DOI:
10.1039/C1PY00008J
(Paper)
Polym. Chem., 2011,
2, 1085-1090
High-quality and excellent green-light-emitting poly(acenaphthylene) film: electrosynthesis and characterization
Received
8th January 2011
, Accepted 28th February 2011
First published on 15th March 2011
Abstract
Conducting poly(acenaphthylene) (PAcN) films were synthesized electrochemically by direct anodic oxidation of acenaphthylene (AcN) in boron trifluoride diethyl etherate (BFEE) containing additional 20% anhydrous ethyl ether (by volume). The oxidation potential onset of AcN in this medium was measured to be 0.92 V, which was much lower than that determined in CH3CN containing 0.1 mol L−1 tetra butylammonium tetrafluoroborate (Bu4NBF4, 1.34 V). PAcN films obtained from BFEE + 20% EE showed good electrochemical behavior and good thermal stability with an electrical conductivity of 0.43 S cm−1. The average molecular weight with a polydispersity index of 1.06 was determined by gel permeation chromatography to be Mw = 426,000 g mol−1 for the electrochemically synthesized polymer. The structure and morphology of the polymer were investigated by ultraviolet-visible spectroscopy, infrared spectroscopy, nuclear molecular resonance spectroscopy and scanning electron microscopy, respectively. Fluorescent spectra indicated that the electrochemically polymerized PAcN was an excellent green light-emitting material (fluorescence quantum yield as high as 0.3). All these results indicate that the striking PAcN films obtained have potential applications in various fields.
Introduction
High-quality conducting polymer (CP) films, such as polyaniline, polypyrrole, polythiophene, and their derivatives have received extensive attention for more than thirty years because of their potential applications in energy storage system, sensors, electrochromic display devices, antistatic coating, biosensors, etc.1–5 Different approaches are used to deposit CP films onto substrates. These preparations include spin-coating,6 ink jet printing,7dip-coating, drop-coating,8thermal evaporation,9 Langmuir–Blodgett self-assembly techniques10 and electrochemical deposition. Among these methods, electrochemical polymerization has been proven to be an especially useful method.11–14
Xu's group has developed many novel conducting polymers (CPs) from fused ring compounds in ionic liquid media by electrochemical polymerization.15–17 For instance, polybenzanthrone was electrodeposited in boron trifluoride diethyl etherate (BFEE) or in acetonitrile solution containing Bu4NBF4.16 Polyselenophene18 and poly(5-methoxyindole)19 obtained from BFEE were proved to be high-quality free-standing CPs. Zhuang and Chen20 obtained the high-quality polycarbazole films electropolymerized in two ionic liquids, 1-butyl-3-methylimidazolium hexafluorophosphate and N-butyl-N-methyl pyrrolidinium bis((trifluoromethyl)sulfonyl) imide, respectively; however, the qualities of polycarbazole films electrodeposited in other volatile organic solvents were usually very poor. The electrolyte and solvent can greatly influence the morphology, electrochemical performance and other properties of CP films.21,22 As an excellent solvent and electrolyte, BFEE has been proved to be the appropriate electrolyte to polymerize the fused aromatic compounds, such as phenanthrene, pyrene, fluoranthene, benzanthrone and their derivatives.16,23–25 The Lewis acidity of BFEE lowers the electropolymerization potential of many monomers, usually resulting in high-quality and stable polymer films as previous reported.
Many CPs show excellent fluorescent properties, and can be used as good candidates for the development of electronic and electrochemical devices such as photovoltaic devices, electroluminescence, organic light-emitting diodes, and sensors. Poly(5-methoxyindole) is a good blue-light emitter.19 Polybenzanthrone is a green light-emitting polymer with fluorescence quantum yields as high as 0.52.16 Conjugated oligopyrene films electropolymerized in a mixed electrolyte of BFEE and diethyl ether exhibit visible blue luminescence with high fluorescence quantum yield, and the films are used as naked-eye fluorescent sensors for nitroaromatic compound vapours.26 Polyacenaphthene is reported as a blue-light emitter.13
Polyacenaphthylene (PAcN) is known as a ‘light-harvesting’ or ‘antenna’ polymer containing the acenaphthylchromophore.27 The rate and efficiency of energy migration in polymers containing an acenaphthylene (AcN) chromophore, such as PAcNhomopolymer and its copolymers with maleic anhydride, were investigated by Smith and Ghiggino.28 The reported PAcN showed a fluorescence emission in the blue band. The polymers containing acenaphthyl energy donors and a terminal anthryl energy acceptor exhibited excitation energy transfer efficiency up to 70%.27 Like other light harvestingpolymers, PAcN is usually synthesized by conventional free radical polymerisation but with uncontrollable molecular weight and a broad molecular weight distribution.29 Therefore, other polymerization techniques have been investigated. Thermal polymerization is found to be a good method to obtain high molecular weight PAcN, and may yield molecular weights of 1,000,00030 ∼ 2,000,000.31 However, there have been no reports on the electropolymerization of AcN in un-aqueous media, as far as we know. Therefore, the electrosysthesis of high-quality PAcN films from the direct anodic oxidation of AcN has become a great challenge.
In this paper, a high-quality and excellent green-light-emitting PAcN film was successfully electrodeposited by direct anodic oxidation of AcN both in the BFEE medium containing 20% (by volume) ethyl ether (EE). The electrochemical behavior, thermal stability, electrical conductivity, fluorescence properties and morphology of the as-prepared PAcN films were investigated in detail.
Results and discussion
Electrochemical synthesis of PAcN films
Because the solubility of AcN monomer is poor in pure BFEE, 20% EE was added into BFEE (by volume) as the mixed electrolyte medium for the electrochemical polymerization of AcN. The anodic polarization curves of 0.01 mol L−1 AcN were tested both in the mixed electrolyte mentioned above and in the CH3CN medium containing 0.1 mol L−1Bu4NBF4. The results are shown in Fig. 1. The oxidation onset potential of AcN is initiated at 0.92 V in BFEE + 20% EE [Fig. 1, (B)], which is much lower than that of AcN in CH3CN + 0.1 mol L−1Bu4NBF4 (1.34 V) [Fig. 1, (A)]. This is mainly due to BFEE electrolyte as a middle Lewis acid, which can increase the ionic conductivity of the electrolyte. Generally, the lower oxidation potential of the monomer the more decreased the possibility of side reactions during the polymerization process. Based on these aforementioned considerations, BFEE + 20% EE was chosen as the best electrosynthesis of PAcN films.
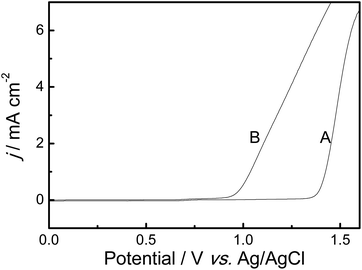 |
| Fig. 1 Anodic polarization curves of 0.01 mmol L−1 AcN in mixed electrolytes of BFEE containing 20% EE (B) (by volume), and in CH3CN + 0.1 mol L−1Bu4NBF4 (A). Potential scan rates, 50 mV s−1. | |
The successive cyclic voltammograms (CVs) of 0.01 mol L−1 AcN in BFEE + 20% EE or in CH3CN + 0.1 mol L−1Bu4NBF4 (Fig. 2, inset) on a Pt wire electrode at the potential scan rate of 500 mV s−1 are shown in Fig. 2. The CVs of AcN in BFEE show characteristic features of conducting polymers during potentiodynamic synthesis such as benzanthrone.16 As the CV scan continued, the film was formed on the working electrode surface from brown to deep brown as it deposited, and the PAcN film can be reduced at −1.09 V. The increase in the redox wave currents implied that the PAcN film on the electrode was increasing. Although in the medium of CH3CN + 0.1 mol L−1Bu4NBF4, the PAcN film could be reduced and oxidized between −1.6 V and −1.86 V, there was little polymer formed on the electrode surface. The main reason for this phenomenon is the high oxidation potential of AcN. However, in both of media at a lower potential scan rate, the CVs did not show any redox wave in the same potential range. A possible reason is that the scan rate is much slower than the rate of the reverse reaction, thus the reverse peak will disappear totally.32 Obviously, CVs of AcN tested in BFEE + 20% EEsolvent system were very successful.
Electrochemical performance of PAcN films
The electrochemical behavior of the prepared PAcN films from BFEE + 20% EE was studied carefully in monomer-free concentrated sulfuric acid (SA). The result is shown in Fig. 3. The steady-state CVs represented broad anodic and cathodic peaks. The peak current densities were proportional to the scanning rates (inset of Fig. 3), indicating the reversible redox behavior of the polymer. Furthermore, these films could be cycled repeatedly between the conducting (oxidized) and insulating (neutral) state without significant decomposition of the materials, indicating the high structural stability of this conducting polymer. The polymer films obtained from BFEE + 20% EE can be oxidized and reduced at 0.53 V (anodic peak potential, Ea) and 0.40 V (cathodic peak potential, Ec) in monomer-free SA at the scan rate of 100 mV S−1. With the scan rate increasing, the peak potentials shifted positively and negatively to about 0.55 V (Ea) and 0.32 V (Ec), respectively. This indicated high stability of PAcN films even in concentrated SA. Moreover, the small anions in concentrated SA (SO42− or HSO4−) can probably facilitate the doping and dedoping process.33 This led to the good redox activity of PAcN films in concentrated SA. In a word, PAcN films showed good redox activity even in concentrated sulfuric acid. The as-obtained PAcN film from the electrolytes was also in a doped state, later proved to be conducting and doped with ions.
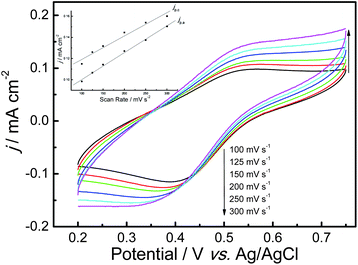 |
| Fig. 3
Cyclic voltammograms of a PAcN film in monomer-free SA at different potential scan rates. The PAcN film was synthesized electrochemically in BFEE + 20% EE at a constant applied potential of 1.2 V. Inset: plots of redox peak current densities vs. potential scan rates. jp is the peak current density, and jp,a and jp,c denote the anodic and cathodic peak current densities, respectively. | |
To remove the doping ions in doped PAcN, the as-produced PAcN was treated with 25% ammonia. The obtained polymer is named dedoped PAcN. The polymer color changed slightly from brown to dark. What is more, both the doped and dedoped PAcN films are partly soluble in the solvents such as tetrhydrofuran, dimethyl sulfoxide (DMSO), and CHCl3. This facilitates characterization in solution. Additionally, it was easily spin-coated on substrates like glass slides or silicon slides.
Molecular weight and its polydispersity
The molecular weights of the dedoped PAcN were determined by MALS-GPC analysis. MALS/GPC provides an absolute measure of weight average molecular weight (Mw) and a column-dependent measure of number average molecular weight (Mn).34 The Mw of the dedoped PAcN is 426000 g mol−1, and Mn is about 403000 g mol−1. This indicates the prepared PAcN has a long chain or high polymer degree. The obtained molecular weights of PAcN here are much higher than those prepared by radical polymerization,29 and much lower than those produced by thermal polymerization,30 moreover, these values are much higher than those of other CPs electropolymerized.16,35 The polydispersity index (Mw/Mn) is calculated as 1.06, close to 1.00, which means the electropolymerized PAcN in this work has a narrow distribution of molecular weights.
Structural characterization
The UV-visible spectra of AcN, doped PAcN and dedoped PAcN dissolved in DMSO are shown in Fig. 4. The AcN monomer shows two strong absorption bands at around 265 nm and 323 nm [Fig. 4 (A)], while spectra of the doped [Fig. 4 (B)] and dedoped [Fig. 4 (C)] PAcN films show much wider absorption bands at around 386 nm, except for the similar sized absorption band at around 268 nm, the overall absorption of PAcN tails off at about 681 nm. The red shift of the UV-vis spectra of PAcN means the that the polymer has formated with a high density of excimers or with the higher conjugation backbone in comparison with the monomer.
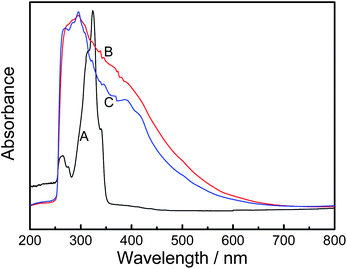 |
| Fig. 4
UV-visible spectra of AcN (A), doped PAcN (B) and dedoped PAcN (C) prepared from mixed electrolyte of BFEE + 20% EE potentiostatically at 1.2 V. Solvent: DMSO. | |
Fig. 5 shows the FTIR spectra of AcN (A), doped PAcN (B) and dedoped PAcN (C) films, respectively. The bands at 3038 cm−1 and 3057 cm−1 are attributed to the C–H stretching of the ethylene of the monomer, and 1424 cm−1 is attributed to the symmetric in-plane bending vibration of C–H bonds [Fig. 5 (A)]. The band at 3045 cm−1 is attributed to the C–H stretching of the ethylene of both doped PAcN and dedoped PAcN [Fig. 5 (B, C)]. The bands near 1623 cm−1 and 1490 cm−1 in monomer and the bands centered at 1595–1600 cm−1 and 1490 cm−1 for both PAcN films can be ascribed to the vibration of the C
C on the naphthalene ring respectively [Fig. 5 (A, B, C)].
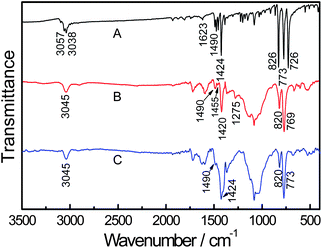 |
| Fig. 5
FT-IR spectra of AcN (A), doped PAcN (B) and dedoped PAcN (C). | |
The bands centered at 826 and 773 cm−1 in Fig. 5 (A) are assigned to the C–H out-of-plane vibration of benzene ring of the monomer, as well as those at 820 and 769 cm−1 for both PAcNpolymers [Fig. 5 (B, C)], which indicates that the 1,2,3-tetrasubstitution of benzene rings exist in both AcN and PAcN molecules. The disappearance of the 726 cm−1 band in both polymers [Fig. 5 (B, C)], corresponding to the out-plane bending vibration of =C–H bond of the ethylene group for AcN in Fig. 5 (A), indicates the polymerization site of AcN is the double bond of the ethylene group in AcN. This is similar to those prepared by other methods.30,31 The peaks at 1455 cm−1 and 1275 cm−1 in Fig. 5 (B) are assigned to B–F stretching and C–O–C stretching of BFEE, respectively, however, they are not appeared in dedoped of PAcN. This indicates that [BF3·Et3O]− or BF4− are removed from the PAcN films at the dedoping state.
The 1H-NMRspectrum of dedoped PAcN was also tested with d6-DMSO as a solvent. As shown in Fig. 6, except two strong proton peaks at 2.50 ppm and 3.35 ppm coming from d6-DMSO and the residual water, respectively, there is a broad and wide peak in the range of 7–8 ppm representing the phenylproton of the naphthyl group. It reveals a typical polymerspectrum of the as-prepared PAcN.
Fluorescence properties
It is widely acknowledged that fused ring compounds have good fluorescence properties. Therefore, it is very necessary to investigate the fluorescence properties of the prepared PAcN films. The fluorescence spectra of the AcN, doped PAcN and dedoped PAcN films prepared in BFEE + 20% EE were examined in DMSO. As shown in Fig. 6, the emission spectra of AcN [Fig. 7 (A)] obtained at the maximum excitation of 384 nm exhibits several peaks mainly at 418 nm and 444 nm in the range of 400–550nm. While the emission spectra of both doped [Fig. 7 (B)] and dedoped [Fig. 7 (C)] PAcN films appear in the higher and wider wavelength range of 450–700 nm, with several peaks mainly at 518 and 553 nm. There is a large bathochromic shift (about 100 nm) in the emission wavelength between the monomer and the polymers.
The broadened and red-shifted emission band of PAcN originates from the polymer excimers produced by interchain interactions of linear PAcN molecules in the DMSO solution. Such linear polymers, not like hyperbranched polymers,36 easily tend to high chain entanglement, which results in the fluorophore moieties becoming closer to each other, leading to the formation of interchain excimers. Compared with the emission band of PAcN prepared by radical polymerization,29 that of PAcN polymerized electrochemically shifts from blue to green emission band, which is due to the higher molecular weight or the longer chain of PAcN formed in this work.
Also, the fluorescence intensities of the PAcNpolymers are stronger than that of the monomer. This is probably explained by the termed fluorescence “Structural Self-Quenching Effect” (SSQE) phenomenon,37 which is caused by the intramolecular charge-transfer interaction between the electron–donor (fused ring chromophore) and electron–acceptor (carbon–carbon double bonds) in the same molecule. Of course, there are also some unclear reasons which need to be investigated in the future.
What is more, the PAcN films can emit strong photoluminescence when exposed to 365 nm UV light. The photographs of AcN, doped PAcN, and dedoped PAcN and the photoluminescence images under UV light irradiation of 365 nm are shown in Fig. 8 (A, B). Compared with the photographs in Fig. 8 A, soluble PAcN samples in both doped [Fig. 8 B (b)] and dedoped [Fig. 8 B (c)] states exhibit bright green-light-emitting properties, whereas the monomer shows no emissions under the same condition [Fig. 8 B (a)]. These results demonstrate that PAcN may be a good candidate in green light-emitting materials.
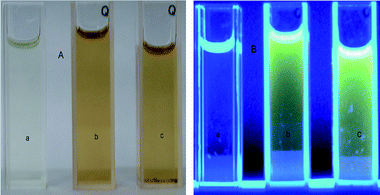 |
| Fig. 8 The photographs (A) of AcN (a), doped PAcN (b), and dedoped PAcN (c) and their photoluminescence images (B) under UV light irradiation of 365 nm. Solvent: DMSO. | |
The fluorescence quantum yields (Φoverall) of the soluble samples were measured by using anthracene in acetonitrile (standard, Φref = 0.27 38) as a reference and calculated according to the well-known method based on the expression:
| 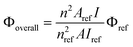 | (1) |
where
n,
A, and
I denote the refractive index of the
solvent, the absorbance at the excitation wavelength, and the intensity of the
emission spectrum, respectively. According to
eqn (1), the fluorescence quantum yield (
Φoverall) of AcN monomer was calculated to be only 0.075. The
Φoverall of the doped and dedoped PAcN were 0.16 and 0.30, 2 times and 4 times more than the monomer, respectively. The fluorescence quantum yields of PAcN are much higher than many
polymers of
naphthalene derivatives, such as poly((S)-(−)-1,1′-bi-2-naphthol dimethyl
ether) (PBNME,
Φoverall = 0.15)
16 and poly(1,5-dihydroxynaphthalene) (
Φoverall = 0.1).
24 These results indicate that PAcN may be a good candidate for the materials used in light-emitting diodes.
Thermal analysis
Thermal stability of CPs is very important for their potential application. Thermogravimetric analyses (TGA) and differential thermal analyses (DTA) are significantly dynamic ways to detect the degradation behaviors of CPs. The weight loss of a polymer sample is measured continuously, whereas the temperature is changed at a constant rate. The thermal analysis of dedoped PAcN film was tested, as shown in Fig. 9. It was performed under a nitrogen stream in the temperature range of 323–1073 K, with a heating rate of 10 K min−1. As can be seen from Fig. 9, there is a slight weight loss from 323 to 428 K, of up to 2.9%. This degradation can be ascribed to waterevaporation or other moisture trapped in the polymer. The decomposition up to 35.5% occurs between 428 and 926 K. This weight loss is attributed to the degradation of the PAcN backbone chain structure. The total weight loss is less 40%. All these results indicate that PAcN films have good thermal stability.
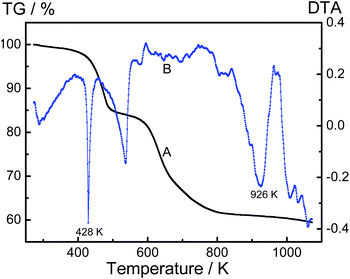 |
| Fig. 9
TG (A) and DTA (B) curves of dedoped PAcN. | |
Morphology and conductivity
The SEM images of doped and dedoped PAcN films deposited electrochemically on the ITO electrodes from BFEE + 20% EE are shown in Fig. 10. Macroscopically, both the doped and dedoped PAcN films appear to be flat and compact [Fig. 10, (A, B)]. Microscopically, the polymer film resembles ordered arrangements of the granules. The growth of the nuclei is in the form of clusters. This morphology facilitates the movement of doping anions into and out of the polymer film during doping and dedoping, in good agreement with the high redox activity of the PAcN films.
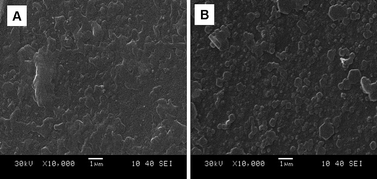 |
| Fig. 10
SEM images of doped (A) and dedoped PAcN (B) deposited on the ITO electrode surface from BFEE + 20% EE at a constant applied potential of 1.2 V. | |
The electrical conductivity of the pressed pellet of doped PAcN films obtained from BFEE + 20% EE was measured to be 0.43 S cm−1 by the conventional four-probe technique, higher than that of polyacenaphthene (10−2 S cm−1).13 This means the doped PAcN prepared in this work is a conducting polymer.
Experimental
Materials
AcN (Aladdin-reagent, 90%; ShangHai), BFEE (Sinapharm Chemical Reagent Co., Ltd) and tetrabutylammonium tetrafluoroborate (Bu4NBF4, 98%; TCl, Japan) were obtained commercially. BFEE was purified by distillation before use. Dimethyl sulfoxide (DMSO), anhydrous ethylether (EE), acetonitrile (CH3CN) and acetone were all A.R. grade and used directly without further purification. Concentrated sulfuric (SA) acid and 25% ammonia was used as received.
Electrochemical polymerization of PAcN films
All the electrochemical experiments were performed in a three-electrodecell using a CHI660B workstation (Shanghai, Chenhua). Platinum was used as working and counter electrode in wire and foil shapes. For electrochemical examinations, the working and counter electrodes were wire with the diameter of 0.3 and 0.5 mm, respectively. They were placed 5 mm apart during the experiment. Prior to each experiment, these electrodes mentioned above were carefully polished with abrasive papers (1500 mesh) and cleaned successively with water and acetone, and then dried in air. To obtain a sufficient amount of polymer for characterization, a Pt foil with 2 cm2 surface area was used as the working electrode, and a 4 cm2 foil was used as the counter electrode. All potentials were referred to a Ag/AgCl reference electrode. The amount of the polymer deposited on electrode was controlled by the total charge passed through the cell which was read directly from the current–time curves. To remove the adsorbed monomer, electrolyte, and oligomer, the obtained PAcN films were rinsed with anhydrous EE and pure water respectively. The formed polymer (doped PAcN) was scraped from the Pt foil. For spectral analysis, the scraped polymer was dedoped with 25% ammonia for 3 days and then washed repeatedly with pure water, signed as dedoped PAcN. Finally, it was dried in vacuum at 60 °C for 24 h. All experiments were carried out at the temperature 22 ± 2 °C.
Characterization
Fourier transform infrared (FT-IR)
spectra were recorded with a Bruker Vector 22 FT-IR spectrometer in the region of 400–4000 cm−1 using KBr pellets. Ultraviolet-visible (UV-vis) spectra were obtained with a Perkin–Elmer Lamda 900 spectrophotometer. The 1H NMR spectrum was recorded on a Bruker 300 NMR spectrometer with d6-DMSO as the solvent and tetramethylsilane as an internal standard (TMS, singlet, chemical shift: 0.0 ppm). The fluorescence spectra were determined with a RF-540 fluorescence spectrophotometer (Shimadzu, Japan). Scanning electron microscopy (SEM) measurements were carried out on a JEOL JSM-6380LV and JEM-2100electron microscopes. The conductivity of as-formed PAcN films was measured by a RTS-9 4-point resistivity measurement system. Thermogravimetric analyses (TGA) and differential thermal analyses (DTA) were performed on a Pyris DiamondTG/DTA thermal analyzer (Perkin-Elmer). The molecular weight of the polymer was analyzed with a Wyatt multi-angle laser scattering-gel permeation chromatography (MALS-GPC) system. A PSS SDV column was used with tetrahydrofuran as eluent at a flow rate of 1 mL min−1. A spin coating device P 6700 (Specialty Coating Systems Inc., Indianapolis/USA) was used to prepare the polymer films on glass slides.
Conclusions
Conducting PAcN films with conductivity of 0.43 S cm−1 have been electrochemically synthesized in BFEE + 20% EE in the first time. The electropolymerized PAcN film with high quality has the average molecular weight as high as 426,000 g mol−1, and a narrow distribution of molecular weights. The as-prepared PAcN film in the mixed electrolyte is proved to be doped with [BF3·Et3O]− or BF4− at the doping state. Thermal analysis indicates PAcN has good thermal stability. The absorption of the polymer tails to the visible light region in its UV-vis spectrum. Remarkably, compared with the emission band of PAcN prepared by radical polymerization, that of the electropolymerized PAcN shifts from blue to green light region. The electrochemically prepared PAcN films in both doping and dedoping states exhibit excellent green-light-emitting properties. All these results mean that the electropolymerized PAcN film is a good conducting material and a green-light emitter, which show good application prospects in the development of electronic and organic light-emitting devices.
Acknowledgements
This work was supported by NSFC (21073092), Science and Technology Supporting item of Jiangsu Province, China (No. BE2009159). SRF for ROCS, State Education Ministry and Ministry of Personnel of the PRC (2006), NUST Research Funding (ZDJH07) and Excellent Plan Foundation of NUST (2008).
Notes and references
- A. Heeger, Rev. Mod. Phys., 2001, 73, 681 CrossRef CAS.
- U. Lange, N. V. Roznyatovskaya and V. M. Mirsky, Anal. Chim. Acta, 2008, 614, 1 CrossRef CAS.
- T. Ahuja, I. A. Mir and D. K. Rajesh, Biomaterials, 2007, 28, 791 CrossRef CAS.
- S. Günes, H. Neugebauer and N. S. Sariciftci, Chem. Rev., 2007, 107, 1324 CrossRef.
- R. J. Mortimer, A. L. Dyer and J. R. Reynolds, Displays, 2006, 27, 2 CrossRef CAS.
- S. Brady, K. T. Lau, W. Megill, G. G. Wallace and D. Diamond, Synth. Met., 2005, 154, 25 CrossRef CAS.
- Y. Yoshioka and G. E. Jabbour, Synth. Met., 2006, 156, 779 CrossRef CAS.
- H. G. O. Sandberg, T. G. Bäcklund, R. Österbacka, S. Jussila, T. Mäkelä and H. Stubb, Synth. Met., 2005, 155, 662 CrossRef CAS.
- N. E. Agbor, M. C. Petty and A. P. Monkman, Sens. Actuators, B, 1995, 28(3), 173–179 CrossRef.
- R. Nohria, R. K. Khillan, Y. Su, R. Dikshit, Y. Lvov and K. Varahramyan, Sens. Actuators, B, 2006, 114, 218 CrossRef.
- Q. Hao, V. Kulikov and V. M. Mirsky, Sens. Actuators, B, 2003, 94, 352 CrossRef.
- Q. Hao, X. Wang, L. D. Lu, X. J. Yang and V. M. Mirsky, Macromol. Rapid Commun., 2005, 26, 1099 CrossRef CAS.
- H. T. Liu, J. K. Xu, S. Z. Pu, M. Luo and Z. Le, Mater. Lett., 2007, 61, 1392 CrossRef CAS.
- L. Shen, J. K. Xu, Z. Wei, Q. Xiao and S. Z. Pu, Eur. Polym. J., 2005, 41, 1738 CrossRef CAS.
- C. L. Fan, J. K. Xu, W. Chen, B. Y. Lu, H. M. Miao, C. C. Liu and G. D. Liu, J. Phys. Chem. C, 2009, 113, 9900 CrossRef CAS.
- B. Y. Lu, J. K. Xu, C. L. Fan and H. M. Miao, J. Phys. Chem. B, 2009, 113, 37 CrossRef CAS.
- W. Q. Zhou, M. P. Guo, J. K. Xu and X. L. Yuan, Eur. Polym. J., 2008, 44, 656 CrossRef CAS.
- B. Dong, Y. H. Xing, J. K. Xu, L. Q. Zheng, J. Hou and F. Zhao, Electrochim. Acta, 2008, 53, 5745 CrossRef CAS.
- G. M. Nie, Y. Zhang, J. K. Xu and S. S. Zhang, J. Electroanal. Chem., 2008, 622, 121 CrossRef CAS.
- D. X. Zhuang and P. Y. Chen, J. Electroanal. Chem., 2009, 626, 197 CrossRef CAS.
- A. Sezai Sarac, M. Ates and E. A. Parlak, J. Appl. Electrochem., 2006, 36, 889 CrossRef.
- Q. Hao, M. Rahm, D. Weiss and V. M. Mirsky, Microchim. Acta, 2003, 143, 147 CrossRef CAS.
- B. Y. Lu, J. K. Xu, C. L. Fan, F. Jiang and H. M. Miao, Electrochim. Acta, 2008, 54, 334 CrossRef CAS.
- B. Y. Lu, L. Q. Zeng, J. K. Xu and Z. G. Le, Eur. Polym. J., 2009, 45, 2279 CrossRef CAS.
- Y. Xie, F. Jiang, J. K. Xu, L. Q. Zeng, B. Dong, C. L. Fan and F. Zhao, Synth. Met., 2009, 159, 298 CrossRef CAS.
- H. Bai, C. Li and G. Q. Shi, Sens. Actuators, B, 2008, 130, 777 CrossRef.
- M. Chen, K. P. Ghiggino, A. W. H. Mau, E. Rizzardo, S. H. Thang and G. J. Wilson, Chem. Commun., 2002, 2276 RSC.
- T. A. Smith and K. P. Ghiggino, Polym. Int., 2006, 55, 772 CrossRef CAS.
- J. Springer, J. Schmelzer and T. Zeplichal, Chromatographia, 1980, 13, 164 CrossRef CAS.
- H. Agbaje and J. Springer, Eur. Polym. J., 1986, 22, 943 CrossRef CAS.
- J. Moacanin, A. Rembaum, R. K. Laudenslager and R. Adler, J. Macromol. Sci., Part A: Pure Appl. Chem., 1967, 1, 1497 Search PubMed.
-
Y. Imai and M. Kakimoto, Handbook of Polymer Science and Technology: Synthesis and Properties, Marcel Dekker, New York, 1988, pp. 275–289 Search PubMed.
- L. Q. Zeng, Z. Le, J. K. Xu, H. T. Liu, F. Zhao and S. Z. Pu, J. Mater. Sci., 2008, 43, 1135 CrossRef CAS.
- H. S. Kolla, S. P. Surwade, X. Zhang, A. G. MacDiarmid and S. K. Manohar, J. Am. Chem. Soc., 2005, 127, 16770 CrossRef CAS.
- M. R. A. Alves, H. D. R. Calado, C. L. Donnici and T. Matencio, Synth. Met., 2010, 160, 22 CrossRef CAS.
- C. Gao, J. Hou, D. Yan and Z. Wang, React. Funct. Polym., 2004, 58, 65 CrossRef CAS.
- F. M. Li, S. J. Chen, Z. C. Li and J. Qiu, J. Polym. Sci., Part A: Polym. Chem., 2000, 34, 1881.
- C. Zimmermann, M. Mohr, H. Zipse, R. Eichberger and W. Schnabel, J. Photochem. Photobiol., A, 1999, 125, 47 CrossRef CAS.
|
This journal is © The Royal Society of Chemistry 2011 |
Click here to see how this site uses Cookies. View our privacy policy here.