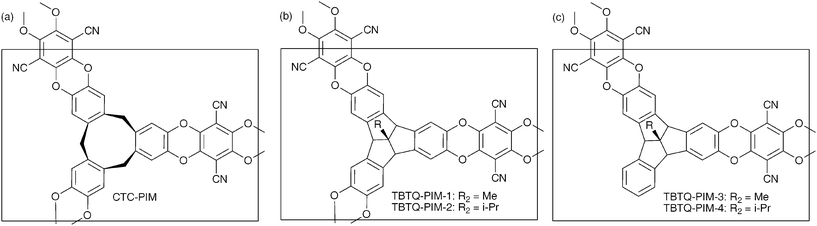 |
| Fig. 1 The structures of (a) the CTC-PIM network, (b) the proposed TBTQ network polymers and (c) the proposed TBTQ non-network polymers. | |
Experimental
Experimental details for the synthesis of precursors 1–22 and TBTQ-PIMs1–4 are given in the supplemental information along with solid state NMR spectra and nitrogen isotherms.†
Results and discussion
1. Synthesis
The original synthesis of 2,3,6,7,10,11-tetramethoxy-12d-methyltribenzotriquinacene used by Kuck et al.25 was modified so as to reduce the number of synthetic steps, to allow for the simple introduction of apical substituents and to provide non-symmetrical derivatives (Scheme 1). The key intermediates for the synthesis of monomers 1–4 are the diones 5 and 6, which are prepared conveniently by a one-step reaction between commercially available veratrole and methylmalonic acid or i-propylmalonic acid, respectively, in polyphosphoric acid (PPA). This direct method is higher yielding than the four-step preparation of dione 5 used by Kuck and co-workers25 and could be readily adapted to introduce other apical substituents to the TBTQ unit. The benzophenones 7 and 8, prepared by the acylation of veratrole by veratroic acid or benzoic acid in PPA using the procedure published by Kulkarni et al.,26 are reduced with sodium borohydride to give the required benzhydrol intermediates 9 and 10. The moderate yield of 9 is due to its tendency to disproportionate to form bis(3,4-dimethoxyphenyl)methane and 3,3′,4,4′-tetramethoxy-benzophenone.25 The synthesis of the TBTQ monomers 1–4 proceeds by the coupling of dione 5 with alcohols 9 or 10 to obtain the intermediates 11 and 13, or with dione 6 with alcohols 9 or 10 to give 12 and 14, respectively. Reductions of 11–14 with di-i-butylaluminium hydride (DIBAL-H) yields the alcohols 15–18 in good yields which can then be subjected to two-fold cyclodehydration with Eaton′s reagent to give the desired TBTQ precursors 19–22 which are readily demethylated with BBr3 to give the target monomers 1–4.
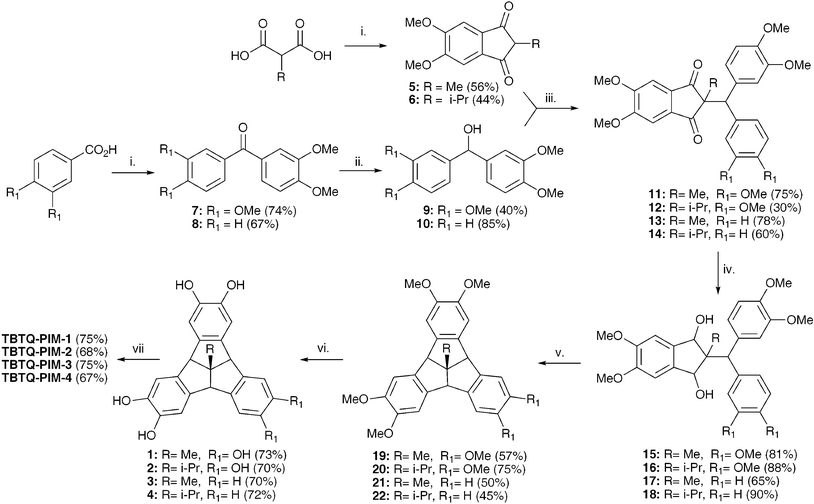 |
| Scheme 1
Reagents and conditions. i. veratrole, polyphosphoric acid, 80 °C, 12 h. ii. NaBH4, DCM, 4 h. iii. TsOH, toluene/dichloroethane, reflux 2h. iv. DIBAL-H, DCM, 12 h. v. Eatons Reagent, 4 h. vi. BBr3, DCM, 12 h. vii. Tetrafluoroterephthalonitrile, K2CO3, DMF, 65–80 °C, 24–96 h. | |
Polymerisation of monomers 1 and 2 with commercially available 2,3,5,6 tetrafluoroterephthalonitrile, by a nucleophilic aromatic substitution reaction, gives TBTQ-PIM-1 and TBTQ-PIM-2 in high yields. Similarly, monomers 3 and 4 provide TBTQ-PIM-3 and TBTQ-PIM-4, which were anticipated to possess non-network structures and hence to be soluble in organic solvents. However, all resulting TBTQ polymers proved insoluble in all solvents tested, which precluded molecular mass characterisation by solution-based techniques such as Gel Permeation Chromatography. However, characterisation was achieved using solid state 13C NMR, which gave spectra consistent with the ideal structures of the polymers (e.g.Fig. 2).
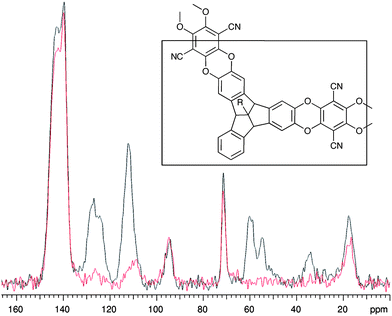 |
| Fig. 2 Solid state 13C NMR spectra for TBTQ-PIM-4 (R = i-Pr). The black spectrum is the full cross-polarisation spectrum and the red spectrum is with interrupted decoupling, which removes signals from carbons attached to single hydrogens (see ESI† for assignments and spectra for the other polymers). | |
2. Microporosity
Nitrogen adsorption measurements at 77 K of powdered samples of the TBTQ polymers confirm their microporosity by demonstrating significant adsorption at low relative pressures (See ESI† for isotherms). The apparent BET surface areas and pore volumes of the polymers calculated from these data are shown in Table 1 along with that of the related CTC-PIM.20 The TBTQ network polymers TBTQ-PIM-1 and TBTQ-PIM-2 demonstrate greater microporosity than the respective non-network TBTQ polymers TBTQ-PIM-3 and TBTQ-PIM-4 containing the same apical substituent on the TBTQ component (i.e. R = Me or i-Pr), although all TBTQ polymers fail to match the microporosity of the CTC-PIM network. Interestingly, the polymers with i-propyl apical substituents on the TBTQ unit demonstrate higher microporosity than the corresponding polymers with methyl substituents suggesting that the larger group helps reduce stacking of the bowl-shaped units within the solid state.
Polymer
|
BET surface area (m2 g−1) |
Pore volumea (mL g−1) |
Calculated from amount of nitrogen adsorbed at 77 K and relative pressure p/po = 0.98.
|
CTC-PIM
20 |
830 |
0.39 |
TBTQ-PIM-1
|
565 |
0.36 |
TBTQ-PIM-2
|
673 |
0.41 |
TBTQ-PIM-3
|
511 |
0.37 |
TBTQ-PIM-4
|
568 |
0.37 |
The key factors that determine the degree of intrinsic microporosity with a polymer are rigidity and inability to pack space efficiently. Although it was assumed that the fused-ring structure of TBTQ possesses greater rigidity, which was anticipated to enhance microporosity, it is clear that the CTC unit once embedded within a polymer network is sufficiently rigid to support microporosity and hence no advantage was gained from using TBTQ units. In general, the effect of adding substituents onto a rigid PIM framework is difficult to predict as the new groups may serve to decrease packing efficiency and thus enhance microporosity or they may simply fill space created by the framework and therefore reduce microporosity. By comparison to the CTC-Network-PIM,20 it seems reasonable to conclude that the additional fused ring structure at the centre of the TBTQ unit fills some of the space created by the rigid polymer framework. In addition, single crystal X-ray diffraction data shows that the TBTQ unit is less concave relative to CTC as demonstrated by the greater angle of 113° between the neighbouring benzene rings as compared27 to analogous angle of 107° within CTC (Fig. 3).28 This too might help to explain the reduced microporosity of the TBTQ-based PIMs relative to that of the CTC-based polymer.
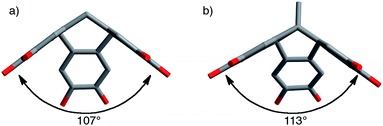 |
| Fig. 3 Representations of the CTC28 and TBTQ25 units showing the greater concavity of CTC as demonstrated by the angle between neighbouring phenyl rings measured from single crystals XRD data. | |
Conclusions
The TBTQ unit has been incorporated successfully into polymers and these new materials have demonstrated significant microporosity. Unfortunately, the anticipated benefits of the TBTQ unit over the more easily prepared CTC unit did not materialise. Although the fused-ring structure of TBTQ is more rigid than CTC, it is clear that the conformational rigidity of CTC is sufficient to support microporosity once it is incorporated into a network polymer. Any additional frustration of packing efficiency that might arise from the apical substitution of the TBTQ component does not appear to compensate for the loss of free volume associated with either the space-filling properties of the central carbon and its apical substituent and/or the shallower concavity of the TBTQ units as compared to that of CTC. However, the original thesis that appropriate apical substitution might enhance microporosity is partially supported by the greater apparent surface areas observed for TBTQ-PIM-2 and 4 that contain the bulkier i-propyl apical group. Finally, although the use of TBTQ did allow the synthesis of non-network polymers TBTQ-PIM-3 and 4, the anticipated benefits of solvent processability were not realised. These results contribute to the growing number of structure–property studies of microporous polymers that will inform future designs of these interesting materials.7 Indeed, further elaboration of the structure of the TBTQ by increasing the bulk or rigidity of the apical substituents or by placing substituents on the peripheral sites, using the synthetic methodologies developed in this study, may yet enhance both microporosity and processability of polymers containing bowl-shaped components.
Acknowledgements
We acknowledge funding from EPSRC grants EP/G01244X (MC) and EP/H024034/1 (CGB) and thank the EPSRC National Solid State NMR Research Service (Durham University).
Notes and references
- J. Rouquerol, D. Avnir, C. W. Fairbridge, D. H. Everett, J. H. Haynes, N. Pernicone, J. D. F. Ramsay, K. S. W. Sing and K. K. Unger, Pure Appl. Chem., 1994, 66, 1739–1758 CrossRef CAS.
-
P. A. Wright, Microporous Framework Solids, RSC, Cambridge, 2007 Search PubMed.
- A. P. Cote, A. I. Benin, N. W. Ockwig, M. O'Keeffe, A. J. Matzger and O. M. Yaghi, Science, 2005, 310, 1166–1170 CrossRef CAS.
- H. M. El-Kaderi, J. R. Hunt, J. L. Mendoza-Cortes, A. P. Cote, R. E. Taylor, M. O'Keeffe and O. M. Yaghi, Science, 2007, 316, 268–272 CrossRef CAS.
- M. P. Tsyurupa and V. A. Davankov, React. Funct. Polym., 2002, 53, 193–203 CrossRef CAS.
- A. I. Cooper, Adv. Mater., 2009, 21, 1291–1295 CrossRef CAS.
- N. B. McKeown and P. M. Budd, Macromolecules, 2010, 43, 5163–5176 CrossRef CAS.
- N. B. McKeown and P. M. Budd, Chem. Soc. Rev., 2006, 35, 675–683 RSC.
- P. M. Budd, B. S. Ghanem, S. Makhseed, N. B. McKeown, K. J. Msayib and C. E. Tattershall, Chem. Commun., 2004, 230–231 RSC.
- M. Carta, K. J. Msayib, P. M. Budd and N. B. McKeown, Org. Lett., 2008, 10, 2641–2643 CrossRef CAS.
- B. S. Ghanem, M. Hashem, K. D. M. Harris, K. J. Msayib, M. C. Xu, P. M. Budd, N. Chaukura, D. Book, S. Tedds, A. Walton and N. B. McKeown, Macromolecules, 2010, 43, 5287–5294 CrossRef CAS.
- N. B. McKeown, P. M. Budd and D. Book, Macromol. Rapid Commun., 2007, 28, 995–1002 CrossRef CAS.
- A. V. Maffei, P. M. Budd and N. B. McKeown, Langmuir, 2006, 22, 4225–4229 CrossRef CAS.
- H. J. Mackintosh, P. M. Budd and N. B. McKeown, J. Mater. Chem., 2008, 18, 573–578 RSC.
- Y. Wang, N. B. McKeown, K. J. Msayib, G. A. Turnbull and I. D. W. Samuel, Sensors, 2011, 11, 2478–2487 CrossRef CAS.
- N. A. Rakow, M. S. Wendland, J. E. Trend, R. J. Poirier, D. M. Paolucci, S. P. Maki, C. S. Lyons and M. J. Swierczek, Langmuir, 2010, 26, 3767–3770 CrossRef CAS.
- P. M. Budd and N. B. McKeown, Polym. Chem., 2010, 1, 63–68 RSC.
- P. M. Budd, N. B. McKeown, B. S. Ghanem, K. J. Msayib, D. Fritsch, L. Starannikova, N. Belov, O. Sanfirova, Y. P. Yampol'skii and V. Shantarovich, J. Membr. Sci., 2008, 325, 851–860 CrossRef CAS.
- M. J. Hardie, Chem. Soc. Rev., 2010, 39, 516–527 RSC.
- N. B. McKeown, B. Gahnem, K. J. Msayib, P. M. Budd, C. E. Tattershall, K. Mahmood, S. Tan, D. Book, H. W. Langmi and A. Walton, Angew. Chem., Int. Ed., 2006, 45, 1804–1807 CrossRef CAS.
- J. Tellenbroker and D. Kuck, Angew. Chem., Int. Ed., 1999, 38, 919–922 CrossRef CAS.
- B. Bredenkotter, S. Henne and D. Volkmer, Chem.–Eur. J., 2007, 13, 9931–9938 CrossRef CAS.
- P. E. Georghiou, L. N. Dawe, H. A. Tran, J. Strube, B. Neumann, H. G. Stammler and D. Kuck, J. Org. Chem., 2008, 73, 9040–9047 CrossRef CAS.
- J. Strube, B. Neumann, H. G. Stammler and D. Kuck, Chem.–Eur. J., 2009, 15, 2256–2260 CrossRef.
- M. Harig, B. Neumann, H.-G. Stammler and D. Kuck, Eur. J. Org. Chem., 2004, 2381–2397 CrossRef CAS.
- A. B. Diwadkar, H. D. Shroff and A. B. Kulkarni, Curr. Sci., 1962, 31, 149–150 CAS.
- M. Harig and D. Kuck, Eur. J. Org. Chem., 2006, 1647–1655 CrossRef CAS.
- J. A. Hyatt, E. N. Duesler, D. Y. Curtin and I. C. Paul, J. Org. Chem., 1980, 45, 5074–5079 CrossRef CAS.
|
This journal is © The Royal Society of Chemistry 2011 |