DOI:
10.1039/C1PY00162K
(Review Article)
Polym. Chem., 2011,
2, 2146-2154
Recognition of polymer side chains by cyclodextrins
Received
12th April 2011
, Accepted 11th May 2011
First published on 7th June 2011
Abstract
This review article describes the interaction of cyclodextrins (CDs) with polymer side chains as model systems for biological molecular recognition, focusing on the steric effect of the polymer chains, the effect of the conformation of the polymer main chain or competition with association of polymer side chains, and the effect of multi-site interaction. Some typical examples of stimuli-responsive systems, nanoparticles for drug delivery systems and macroscopic self-assembly based on the interaction of CDs with polymer side chains are also reviewed briefly.
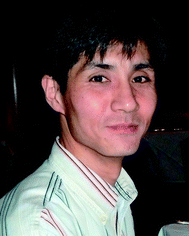 Akihito Hashidzume | Akihito Hashidzume is an associate professor at the Graduate School of Science, Osaka University. He completed his Doctorate of Science from Osaka University in 1997 under the supervision of Prof. Mikiharu Kamachi. After receiving his PhD, he began working at the Graduate School of Science, Osaka University, as an assistant professor. During his academic career, he worked as a visiting scholar with Prof. Steven C. Zimmerman at the University of Illinois at Urbana-Champaign. He has received the Society of Cyclodextrins, Japan Award for Young Scientists in 2008. His research interests include polymer synthesis, water-soluble polymers, and polymer assemblies. |
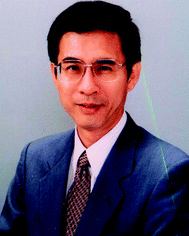 Akira Harada | Akira Harada is a professor at the Graduate School of Science at Osaka University. He earned his academic degrees from Osaka University and has spent most of his career working for the same university. During his career, Dr Harada worked for IBM Research as a visiting scientist and as a Post Doc at Colorado State University. After working at the Institute of Scientific and Industrial Research (ISIR) at Osaka University, he assumed his current position as a professor. Harada's work and research interests include supramolecular science, synthetic polymer chemistry, and biomacromolecules (e.g., antibodies and DNA). He has received many awards, including the IBM Science Award, Osaka Science Award, Japan Polymer Society Award, Cyclodextrin Society Award, a Medal with Purple Ribbon from the Japanese government, and the International Izatt-Chistensen Award in Macrocyclic Chemistry. |
1. Introduction
Biological systems maintain their living activities based on a number of chemical reactions, which proceed selectively in aqueous media under mild conditions, using macromolecules (i.e., nucleic acids, proteins, and polysaccharides).1–3 The chemical reactions in biological systems start with the formation of well-defined supramolecular structures through recognition of macromolecular and low molecular weight species by the biological macromolecules. Molecular recognition in biological systems is specific like “lock and key”. The specific molecular recognition in biological systems is realized by non-covalent bonds of side chains (e.g., nucleosides in nucleic acids and substituents of amino acid residues in proteins), which are arranged three-dimensionally by the main chain with well-defined conformations. To elucidate essences of molecular recognition responsible for selective chemical reactions in biological systems, we have been studying the interaction of cyclodextrins (CDs) with side chains of water soluble polymers as model systems.
CDs are cyclic oligomers of D-(+)-glucopyranose units linked through an α-1,4-glycoside bond. CDs of 6, 7, and 8 glucopyranose units are called α-CD, β-CD, and γ-CD, respectively. CDs are toroidal in shape with narrower primary hydroxyl and wider secondary hydroxyl sides. Since the most important features of CDs are their hydrophobic exterior and hydrophobic cavity, CDs include selectively hydrophobic compounds of size and shape matching their cavity to form inclusion complexes in aqueous media. On the basis of this phenomenon, CDs are considered as simple biomimetic compounds.4–8 Furthermore, since CDs are nontoxic, they are widely used as additives for foods, cosmetics, and personal care items, and their pharmaceutical applications have been also studied.9–15
Before the 1990s, there were only a few papers on the interaction of CDs with side chains of water soluble polymers in aqueous media.16–18 However, since our first paper19 on the molecular recognition of alkyl side chains bound to a poly(acrylamide) by CDs was published in 1997, an increasing number of research groups began to focus on the interaction of CDs with side chains of water soluble polymers. Recent years have brought considerable interest in studying the interaction of CDs with side chains of water soluble polymers from the viewpoint of environmentally benign applications based on aqueous systems. Most of the research on the interaction of CDs with water soluble polymers has been focusing on the control of solution properties of hydrophobically modified water soluble polymers.20–35 To the best of our knowledge, however, research on the interaction of CDs with water soluble polymers as model systems for biological molecular recognition is scarce apart from our research.
This review deals with the interaction of CDs with polymer side chains as model systems for biological molecular recognition, focusing on the steric effect of the polymer chains, the effect of the conformation of the polymer main chain or competition with association of polymer side chains, and the effect of multi-site interaction. Some typical examples on utilization of recognition of polymer side chains by CDs are also reviewed briefly. Interested readers can refer to other comprehensive reviews on utilization of the interaction of CDs with side chains of water soluble polymers.36–42
2. The interaction of cyclodextrins with polymer side chains as model systems for biological molecular recognition
2.1. The steric effect of polymer chains
The simplest effect of attachment of guest moieties onto the polymer main chain is the steric effect of the polymer main and side chains. Since the polymer chains are considered as very large substituents, which may restrict the direction of access of CDs and cause a difference in the structure of inclusion complexes, the steric effect of polymer chains may perturb the formation of inclusion complexes to enhance the selectivity in the interaction of CDs with guest moieties. This subsection reviews a few examples of the selectivity in the interaction of CDs with side chains enhanced by the steric effect of polymer chains.
2.1.1. The interaction of cyclodextrins with poly(acrylamide)s modified with alkyl groups.
The interaction of CDs with poly(acrylamide)s modified with several alkyl groups (i.e., n-butyl, sec-butyl, tert-butyl, hexyl, isooctyl, and dodecyl) (Scheme 1) was studied by 1H NMR.191H NMR spectra exhibited downfield shifts of signals due to the alkyl side chains in the presence of CDs, indicative of the interaction of CDs with polymer side chains. Using the peak shift as a function of the CD concentration, apparent association constants (K) were determined for all the pairs of CDs and alkyl side chains, as listed in Table 1. These K values demonstrate that all α-CD, β-CD, and γ-CD interact most strongly with dodecyl side chains among all the alkyl groups examined because of the hydrophobicity of the dodecyl side chain. The K values in Table 1 also indicate that α-CD interacts favorably with linear alkyl side chains, β-CD interacts preferably with branched alkyl side chains, and γ-CD interacts only weakly with all the alkyl side chains examined. It is noteworthy that α-CD interacted with n-butyl side chains (K = 55 M−1) but not with tert-butyl side chains, whereas β-CD interacted with tert-butyl side chains (K = 340 M−1) but not with n-butyl side chains. On the other hand, α-CD interacts with both the corresponding model compounds, i.e., n-butyl alcohol and tert-butyl alcohol (K = 20 and 6 M−1, respectively). These observations indicate that CDs can interact more selectively with certain alkyl side chains attached to a poly(acrylamide) backbone than with low molecular weight model compounds because of the steric effect of the polymer main chain.
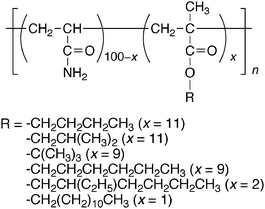 |
| Scheme 1 Chemical structure of poly(acrylamide)s modified with alkyl groups. | |
Table 1 Apparent association constants (K) of the interaction of CDs with alkyl side chains attached to the poly(acrylamide) backbone
Alkyl side chain |
K/M−1 |
α-CD
|
β-CD
|
γ-CD
|
It was not possible to determine the K value because of no significant peak shifts.
|
n-Butyl
|
55 |
—a |
—a |
i-Butyl
|
36 |
—a |
—a |
t-Butyl
|
—a |
340 |
57 |
n-Hexyl
|
290 |
110 |
—a |
Isooctyl
|
203 |
294 |
51 |
Dodecyl
|
990 |
660 |
245 |
2.1.2. The interaction of cyclodextrins with poly[(meth)acrylamides] possessing amino acid residues.
The interaction of CDs with poly[(meth)acrylamides] possessing several amino acid residues (pMXaa and pAXaa, Scheme 2) was investigated by 1H NMR and two-dimensional nuclear Overhauser effect spectroscopy (2D NOESY).43,441H NMR spectra for 1 mM CD were recorded in the presence of varying concentrations of pMXaa and pAXaa. In the cases of pMXaa polymers (i.e., pMPhe and pMTrp), the signals ascribable to CDs did not show any significant shifts. In the case of pMTrp, however, the signals due to CDs were very broad in the presence of the polymer. Whereas the signals did not exhibit any significant broadening even in the presence of pMPhe. For the systems of pMTrp and CDs, the excess halfwidths of signals due to C1 protons in CD were plotted against the monomer unit concentration to roughly estimate apparent K values, as listed in Table 2. It is likely that the apparent K values for the systems of pMPhe and CDs were considerably smaller than 10 M−1. In the case of some pAXaa polymers, on the other hand, signals due to CDs exhibited significant shifts. The differences between chemical shifts for signals due to the C1 and C3 protons in CDs were plotted against the monomer unit concentrations to roughly estimate the apparent K values, as listed in Table 3. In the table, “—” means that it was not possible to estimate the apparent K values because of very small shifts or no saturation tendency, indicative of no or very weak interaction. Comparing the data for the pMXaa polymers with those for the pAXaa polymers indicates that the α-methyl group has significant effects on the interaction of CDs. In the case of the Phe polymers, α-methyl groups made the interaction weaker. In the case of the Trp polymers, changes in 1H NMR spectra upon interaction were contrasting. It is concluded that α-methyl groups can be important in macromolecular recognition.
![Chemical structure of poly[(meth)acrylamides] possessing amino acid residues.](/image/article/2011/PY/c1py00162k/c1py00162k-s2.gif) |
| Scheme 2 Chemical structure of poly[(meth)acrylamides] possessing amino acid residues. | |
Table 2 Apparent association constants (K) for the interaction of CDs with pMTrp and pMPhe
Polymer code |
K/M−1 |
α-CD
|
β-CD
|
γ-CD
|
It was not possible to determine the K value because of small excess halfwidths.
|
pMTrp |
30 ± 2 |
83 ± 3 |
11 ± 2 |
pMPhe |
—a |
—a |
—a |
Table 3 Apparent association constants (K) for the interaction of CDs with pAXaa
Polymer code |
K/M−1 |
α-CD
|
β-CD
|
γ-CD
|
It was not possible to estimate the K value because of small shifts.
It was not possible to estimate the K value because no saturation tendency was observed.
|
pAVal |
—a |
—a |
—a |
pAIle |
—b |
—b |
—a |
pAPhe |
8 ± 1 |
—a |
—a |
pATyr |
(1.9 ± 0.7) × 101 |
—b |
—a |
pATrp |
—b |
(2.3 ± 0.6) × 102 |
(2.0 ± 0.1) × 101 |
For the model compounds, i.e., sodium salts of the corresponding amino acids, 1H NMR spectra for 1 mM CD were also obtained in the presence of varying concentrations of the model compounds. The differences between the chemical shifts for the C1 and C3 protons in CD were plotted against the concentration of the model compounds to determine K values, as listed in Table 4.
Table 4 Association constants (K) for the interaction of CDs with the model compounds
Model compound |
K/M−1 |
α-CD
|
β-CD
|
γ-CD
|
It was not possible to estimate the K value because no saturation tendency was observed.
It was not possible to estimate the K value because of the formation of higher-order complexes.
|
Val
|
—a |
7 ± 4 |
—b |
Ile
|
(2.60 ± 0.08) × 102 |
9 ± 1 |
—b |
Phe |
(1.9 ± 0.2) × 101 |
(8.8 ± 0.4) × 101 |
3 ± 2 |
Tyr |
(5 ± 2) × 101 |
(1.40 ± 0.05) × 102 |
(2.8 ± 0.8) × 101 |
Trp |
(1.5 ± 0.1) × 101 |
(8.9 ± 0.9) × 101 |
(1.6 ± 0.3) × 101 |
The K values for the pAXaa polymers were compared with those for the model compounds to elucidate the effect of the attachment of amino acid residues onto the polymer chains, although it should be noted that the K values for the polymers are apparent ones because amino acid residues are extremely localized on the polymer chains in the interaction of CDs with the polymers. On the basis of the K values in Tables 3 and 4, the amino acid residues can be divided into three categories based on the molar volume: category 1 contains Val and Ile (90.8–107.5 cm3 mol−1), category 2 Phe and Tyr (121.2–123.1 cm3 mol−1), and category 3 Trp (143.9 cm3 mol−1).45 The interaction of CDs with Val or Ile residues was not detected when they were bound to the polymer chains. When Phe or Tyr residues were bound to the polymer chains, the interaction became significantly weaker and showed a slightly enhanced selectivity toward α-CD, compared to the interaction with the corresponding model compound. When Trp residues were attached to the polymer chains, the interaction did not change significantly and exhibited a slightly increased selectivity toward β-CD and γ-CD. These observations indicate that when amino acid residues of a smaller molar volume are bound to the polymer chains, the interaction of CDs becomes weaker compared to the interaction with the corresponding model compound.
When guest moieties are bound to the polymer chains, the effects of the polymer chains on the interaction of CDs with the guest moieties may include the steric effect of the polymer main chain and neighboring side chains, the effect of localized binding sites, and noncovalent bonds of CDs with neighboring side chains. The steric effect may hinder the interaction, whereas the effect of localized binding sites and the noncovalent bonds may assist the interaction. It is likely that the pAXaa polymers used in this study take almost the same conformation, independent of the amino acid residues.46,47 Thus, the effect of localized binding sites and the steric effect of the polymer main chain may be practically the same for all the pAXaa polymers and the steric effect of neighboring side chains may correspond to the molar volume of amino acid residues. Since the interactions were reduced for most of the systems examined, the dominant effect of the polymer chains may be the steric hindrance in the CDs/pAXaa systems.
2.1.3. The interaction of cyclodextrins with human and bovine serum albumins.
The interactions of CDs with proteins have been investigated from the viewpoint of pharmaceutical applications, including drug delivery and control of denaturation, renaturation, and aggregation of proteins.9,48–61 To the best of our knowledge, however, fundamental studies on the interactions of CDs with proteins have been scarce presumably because of very weak interaction of CDs with proteins.
The interaction of CDs with human and bovine serum albumins (HSA and BSA, respectively) was investigated by steady state fluorescence, circular dichroism, and 2D NOESY spectroscopies and isothermal titration calorimetry.62 For any systems of CDs/HSA and CDs/BSA, steady state fluorescence and circular dichroism spectra and isothermal titration calorimetric data showed no significant interaction. However, 2D NOESY data exhibited correlation signals between signals ascribable to CDs and aromatic amino acid residues for the α-CD/HSA and β-CD/HSA systems, whereas no significant correlation signals were observed for the γ-CD/HSA, α-CD/BSA, β-CD/BSA, or γ-CD/BSA system. These data indicate that α-CD and β-CD interact with Phe and/or Tyr residues exposed on the surface of HSA and discriminate between HSA and BSA. Since HSA and BSA show ca. 76% sequence homology, there are only small differences between neighboring residues around Phe and Tyr residues exposed on their surfaces. Because Phe and Tyr residues have middle molar volumes (i.e., category 2 in the former subsection), the interaction of CDs with these residues may be perturbed significantly by the environments.
2.2. The effect of the conformation of the polymer main chain or competition with association of polymer side chains
Hydrophobically modified water soluble polymers form various types of aggregates in aqueous media, depending on their chemical structures.63 At higher hydrophobe contents, intrapolymer hydrophobic associations may be dominant to form polymer micelles in which the polymer main chains take highly folded conformations.46,64,65 In the interaction of CDs with this kind of polymer micelles, the formation of inclusion complexes of CDs with hydrophobic side chains may compete with hydrophobic associations of side chains, resulting in interaction different from that of CDs with water soluble polymers modified with a small amount of hydrophobes.
The interaction of CDs with polymer micelles formed from an alternating copolymer of sodium maleate and dodecyl vinyl ether (Mal/C12, Scheme 3) was studied by 1H NMR and 2D NOESY.66,67 It has been reported that Mal/C12 forms flower-like micelles or flower necklaces, depending on the molecular weight.65 In the case of polyacrylamides modified with a small amount of dodecyl groups, all α-CD, β-CD, and γ-CD include dodecyl side chains.19 In the case of Mal/C12, however, only α-CD includes dodecyl groups but not β-CD or γ-CD. These observations are indicative of an enhanced selectivity toward α-CD. It is noteworthy that the interaction of α-CD with polymer micelles of Mal/C12 was cooperative and the cooperativity increased with increasing the molecular weight of Mal/C12. To understand details of the cooperative interaction, the binding isotherms for the interaction of α-CD with Mal/C12 were analyzed using a model proposed based on the one-dimensional lattice theory. On the basis of the analysis, it was concluded that the dominant factor for the cooperative interaction was the molecular weight dependent hydrophobic interaction of dodecyl side chains. The cooperative interaction is explained as shown in Fig. 1. In the absence of α-CD, Mal/C12 forms flower-like micelles or flower necklaces. (In the figure, a flower-like micelle, in which most of the dodecyl groups are omitted, is drawn for simplicity.) At lower concentrations, α-CD does not interact with dodecyl groups because they form hydrophobic domains. When the α-CD concentration is beyond a certain level, α-CD commences to interact with dodecyl side chains. Once the α-CD commences to interact with dodecyl groups to form inclusion complexes, the polymer main chain is unfolded because of the steric hindrance in inclusion complexes, and neighboring dodecyl groups become more accessible, resulting in the cooperativity.
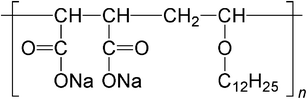 |
| Scheme 3 Chemical structure of Mal/C12. | |
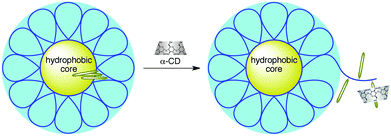 |
| Fig. 1 Conceptual illustration of the interaction of α-CD with Mal/C12. | |
The interaction of β-CD with alternating copolymers of sodium maleate and vinyl ethers carrying an adamantyl group (i.e., 1-adamantyl vinyl ether and 4-(1-adamantyl)phenyl vinyl ether) (Mal/Ad and Mal/AdPh, Scheme 4) was also investigated mainly by 1H and 2D NMR.68 The complexation of β-CD with the corresponding model compounds, i.e., sodium 1-adamantanecarboxylate and sodium 4-(1-adamantyl)benzoate, was also examined by isothermal titration calorimetry. Calorimetric data indicated that β-CD included sodium 1-adamantanecarboxylate and sodium 4-(1-adamantyl)benzoate with K values of 3.1 × 104 and 7.8 × 105 M−1, respectively, indicating that β-CD interacts more favorably with the adamantylphenyl group than with the adamantyl group. 1H NMR spectra of Mal/Ad and Mal/AdPh indicated downfield shifted signals due to adamantyl groups in the presence of β-CD. Using peak shifts of 1H NMR, the apparent K values were roughly estimated to be 6.6 × 102 and 2.3 × 102 M−1 for adamantyl and adamantylphenyl side chains, respectively, indicating that β-CD includes more preferably adamantyl side chains than adamantylphenyl side chains. These observations can be considered as inversion of the selectivity of β-CD. To understand the mechanism for the inversion of selectivity, the structures of inclusion complexes were characterized by 2D NMR (i.e., ROESY and NOESY) for the model and polymer systems. In the inclusion complex of β-CD with the model compound the β-CD included deeply the adamantylphenyl moiety, and the phenylene ring and the adamantyl group are close to the primary and secondary hydroxyl sides, respectively (Fig. 2a). In the polymer system, on the other hand, β-CD included adamantylphenyl side chains from both the primary and secondary hydroxyl sides (Fig. 2b and c). Since the adamantylphenyl group is more hydrophobic, these groups associate strongly by hydrophobic interaction. The hydrophobic interaction of the adamantylphenyl group competes with the inclusion complex formation, and thus, the competition may destabilize the stable inclusion mode (Fig. 2b), resulting in the inversion of the selectivity of β-CD.
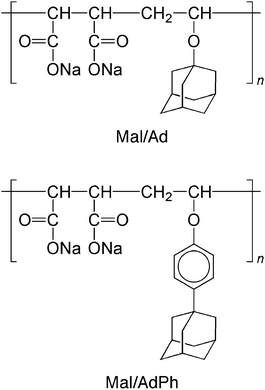 |
| Scheme 4 Chemical structures of Mal/Ad and Mal/AdPh. | |
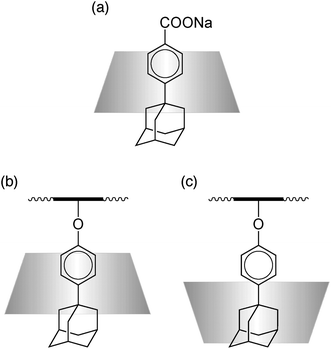 |
| Fig. 2 Conceptual illustration of the interaction of α-CD with sodium 4-(1-adamantyl)benzoate and Mal/AdPh. | |
2.3. The effect of multi-site interaction
Another important point of attaching interaction sites (i.e., CD and guest moieties) to polymer chains is that polymers may interact with each other through simultaneous formation of plural inclusion complexes between CD and guest moieties. In polymer–polymer systems, the interaction at multi-sites may enhance the selectivity.
The interaction of a poly(acrylamide) modified with β-CD moieties with poly(acrylamide)s possessing naphthyl groups (1-naphthylmethyl and 2-naphthylmethyl groups) was investigated by viscometry (Scheme 5).69 Native β-CD interacted with polymer-carrying 1-naphthylmethyl and 2-naphthylmethyl groups with apparent K values of (0.70–1.4) × 102 and (1.6–2.3) × 102 M−1, respectively, indicative of a slight selectivity of β-CD toward the 2-naphthylmethyl group.70 In the case of polymer–polymer interactions, both the mixtures were Newtonian liquids. The zero-shear viscosities of mixtures of the 1-naphthylmethyl polymer and the β-CD polymer were practically the same as those for mixtures of an unmodified poly(acrylamide) and the β-CD polymer, whereas the zero-shear viscosities of mixtures of the 2-naphthylmethyl polymer and the β-CD polymer were much higher than those of mixtures of the 1-naphthylmethyl polymer and the β-CD polymer. These observations indicate that the hydrodynamic size of aggregates of the 2-naphthylmethyl polymer was markedly larger than that of aggregates of the 1-naphthylmethyl polymer (Fig. 3). It can be considered as an enhanced selectivity of the β-CD polymer toward the 2-naphthylmethyl polymer through multi-site interaction.
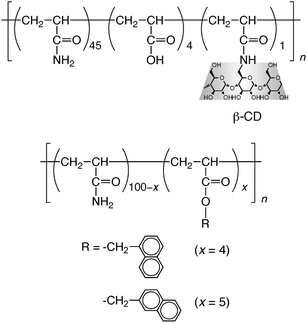 |
| Scheme 5 Chemical structures of poly(acrylamide)s possessing β-CD, 1-naphthylmethyl, and 2-naphthyl moieties. | |
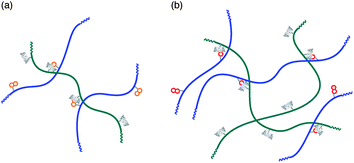 |
| Fig. 3 Conceptual illustration of interpolymer aggregates of a poly(acrylamide) carrying β-CD moieties and poly(acrylamide)s modified with naphthyl groups: 1-naphthylmethyl (a) and 2-naphthylmethyl (b). | |
The molecular imprinting technique using CD monomers as functional monomers is useful to obtain polymers carrying a number of CD moieties with some three-dimensional arrangement.71 It was reported that molecularly imprinted polymers prepared using angiotensin derivatives as the template discriminated small differences in the angiotensin derivatives presumably based on multi-site interaction of β-CD moieties with the hydrophobic amino acid residues, which are somehow arranged three-dimensionally by the main chain.72
3. Utilization of recognition of polymer side chains by cyclodextrins
Since various types of aggregates formed from hydrophobically modified water soluble polymers act as emulsifiers, dispersants, surfactants, associative thickeners, and rheology modifiers, these polymers are extensively used in various applications, including printing, paints, coatings, cosmetics, personal care goods, and drug delivery systems, along with increasing interests in water-borne systems because of recent environmental issues.73–75 As described in the Introduction, on the other hand, CDs have been widely investigated because of their unique molecular recognition ability and nontoxicity.4–9,12–15 Combination of CDs and hydrophobically modified water soluble polymers may allow one to construct smart materials. This section briefly reviews utilization of the interaction of CDs with polymer side chains of water soluble polymers, focusing on stimuli-responsive systems and nanoparticles for drug delivery systems.
3.1. Stimuli-responsive systems
Living bodies are stimuli-responsive supramolecular assemblies of hierarchical structures based on biological macromolecules.1–3 These biological stimuli-responsive supramolecular assemblies have inspired a number of research groups to devote their efforts to construction of artificial stimuli-responsive supramolecular assemblies, aiming at supramolecular motors and machines.76,77 Stimuli, applicable to stimuli-responsive supramolecular assemblies, include temperature, force (pressure), pH, chemical species, light, and redox. Among them, chemical, light, and redox stimuli are often used to control the molecular recognition of CDs. Since chemical species, which CDs include strongly, act as competitive guests, combination with these species provides chemical-responsive molecular recognition systems. Azobenzene and stilbene undergo trans-to-cis and cis-to-transphotoisomerization under irradiation with UV and visible light, respectively. α-CD includes their trans-isomers but not their cis-isomers, indicative of photo-responsive molecular recognition. β-CD and metalocenes are known as a representative pair of redox-responsive molecular recognition: β-CD includes metalocenes in their neutral and reduced states but not in their cationic and oxidized states.
Side chain poly(-pseudo-)rotaxanes are one of the simplest artificial supramolecular assemblies based on polymers. A series of photo-responsive poly(-pseudo-)rotaxanes using azobenzene moieties have been reported. Side chain poly-pseudo-rotaxanes were prepared from α-CD and poly(acrylic acid)s modified with the azobenzene moiety through hexamethylene and dodecamethylene linkers. After trans-to-cisphotoisomerization of the azobenzene moiety, a side chain poly-pseudo-rotaxane was dissociated for the hexamethylene linker, whereas a side chain polyrotaxane with unidirectional inclusion was obtained for the dodecamethylene linker.78Azobenzene moieties were attached to an alternating copolymer of maleic acid and 9-decanoic acid through an oligo(ethylene glycol) linker. After the formation of a side chain poly-pseudo-rotaxane by adding α-CD, trans-to-cisphotoisomerization yielded a side chain polyrotaxane, in which α-CD included the heptamethylene moiety in the 9-decanoic acid unit. β-CD was further added to the polyrotaxane to obtain a side chain hetero-poly-pseudo-rotaxane, in which β-CD included the cis-azobenzene moiety.79 A photo-responsive side chain polyrotaxane was synthesized by coupling reaction of a poly(acrylic acid) with a graft chain, consisting of heptamethylene and azobenzene moieties linked with an oligo(ethylene glycol) linker and an adamantyl stopper, in the presence of α-CD. In the side chain polyrotaxane, α-CD included the azobenzene moiety in the trans state whereas it included the heptamethylene moiety in the cis state.80
There have been a number of reports on stimuli-responsive solution properties of mixtures of CDs with hydrophobically modified water soluble polymers. Since it is known that β-CD includes strongly adamantane moieties, adamantane derivatives are often used as chemical stimuli for CD-based supramolecular assemblies. Nanogels formed from a pullulan modified with cholesteryl side chains were dissociated in the presence of β-CD because cholesteryl side chains were masked by β-CD. When 1-adamantanecarboxylic acid was added as a chemical stimulant to the binary mixture of β-CD with the cholesteryl modified pullulan, β-CD included the competitive guest more favorably, resulting in re-formation of nanogel.20 A chemical-responsive hydrogel containing single walled carbon nanotubes was prepared from single-walled carbon nanotubes solubilized with a pyrene-carrying β-CD derivative and a dodecyl modified poly(acrylic acid). When sodium 1-adamantanecarboxylate or α-CD was added as a chemical stimulant to the hydrogel, the mixture becomes sol because of dissociation of inclusion complexes of β-CD moieties with dodecyl side chains.81 Some examples of photo-responsive aggregation behavior utilizing the combination of α-CD and azobenzene moieties have been reported. Hydroxypropyl methylcellulose modified with azobenzene moieties exhibited photoregulated thermoresponsive sol–gel transition based on the lower critical temperature-type phase transition. Since the trans-azobenzene moiety is more hydrophobic, the critical temperature for the trans form is lower than that for the cis form. In the presence of α-CD, on the other hand, the CD formed inclusion complexes with the trans-azobenzene moieties, and thus the trans form exhibited a higher critical temperature.26–28 A ternary mixture of α-CD, a dodecyl modified poly(acrylic acid), and 4,4′-azodibenzoic acid exhibited photo-responsive gel-to-sol and sol-to-gel transitions. In the case of the trans form, α-CD included preferably azodicarboxylic acid, and thus the dodecyl modified poly(acrylic acid) formed the hydrogel through interpolymer association of dodecyl side chains. In the case of the cis form, on the other hand, α-CD masked dodecyl side chains, and thus the mixture turned sol.82 Photo-responsive systems based on polymer–polymer interaction have been also reported. A mixture of an azobenzene-modified poly(acrylamide) and a poly(allylamine)-based β-CD polymer formed a supramolecular gel in the trans state, whereas the mixture was sol in the cis state.83 Mixtures of poly(acrylic acid)s modified with α-CD moieties and a poly(acrylic acid) modified with the azobenzene moiety through a dodecamethylene linker showed photo-responsive viscosity changes, depending on the substituted position on the α-CD moiety.84 Recently, a mixture of a poly(acrylic acid) modified with the azobenzene moiety through a dodecamethylene linker and a curdlan possessing a number of α-CD moieties also showed photo-responsive gel-to-sol and sol-to-gel transitions.85 The curdlan in the mixture included carbon nanotubes to form the photo-responsive hydrogel containing single-walled carbon nanotubes.86 Examples of redox-responsive systems are fewer, compared with those of photo-responsive systems. A ternary mixture of β-CD, a dodecyl modified poly(acrylic acid), and ferrocenecarboxylic acid formed a redox-responsive hydrogel. In the reduced state, β-CD included ferrocenecarboxylic acid, and thus the dodecyl modified poly(acrylic acid) formed a hydrogel. In the oxidized state, on the other hand, β-CD masked dodecyl side chains and the mixture turned sol.87
CD polymers and guest polymers form polymer aggregates through the formation of inclusion complexes. Under optimized conditions, these polymer aggregates are nanoparticles of a narrower size distribution. Since a considerable content of CD moieties in the nanoparticles are usually unoccupied, these unoccupied CD moieties include and release slowly hydrophobic drugs. Thus, these nanoparticles, which are promising for drug delivery systems, have been investigated by several research groups.40,88–90
4. Macroscopic self-assembly through molecular recognition
Macroscopic observation of molecular recognition has been realized using the interaction between poly(acrylamide)-based CD and guest gels through multi-site interaction.91,92Poly(acrylamide) gels possessing CDs and several guests (i.e., adamantyl, n-butyl, tert-butyl, n-hexyl, cyclohexyl, n-dodecyl, and cyclododecyl side chains) were prepared by conventional radical copolymerization (Scheme 6). The gels were cut into pieces of millimetre or centimetre scale with a knife and dyed using conventional hydrophilic dyes, which do not interact with CDs. When pieces of β-CD-gel and adamantyl-gel were mixed and shaken in water, β-CD-gel and adamantyl-gel stuck with each other to form an assembly of alternating or checkered structure. When a gel assembly formed from a piece of β-CD-gel and a piece of adamantyl-gel was pulled from both sides, one of the gel pieces was broken without damaging the contact interface, indicative of strong interaction of gels. In the presence of an excess of β-CD or adamantanamine hydrochloride, the β-CD-gel and adamantyl-gel did not adhere, because the competitive host and guest masked guest and host moieties, respectively. These competitive experiments confirmed that the formation of gel assemblies was based on molecular recognition. When pieces of α-, β- or γ-CD-gel, gel possessing linear side chains (i.e., n-butyl, n-hexyl, or n-dodecyl side chains) and gel possessing branched or cyclic side chains (i.e., tert-butyl, cyclohexyl, or cyclododecyl side chains), were shaken in water for several minutes, α-CD-gel formed gel assemblies only with gel possessing linear side chains, and β- or γ-CD-gel only with gel possessing branched or cyclic side chains (Fig. 4). These observations indicate that the gels discriminate precisely their corresponding counterparts.
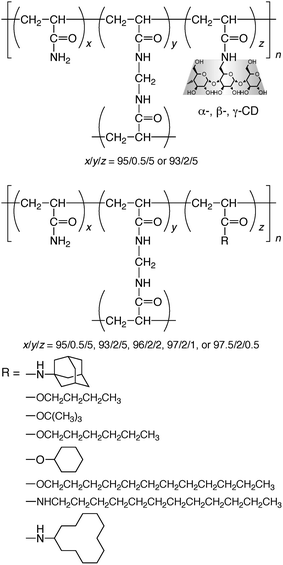 |
| Scheme 6 Chemical structures of poly(acrylamide)-based gels possessing α-CD, β-CD, γ-CD, and guest moieties. | |
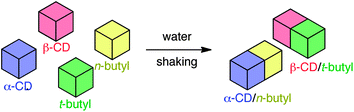 |
| Fig. 4 Conceptual illustration of the formation of gel assemblies. | |
5. Conclusion
This article reviewed briefly studies on the interaction of CDs with polymer side chains as model systems for biological molecular recognition and their utilization. As described in the Introduction, molecular recognition by biological macromolecules (i.e., macromolecular recognition) is important for chemical reactions in biological systems. Since biological chemical reactions proceed highly selectively in aqueous media under mild conditions, it becomes more important to understand in depth biological macromolecular recognition and to use widely the essences of biological macromolecular recognition.
Acknowledgements
The authors would like to express their acknowledgement to Associate Professor Hiroyasu Yamaguchi and Assistant Professor Yoshinori Takashima, Department of Macromolecular Science, Graduate School of Science, Osaka University, University, for fruitful discussion and suggestions.
References
-
D. Voet and J. G. Voet, Biochemistry, Wiley & Sons, New York, 2004 Search PubMed.
-
J. M. Berg, J. L. Tymoczko and L. Stryer, Biochemistry, W.H. Freeman, New York, 2007 Search PubMed.
-
B. Alberts, A. Johnson, J. Lewis, M. Raff, K. Roberts and P. Walter, Molecular Biology of the Cell, Garland Publishing Inc., New York, 2008 Search PubMed.
-
M. L. Bender and M. Komiyama, Cyclodextrin Chemistry, Springer, Berlin, Germany, 1978 Search PubMed.
-
J. Szejtli, Cyclodextrins and Their Inclusion Complexes, Akadémiai Kiadó, Budapest, Hungury, 1982 Search PubMed.
-
Cyclodextrins, ed. J. Szejtli and T. Osa, Pergamon, Oxford, U.K., 1996 Search PubMed.
-
A. Harada, in Large Ring Molecules, ed. J. A. Semlyen, Wiley & Sons, Chichester, U.K., 1996, pp. 407–432 Search PubMed.
-
Cyclodextrins and Their Complexes: Chemistry, Analytical Methods, Applications, ed. H. Dodziuk, Wiley-VCH, Weinheim, 2006 Search PubMed.
- K. Uekama, F. Hirayama and T. Irie, Chem. Rev. (Washington, DC, U. S.), 1998, 98, 2045–2076 CrossRef CAS.
-
K. Uekama, F. Hirayama and H. Arima, in Cyclodextrins and Their Complexes, ed. H. Dodziuk, Wiley-VCH, Weinheim, 2006, pp. 381–422 Search PubMed.
-
H. Hashimoto, in Cyclodextrins and Their Complexes, ed. H. Dodziuk, Wiley-VCH, Weinheim, 2006, pp. 452–459 Search PubMed.
- J. Szejtli and L. Szente, Eur. J. Pharm. Biopharm., 2005, 61, 115–125 Search PubMed.
- M. E. Brewster and T. Loftsson, Adv. Drug Delivery Rev., 2007, 59, 645–666 CrossRef CAS.
- G. Astray, C. Gonzalez-Barreiro, J. C. Mejuto, R. Rial-Otero and J. Simal-Gandara, Food Hydrocolloids, 2009, 23, 1631–1640 CrossRef CAS.
- T. Loftsson and M. E. Brewster, J. Pharm. Pharmacol., 2010, 62, 1607–1621 Search PubMed.
- T. Iijima, T. Uemura, S. Tsuzuku and J. Komiyama, J. Polym. Sci., Polym. Phys. Ed., 1978, 16, 793–802 Search PubMed.
- T. Uemura, T. Moro, J. Komiyama and T. Iijima, Macromolecules, 1979, 12, 737–739 Search PubMed.
- T. Moro, S. Kobayashi, K. Kainuma, T. Uemura, T. Harada, J. Komiyama and T. Iijima, Carbohydr. Res., 1979, 75, 345–348 Search PubMed.
- A. Harada, H. Adachi, Y. Kawaguchi and M. Kamachi, Macromolecules, 1997, 30, 5181–5182 CrossRef CAS.
- K. Akiyoshi, Y. Sasaki, K. Kuroda and J. Sunamoto, Chem. Lett., 1998, 27, 93–94 CrossRef.
- M. F. Islam, R. D. Jenkins, D. R. Bassett, W. Lau and H. D. Ou-Yang, Macromolecules, 2000, 33, 2480–2485 CrossRef CAS.
- H. Ritter, O. Sadowski and E. Tepper, Angew. Chem., Int. Ed., 2003, 42, 3171–3173 CrossRef CAS.
- O. Kretschmann, S. W. Choi, M. Miyauchi, I. Tomatsu, A. Harada and H. Ritter, Angew. Chem., Int. Ed., 2006, 45, 4361–4365 CrossRef.
- O. Kretschmann, C. Steffens and H. Ritter, Angew. Chem., Int. Ed., 2007, 46, 2708–2711 CrossRef CAS.
- C. Koopmans and H. Ritter, J. Am. Chem. Soc., 2007, 129, 3502–3503 CrossRef CAS.
- P. Zheng, X. Hu, X. Zhao, L. Li, K. C. Tam and L. H. Gan, Macromol. Rapid Commun., 2004, 25, 678–682 CrossRef CAS.
- X. Hu, P. J. Zheng, X. Y. Zhao, L. Li, K. C. Tam and L. H. Gan, Polymer, 2004, 45, 6219–6225 CrossRef CAS.
- P. J. Zheng, C. Wang, X. Hu, K. C. Tam and L. Li, Macromolecules, 2005, 38, 2859–2864 CrossRef CAS.
- C. Galant, A.-L. Kjøniksen, G. T. M. Nguyen, K. D. Knudsen and B. Nyström, J. Phys. Chem. B, 2006, 110, 190–195 CrossRef CAS.
- N. Beheshti, H. Bu, K. Zhu, A.-L. Kojniksen, K. D. Knudsen, R. Pamies, J. G. H. Cifre, J. G. de la Torre and B. Nyström, J. Phys. Chem. B, 2006, 110, 6601–6608 CrossRef CAS.
- X. Guo, A. A. Abdala, B. L. May, S. F. Lincoln, S. A. Khan and R. K. Prud'homme, Macromolecules, 2005, 38, 3037–3040 CrossRef CAS.
- X. Guo, A. A. Abdala, B. L. May, S. F. Lincoln, S. A. Khan and R. K. Prud'homme, Polymer, 2006, 47, 2976–2983 Search PubMed.
- L. Li, X. Guo, L. Fu, R. K. Prud'homme and S. F. Lincoln, Langmuir, 2008, 24, 8290–8296 CrossRef CAS.
- L. Li, X. Guo, J. Wang, P. Liu, R. K. Prud'homme, B. L. May and S. F. Lincoln, Macromolecules, 2008, 41, 8677–8681 CrossRef CAS.
- X. Guo, J. Wang, L. Li, D.-T. Pham, P. Clements, S. F. Lincoln, B. L. May, Q. Chen, L. Zheng and R. K. Prud'homme, Macromol. Rapid Commun., 2010, 31, 300–304 Search PubMed.
- G. Wenz, B.-H. Han and A. Müller, Chem. Rev. (Washington, DC, U. S.), 2006, 106, 782–817 CrossRef CAS.
- A. Hashidzume, I. Tomatsu and A. Harada, Polymer, 2006, 47, 6011–6027 CrossRef CAS.
- A. Harada, A. Hashidzume, H. Yamaguchi and Y. Takashima, Chem. Rev. (Washington, DC, U. S.), 2009, 109, 5974–6023 CrossRef CAS.
- A. Harada and A. Hashidzume, Aust. J. Chem., 2010, 63, 599–610 Search PubMed.
- J. Zhou and H. Ritter, Polym. Chem., 2010, 1, 1552–1559 RSC.
- F. Yuen and K. C. Tam, Soft Matter, 2010, 6, 4613–4630 RSC.
- G. Chen and M. Jiang, Chem. Soc. Rev., 2011, 40, 2254–2266 RSC.
- A. Hashidzume and A. Harada, Polymer, 2006, 47, 3448–3454 Search PubMed.
- W. Oi, A. Hashidzume and A. Harada, Polymer, 2011, 52, 746–751 Search PubMed.
- A. A. Zamyatnin, Annu. Rev. Biophys. Bioeng., 1984, 13, 145–165 Search PubMed.
- T. Kawata, A. Hashidzume and T. Sato, Macromolecules, 2007, 40, 1174–1180 Search PubMed.
- A. Hashidzume, T. Kawata, A. Tanaka, Y. Takabayashi and T. Sato, J. Chromatogr., A, 2010, 1217, 2990–2992 Search PubMed.
- A. Cooper, J. Am. Chem. Soc., 1992, 114, 9208–9209 CrossRef CAS.
- D. Rozema and S. H. Gellman, J. Am. Chem. Soc., 1995, 117, 2373–2374 CrossRef CAS.
- D. Rozema and S. H. Gellman, Biochemistry, 1996, 35, 15760–15771 CrossRef CAS.
- T. Irie and K. Uekama, Adv. Drug Delivery Rev., 1999, 36, 101–123 CrossRef CAS.
- L. Sharma and A. Sharma, Eur. J. Biochem., 2001, 268, 2456–2463 Search PubMed.
- D. E. Otzen, B. R. Knudsen, F. Aachmann, K. L. Larsen and R. Wimmer, Protein Sci., 2002, 11, 1779–1787 Search PubMed.
- F. L. Aachmann, D. E. Otzen, K. L. Larsen and R. Wimmer, Protein Eng., 2003, 16, 905–912 Search PubMed.
- A. Desai, C. Lee, L. Sharma and A. Sharma, Biochimie, 2006, 88, 1435–1445 Search PubMed.
- S. Tavornvipas, F. Hirayama, S. Takeda, H. Arima and K. Uekama, J. Pharm. Sci., 2006, 95, 2722–2729 Search PubMed.
- M. Toda, H. Itoh, Y. Kondo and F. Hamada, Bioorg. Med. Chem., 2007, 15, 1983–1988 Search PubMed.
- L. Matilainen, K. L. Larsen, R. Wimmer, P. Keski-Rahkonen, S. Auriola, T. Järvinen and P. Jarho, J. Pharm. Sci., 2008, 97, 2720–2729 Search PubMed.
- E. Bajorunaite, A. Cirkovas, K. Radzevicius, K. L. Larsen, J. Sereikaite and V.-A. Bumelis, Int. J. Biol. Macromol., 2009, 44, 428–434 Search PubMed.
- T. Serno, J. F. Carpenter, T. W. Randolph and G. Winter, J. Pharm. Sci., 2010, 99, 1193–1206 Search PubMed.
- H. S. Samra, F. He, A. Bhambhani, J. Pipkin, R. Zimmerer, S. B. Joshi and C. R. Middaugh, J. Pharm. Sci., 2010, 99, 2800–2818 Search PubMed.
- W. Oi, M. Isobe, A. Hashidzume and A. Harada, Macromol. Rapid Commun., 2011, 32, 501–505 Search PubMed.
-
A. Hashidzume, Y. Morishima and K. Szczubialka, in Handbook of Polyelectrolytes and Their Applications, ed. S. K. Tripathy, J. Kumar and H. S. Nalwa, American Scientific Publishers, Stevenson Ranch, CA, 2002, pp. 1–63 Search PubMed.
- Y. Tominaga, M. Mizuse, A. Hashidzume, Y. Morishima and T. Sato, J. Phys. Chem. B, 2010, 114, 11403–11408 Search PubMed.
- M. Ueda, A. Hashidzume and T. Sato, Macromolecules, 2011, 44, 2970–2977 Search PubMed.
- D. Taura, A. Hashidzume and A. Harada, Macromol. Rapid Commun., 2007, 28, 2306–2310 CrossRef CAS.
- D. Taura, A. Hashidzume, Y. Okumura and A. Harada, Macromolecules, 2008, 41, 3640–3645 Search PubMed.
- D. Taura, Y. Taniguchi, A. Hashidzume and A. Harada, Macromol. Rapid Commun., 2009, 30, 1741–1744 CrossRef CAS.
- A. Hashidzume, F. Ito, I. Tomatsu and A. Harada, Macromol. Rapid Commun., 2005, 26, 1151–1154 CrossRef CAS.
- A. Harada, F. Ito, I. Tomatsu, K. Shimoda, A. Hashidzume, Y. Takashima, H. Yamaguchi and S. Kamitori, J. Photochem. Photobiol., A, 2006, 179, 13–19 Search PubMed.
-
M. Komiyama, T. Takeuchi, T. Mukawa and H. Asanuma, Molecular Imprinting: From Fundamentals to Applications, Wiely-VCH, Weinheim, 2003 Search PubMed.
- S.-h. Song, K. Shirasaka, M. Katayama, S. Nagaoka, S. Yoshihara, T. Osawa, J. Sumaoka, H. Asanuma and M. Komiyama, Macromolecules, 2007, 40, 3530–3532 CrossRef CAS.
-
Hydrophilic Polymers. Performance with Environmental Acceptability, Advances in Chemistry Series 248, ed. J. E. Glass, American Chemical Society, Washington, DC, 1996 Search PubMed.
-
Associative Polymers in Aqueous Solutions, ACS Symposium Series 765, ed. J. E. Glass, American Chemical Society, Washington, DC, 2000 Search PubMed.
-
Stimuli-Responsive Water Soluble and Amphiphilic Polymers, ACS Symposium Series 780, ed. C. L. McCormick, American Chemical Society, Washington, DC, 2001 Search PubMed.
-
Molecular Machines, Topics in Current Chemistry 262, ed. T. R. Kelly, Springer, Heidelberg, Germany, 2005 Search PubMed.
-
V. Balzani, A. Credi and M. Venturi, Molecular Devices and Machines: Concepts and Perspectives for the Nanoworld, Wiley-VCH, Weinheim, 2008 Search PubMed.
- I. Tomatsu, A. Hashidzume and A. Harada, Angew. Chem., Int. Ed., 2006, 45, 4605–4608 CrossRef CAS.
- D. Taura, S. Li, A. Hashidzume and A. Harada, Macromolecules, 2010, 43, 1706–1713 CrossRef CAS.
- J. Hu, A. Hashidzume and A. Harada, Macromol. Chem. Phys., 2011, 212, 1032–1038 Search PubMed.
- T. Ogoshi, Y. Takashima, H. Yamaguchi and A. Harada, J. Am. Chem. Soc., 2007, 129, 4878–4879 CrossRef CAS.
- I. Tomatsu, A. Hashidzume and A. Harada, Macromolecules, 2005, 38, 5223–5227 CrossRef CAS.
- Y. Takashima, T. Nakayama, M. Miyauchi, Y. Kawaguchi, H. Yamaguchi and A. Harada, Chem. Lett., 2004, 33, 890–891 CrossRef CAS.
- I. Tomatsu, A. Hashidzume and A. Harada, J. Am. Chem. Soc., 2006, 128, 2226–2227 CrossRef CAS.
- S. Tamesue, Y. Takashima, H. Yamaguchi, S. Shinkai and A. Harada, Angew. Chem., Int. Ed., 2010, 49, 7461–7464 CrossRef CAS.
- S. Tamesue, Y. Takashima, H. Yamaguchi, S. Shinkai and A. Harada, Eur. J. Org. Chem., 2011, 2011, 2801–2806 Search PubMed.
- I. Tomatsu, A. Hashidzume and A. Harada, Macromol. Rapid Commun., 2006, 27, 238–241 CrossRef CAS.
- R. Gref, C. Amiel, K. Molinard, S. Daoud-Mahammed, B. Sebille, B. Gillet, J.-C. Beloeil, C. Ringard, V. Rosilio, J. Poupaert and P. Couvreur, J. Controlled Release, 2006, 111, 316–324 Search PubMed.
- S. Daoud-Mahammed, C. Ringard-Lefebvre, N. Razzouq, V. Rosilio, B. Gillet, P. Couvreur, C. Amiel and R. Gref, J. Colloid Interface Sci., 2007, 307, 83–93 CrossRef CAS.
- S. Daoud-Mahammed, J. L. Grossiord, T. Bergua, C. Amiel, P. Couvreur and R. Gref, J. Biomed. Mater. Res., Part A, 2008, 86, 736–748 Search PubMed.
- A. Harada, R. Kobayashi, Y. Takashima, A. Hashidzume and H. Yamaguchi, Nat. Chem., 2011, 3, 34–37 CrossRef CAS.
- H. Yamaguchi, R. Kobayashi, Y. Takashima, A. Hashidzume and A. Harada, Macromolecules, 2011, 44, 2395–2399 Search PubMed.
|
This journal is © The Royal Society of Chemistry 2011 |