DOI:
10.1039/C0PY00326C
(Paper)
Polym. Chem., 2011,
2, 651-658
Synthesis, characterization and cellular internalization of poly(2-hydroxyethyl methacrylate) bearing α-D-mannopyranose
Received
30th September 2010
, Accepted 1st November 2010
First published on 26th November 2010
Abstract
2-(α-D-Mannopyranosyloxy)ethyl methacrylate (ManEMA) was synthesized in stereochemically pure form, and isolated as an easy-to-handle dry white solid. Poly(ManEMA-co-HEMA)s were prepared by conventional radical copolymerization of ManEMA with 2-hydroxyethyl methacrylate (HEMA). The monomer reactivity ratio rManEMA and rHEMA were estimated to be 1.00 and 0.50, respectively. The initial clustering rate of poly(ManEMA-co-HEMA) with a mannose-binding lectin, concanavalin A, evaluated by turbidimetric assay, increased with increasing mole fraction of ManEMA units in the polymer, even at constant ManEMA unit concentration. This cluster glycoside effect of the linear glycopolymer, poly(ManEMA-co-HEMA), could be well interpreted by the sequence distribution of ManEMA units calculated on the basis of the terminal model. The cellular uptake and cytotoxicity of fluorescently labeled poly(ManEMA) was examined in HeLa cells. Confocal laser scanning microscope imaging revealed the endocytotic internalization of poly(ManEMA) in HeLa cells. The survival rate of HeLa cells treated with poly(ManEMA) was estimated to be 95.7 ± 1.7% versus untreated cells. These results indicate that ManEMA is a promising monomer for development of medicinal glycopolymers.
Introduction
Carbohydrate plays an important role in the biological functions of glycoproteins, such as cellular trafficking, protein quality control, cellular adhesion and so on.1–3 These functions depend upon interactions of carbohydrates with carbohydrate-recognition proteins or enzymes. Therefore, it is attractive to utilize carbohydrate as a functional element, and in the past decade, synthetic polymers bearing carbohydrate moieties on the side chains, so-called glycopolymers, have become an important topic in polymer chemistry and medicinal chemistry.4–8 Many glycopolymers have been synthesized and utilized as polysaccharide mimics,9drug and gene carriers,10inhibitors of viral infection,11 agents for tissue engineering,12,13 diagnostic drugs14 and so on. On the other hand, 2-hydroxyethyl methacrylate (HEMA) is a well-known vinyl monomer; the corresponding polymer is one of the most successful biologically compatible materials for optical lens15 and ocular implants,16 and has also been tested for application as a dental material,17 as a scaffold for tissue engineering18 and as an agent to promote nerve regeneration.19 Hence, glycopolymers based on poly(HEMA) are expected to be a good platform for developing diverse biomaterials. O-Glycosylated HEMA20–34 is a basic class of vinyl monomer for the synthesis of poly(HEMA) bearing carbohydrate moieties. Although various carbohydrates have been used for O-glycosylation of HEMA, to our knowledge, only four O-glycosylated HEMAs have been obtained in stereochemically pure form, namely 2-(β-D-glucopyranosyloxy)ethyl methacrylate,22,302-(β-D-galactopyranosyloxy)ethyl methacrylate,21,28,302-(N-acetyl-β-D-glucosaminopyranosyloxy)ethyl methacrylate,23,24,26 and 2-(β-D-galactopyranosyl-(1–4)-β-D-glucopyranosyloxy)ethyl methacrylate27 (hereinafter referred to as GlcEMA, GalEMA, GlcNAcEMA and LacEMA, respectively). The stereochemistry of O-glycosylation greatly affects the functions of the carbohydrates, and hence its control is a central topic in synthetic carbohydrate chemistry. For example, basic winged bean lectin binds methyl α-D-galactopyranoside with a 6-times-greater binding constant than the β-anomer.35
Mannose is a naturally abundant carbohydrate and also plays an important role in microbial infection. Mannose-binding protein is a typical C-type lectin exerting pattern recognition of high-mannose type glycoprotein and a key component of innate immunity. Mannose can be utilized as a homing device to antigen-presenting cells such as macrophages and dendritic cells, which abundantly express mannose receptor.36,37 In this article, we report synthesis of stereochemically pure O-mannosylated HEMA, namely 2-(α-D-mannopyranosyloxy)ethyl methacrylate (ManEMA). Statistical copolymers of ManEMA and HEMA were prepared and their multivalent ligand nature with respect to a mannose-binding lectin, concanavalin A (ConA), was examined. The clustering behavior of the copolymers and ConA is discussed in the framework of the terminal model of statistical copolymerization. In addition, the cellular localization and cytotoxicity of fluorescently labeled poly(ManEMA) were evaluated to explore the biological compatibility of the newly synthesized monomer ManEMA.
Experimental section
Materials and analytical techniques
All chemicals were of analytical grade. LysoTracker Red was purchased from Molecular Probes (Eugene, OR, USA). 1,2,3,4,6-Penta-O-acetyl-β-D-mannopyranose (AcMan) was prepared according to the literature.381H and 13C NMR spectra were recorded using JNM-AL400 (400 MHz, JEOL Ltd., Tokyo, Japan), AVANCE 400 (400 MHz, Bruker Biospin K. K. Yokohama, Japan) and JNM-EC600 (600 MHz, JEOL Ltd., Tokyo, Japan) instruments. Electronic absorption spectra were recorded on a JASCO V-570 spectrophotometer (JASCO Co., Ltd, Tokyo, Japan). Electron-spray ionization time-of-flight (ESI-TOF) mass spectra were taken on a JEOL JMS-T100LC (JEOL Ltd, Tokyo, Japan). Elemental analyses were performed on a Perkin-Elmer 2400 series II CHNS/O elemental analyzer (Perkin Elmer Inc., MA, USA). Molecular weight of polymer was measured by aqueous size exclusion chromatography (SEC) using Ultrahydrogel Linear (blend, 7.8 × 300 mm) (Waters Co., MA, USA) and Ultrahydrogel 500 (pore size: 500 Å, 7.8 × 300 mm) (Waters Co.) columns equipped with a refractive index detector at a flow rate of 0.5 mL min−1 with 0.1 M NaNO3 (aq.) containing 0.05 wt% NaN3 as a mobile phase. Number-averaged molecular weight (Mn) was calculated on the basis of pullulan standards. Morphological changes of the cells were observed using a confocal laser scanning microscope (CLSM) (Model LSM 510, Carl Zeiss, Jena, Germany).
2-(2,3,4,6-Tetra-O-acetyl-α-D-mannopyranosyloxy)ethyl methacrylate (AcManEMA)
1,2,3,4,6-Penta-O-acetyl-β-D-mannopyranose (10.0 g, 25.6 mmol) and 2-hydroxyethyl methacrylate (3.20 mL, 29.1 mmol) were dissolved in dry CH3CN (100 mL). To the solution was added 19.4 mL (154 mmol) of BF3·OEt2 with cooling on an ice-bath. The mixture was stirred at 20 °C for 12 hours followed by stirring at ambient temperature for an additional 14 hours. The resulting mixture was diluted with ethyl acetate (150 mL) and poured into ice-water. The organic solution was washed with water (200 mL × 2), sat. NaHCO3 (aq.) (150 mL × 2), and brine (150 mL × 2), dried over anhydrous Na2SO4, and evaporated under reduced pressure to give a white solid. The crude product was purified by column chromatography (silica gel, acetone
:
hexane = 3
:
7) to give AcManEMA as a white solid. Yield 4.58 g (9.95 mmol, 39%). 1H NMR (600 MHz, CDCl3, Si(CH3)4 = 0 ppm): δ (ppm) = 6.13 (m, 1H, CH2
C), 5.60 (m, 1H, CH2
C), 5.35 (dd, 3J = 9.96 and 3.42 Hz, 1H, 3-ManH), 5.29–5.26 (m, 2H, 2,4-ManH), 4.88 (d, 3J = 1.9 Hz, 1H, 1-ManH), 4.35–4.34 (m, 2H, –CH2–O–C
O), 4.27 (dd, 2J = 12.12 Hz, 3J = 5.58 Hz, 1H, 6-ManH), 4.09 (dd, 2J = 12.30 Hz, 3J = 2.34 Hz, 1H, 6-ManH), 4.03 (ddd, 3J = 9.89, 5.36 and 2.30 Hz, 1H, 5-ManH), 3.92 (ddd, 2J = 11.45 Hz, 3J = 5.69 and 4.13 Hz, 1H, –CH2–CH2–O–C
O), 3.78 (ddd, 2J = 11.46 Hz, 3J = 5.37 and 4.14 Hz, 1H, –CH2–CH2–O–C
O), 2.16 (s, 3H, O
C–CH3), 2.10 (s, 3H, O
C–CH3), 2.04 (s, 3H, O
C–CH3), 2.00 (s, 3H, O
C–CH3), 1.96 (t, 4J = 1.08 Hz, 3H, C–CH3). 13C{1H} NMR (150 MHz, CDCl3, CDCl3 = 77 ppm): δ (ppm) = 170.3 (CH3–C
O), 169.6 (CH3–C
O), 169.5 (CH3–C
O), 169.3 (CH3–C
O), 166.7 (C
O), 135.6 (CH2
C(CH3)), 125.8 (
CH2), 97.2 (1-ManC), 69.2 (ManC), 68.8 (ManC), 68.4 (–CH2–CH2–O–C
O), 65.9 (ManC), 65.7 (ManC), 63.0 (–CH2–O–C
O), 62.2 (ManC), 20.86 (O
C–CH3), 20.69 (O
C–CH3), 20.66 (O
C–CH3), 18.3 (C–CH3).
2-(α-D-Mannopyranosyloxy)ethyl methacrylate (ManEMA)
2-(2,3,4,6-Tetra-O-acetyl-α-D-mannopyranosyloxy)ethyl methacrylate (10.0 g, 21.8 mmol) was dissolved in dry CH3OH (50 mL). To the solution was added methanolic NaOCH3. After an appropriate time, the solution was neutralized with cation-exchange resin (DOWEX 50 W). After removal of the resin, the solvent was evaporated under reduced pressure to give a yellow oil. The crude product was purified by flash column chromatography (silica gel, acetone
:
hexane = 7
:
3) to give ManEMA as a white solid. Yield 3.76 g (12.9 mmol, 59%). Anal. calcd for C12H20O8: C 49.31, H 6.90, N 0.00%. Found: C 48.92, H 6.73, N 0.09%. 1H NMR (600 MHz, CD3OD, CHD2OD = 3.30 ppm): δ (ppm) = 6.11 (s, 1H,
CH2), 5.63–5.61 (m, 1H,
CH2), 4.80 (d, 3J = 1.6 Hz, 1H, 1-ManH), 4.33 (ddd, 2J = 12.06 Hz, 3J = 6.30 and 3.19 Hz, 1H, –CH2–O–C
O), 4.28 (ddd, 2J = 12.06 Hz, 3J = 6.30 and 3.35 Hz, 1H, –CH2–O–C
O), 3.95 (ddd, 2J = 11.60 Hz, 3J = 6.30 and 3.19 Hz, 1H, –CH2–CH2–O–C
O), 3.82–3.54 (m, 7H, 2,3,4,5,6-ManH and –CH2–CH2–O–C
O), 1.93 (s, 3H, CH3). 13C{1H} NMR (150 MHz, CD3OD, CD3OD = 49 ppm): δ (ppm) = 168.7 (C
O), 137.6 (
C<), 126.4 (
CH2), 101.6 (1-ManC), 74.8 (ManC), 72.5 (ManC), 72.1 (ManC), 68.5 (ManC), 66.3 (–CH2–O–C
O), 64.8 (–CH2–CH2–O–C
O), 62.9 (6-ManC), 18.4 (CH3).
7-Amino-4-trifluorocoumarin 187 mg (0.82 mmol), dried K2CO3 228 mg (1.65 mmol), and CH3CN (6 mL) were placed in a 50 mL flask. To the suspension was added 1.6 mL of acetonitrile solution of 4-vinylbenzyl iodide. The mixture was stirred at room temperature under argon for 2 days, then evaporated under reduced pressure, and the residue was suspended in CHCl3. The insoluble salt was removed by filtration. The filtrate was purified by gel permeation chromatography to give a yellow powder (255 mg, 30%). 1H NMR (400 MHz, CDCl3, Si(CH3)4 = 0 ppm): δ (ppm) = 7.46 (dq, 3J = 8.91 Hz, 5JH–F = 1.92 Hz, 1H, 5-coumarinH), 7.40 (d, 3J = 8.06 Hz, 2H, 3,5-PhH), 7.29 (d, 3J = 8.30 Hz, 2H, 2,6-PhH), 6.70 (dd, 3J = 10.9 and 17.6 Hz, 1H, –CH
CH2), 6.58 (dd, 3J = 8.91 Hz, 4J = 2.38 Hz, 1H, 6-coumarinH), 6.49 (d, 4J = 2.38 Hz, 1H, 8-coumarinH), 6.43 (s, 1H, 3-coumarinH), 5.75 (d, 3J = 17.6 Hz, 1H, –CH
CH2), 5.26 (d, 3J = 10.9 Hz, 1H, –CH
CH2), 4.83 (s, 1H, –NH–), 4.40 (d, 3J = 3.97 Hz, 2H, –NH–CH2–). 13C{1H} NMR (100 MHz, CDCl3, CDCl3 = 77 ppm): δ (ppm) = 159.99 (C), 156.74 (C), 151.64 (C), 141.63 (q, 2JC–F = 32.1 Hz, 4-coumarinC), 137.08 (C), 136.65 (C), 136.01 (–CH
CH2), 127.37 (CH), 126.60 (CH), 126.09 (C), 121.67 (q, 1JC–F = 274 Hz, –CF3), 114.17 (–CH
CH2), 111.32 (C), 108.94 (q, 3JC–F = 5.8 Hz, 3-coumarinC), 104.10 (C), 98.50 (C), 47.40 (–NH–CH2–). ESI-TOF HRMS: m/z for C19H14O2NF3Na ([M + Na]+) calcd 368.08743, found 368.08614 (error 1.29 mmu, 3.50 ppm). Anal. calcd for C19H14O2NF3·H2O (M·H2O): C 62.81, H 4.44, N 3.86%. Found: C 62.80, H 4.02, N 3.56%. IR (KBr): ν (cm−1) = 3365.6 (νRR′NH), 1627.8 (νC
O). UV-vis (c = 66.0 μM, DMSO, path length = 1 cm): λ/nm (ε/M−1 cm−1) = 394.5 (19.2 × 103).
Synthesis of poly(ManEMA-co-HEMA)
The radical copolymerization of ManEMA with HEMA was carried out using 2,2′-azobis(2-methylpropionitrile) (AIBN) in N,N-dimethylformamide (DMF) at 60 °C under nitrogen atmosphere. After an appropriate time, the polymerization mixture was cooled on an ice-bath, then diluted with water and dialyzed for 3 days. Lyophilization of the dialyzed solution gave the polymer as a white solid. The composition of the resulting polymer was determined from the peak area ratio of side groups and main chain regions in the 1H NMR spectrum (Fig. 1).
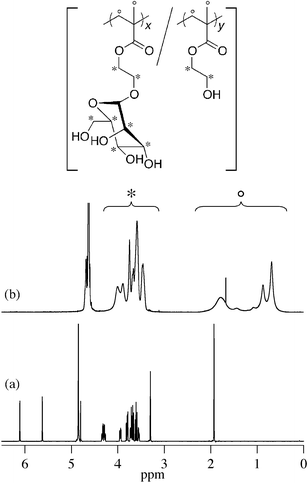 |
| Fig. 1
1H NMR spectra of ManEMA in CD3OD (a) and poly(ManEMA-co-HEMA) (run no. 5, FManEMA = 0.63) in D2O (b). | |
Synthesis of poly(ManEMA-co-C151St)
ManEMA (1.0 g, 3.44 mmol), C151St (8.03 mg, 0.023 mmol, mole fraction of C151StfC151St = 6.6 × 10−3), AIBN (10.1 mg, 0.061 mmol) and dry DMF (5 mL) were placed into a Schlenk tube, which was tightly sealed with a rubber septum. The tube was degassed by means of a freeze–pump–thaw cycle, and heated at 60 °C for 24 hours. The mixture was then diluted with deionized water and dialyzed using a cellulose ester membrane (molecular weight cut-off: 500). After lyophilization of the aqueous crude solution, the residue was purified by size exclusion chromatography using Sephadex G-15 and G-50 columns to remove low-molecular weight fluorescent compounds. After dialysis using a cellulose ester membrane (molecular weight cut-off: 1000), lyophilization of the aqueous polymer solution gave fluorescently labeled poly(ManEMA) as a greenish fluffy solid (0.7791 g, yield 72.2%). The mole fraction of C151St units in the poly(ManEMA-co-C151St) (FC151St) was estimated to be 5.9 × 10−3 by means of UV-vis spectrometry. Mn (Mw/Mn) = 2.59 × 104 (2.49).
Single-crystal X-ray diffraction data were recorded on Rigaku RAXIS RAPID imaging plate area detector using filtered Mo Kα radiation. All datasets were corrected for Lorentzian polarization effects and for absorption. All structures were solved by the direct method (SIR9239). Hydrogen atoms were placed at calculated positions, and refined with isotropic thermal parameters riding on those of the parent atoms. Refinement of the non-hydrogen atoms was carried out with the full-matrix least-squares technique. Crystal data: C12H20O8, monoclinic, space groupP21 (no. 4), a = 10.336(2), b = 9.4569(17), c = 15.074(3) Å, β = 106.776(5)°, V = 1410.8(5) Å3, Z = 4, Dcalc = 1.376 g cm−3, F000 = 624, μ = 0.1161 mm−1, collected reflections = 10
926, unique reflections = 4931, reflections with I > 2σ(I) = 3852, GOF = 1.078, R [F2 > 2σ(F2)] = 0.0618, Rint = 0.045, wR = 0.1575, Δρmax = 0.29 e Å−3, Δρmin = −0.32 e Å−3.
Turbidimetric assay
The binding behavior of poly(ManEMA-co-HEMA) with concanavalin A (ConA) was examined by measuring the turbidity change rate according to the literature40 with minor modifications. ConA was diluted to 1 μM (based on ConA tetramer) with 10 mM HEPES-buffered (pH = 7.4) saline containing 150 mM NaCl and 1 mM CaCl2 (hereinafter referred to as HBS). The ConA solution (2.7 mL) was transferred to a 1 cm cuvette, which was put into a thermostatted cell holder at 25 °C. Into the cuvette was rapidly injected 0.3 mL of HBS solution of poly(ManEMA-co-HEMA), in which the concentration of ManEMA unit was adjusted to be 500 μM. The absorbance at 420 nm was recorded for 10 min at 1 Hz.
Cellular internalization of poly(ManEMA-co-C151St)
HeLa cells (ATCC CCL-2) (1 × 104cells) in 500 μL of Dulbecco's modified Eagle's medium (DMEM, Nissui Pharmaceutical, Tokyo, Japan) containing 10% fetal calf serum (FCS) (Hyclone Laboratories, Inc., Logan, UT, USA) were plated in a 24-well plate (Nalge Nunc International, Naperville, IL, USA), and incubated for 24 hours (37 °C, 5% CO2). Five hundred microlitres of poly(ManEMA-co-C151St) in DMEM containing 10% FCS was added to each well (n = 2), and incubation was continued for 18 hours in the presence of the polymer. The final concentrations of the polymer were 5.0 mg mL−1. After removal of the medium, 500 μL of 75 nM LysoTracker Red in DMEM containing 10% FCS were added to each well. The cells were incubated for 1 hour (37 °C, 5% CO2). The cells were washed twice with 500 μL of Dulbecco's phosphate-buffered saline (PBS), and 500 μL of Hank's balanced salt solution (HBSS, Nissui Pharmaceuticals) was added to each well. Fluorescence images of LysoTracker Red and poly(ManEMA-co-C151St) in cells were taken with a confocal laser scanning microscope (CLSM) (LSM510, Carl Zeiss) using excitation wavelengths of 543 and 364 nm, respectively.
Cytotoxicity of poly(ManEMA-co-C151St)
HeLa cells (1 × 104cells) in 500 μL of DMEM containing 10% FCS were plated in a 24-well plate, and incubated for 24 hours (37 °C, 5% CO2). Five hundred microlitres of poly(ManEMA-co-C151St) in DMEM containing 10% FCS was added to each well (n = 2), and incubation was continued for 21 hours in the presence of the polymer. The final concentrations of the polymer were 5.0 mg mL−1. The cells were washed twice with 500 μL of PBS, and 500 μL of HBSS was added to each well. After the addition of WST-8 reagent (50 μL) from Cell Counting Kit-8 (Dojindo, Tokyo, Japan) to each well, the plate was further incubated for 3 hours (37 °C, 5% CO2). Then, the absorbance at 450 nm was measured using a plate reader. The percentage cell survival was calculated by normalization with respect to the value for cells with no polymer treatment.
Results and discussion
Synthesis of poly(ManEMA-co-HEMA)
2-(α-D-Mannopyranosyloxy)ethyl methacrylate (ManEMA) was synthesized in three steps from D-mannose. O-Glycosylation of peracetylated D-mannose with 2-hydroxyethyl methacrylate (HEMA) was conducted in dry acetonitrile using BF3·OEt2 as a Lewis acid (Scheme 1). Then removal of acetyl groups was carefully performed by using methanolic sodium methoxide to give ManEMA. The O-glycosylation proceeded with excellent α-anomer selectivity (α/β > 20) thanks to both steric and anomeric effects (Fig. 1a). In contrast to the hygroscopic and sticky nature of GlcEMA30 and GalEMA,30 ManEMA was obtained as a dry white solid after alcohol-free column chromatography. Moreover, it can be crystallized from a mixture of acetone and hexane. Fig. 2 shows the ORTEP drawing of ManEMA, clearly indicating a 4C1 chair conformation of α-D-mannopyranose.41 ManEMA is quite stable, whereas GlcEMA gradually polymerizes even at −20 °C. These characteristics make ManEMA a very convenient glycomonomer for studies on glycopolymer chemistry.
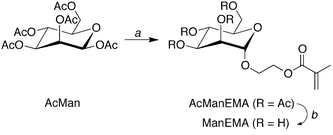 |
| Scheme 1 Synthesis of 2-(α-D-mannopyranosyloxy)ethyl methacrylate (ManEMA). Reagents and conditions: (a) 2-hydroxyethyl methacrylate, BF3·OEt2, dry CH3CN, r.t. and (b) NaOCH3, dry CH3OH. | |
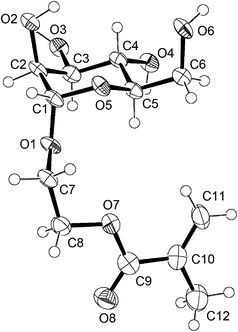 |
| Fig. 2 ORTEP drawing of ManEMA with thermal ellipsoids shown at the 50% probability level.41 | |
Radical copolymerizations of ManEMA with HEMA were carried out using 2,2′-azobis(2-methylpropionitrile) (AIBN) in N,N-dimethylformamide (DMF) at 60 °C (Scheme 2 and Table 1). The polymerizations proceeded homogeneously at every feed composition (fManEMA) examined. The resulting mixture was dialyzed to remove the remaining monomeric ManEMA and HEMA, and lyophilized to give a water-soluble white solid. The number-average molecular weights (Mns) of the polymers were determined by aqueous size exclusion chromatography (SEC) using pullulan standards. All aqueous SEC traces of the polymers were unimodal suggesting formation of copolymer not a mixture of homopolymers. The Mn values ranged from 1.90 × 104 to 17.1 × 104 and the Mw/Mn values ranged from 2.46 to 1.84. The mole fraction of ManEMA units in the polymer (FManEMA) was determined by 1H NMR spectroscopy (Fig. 1b and Table 1). The FManEMA value was greater than the corresponding fManEMA value in every case. Because of the high yield of the polymers, we used the integration form of the Mayo–Lewis equation to estimate monomer reactivity ratio.42 Non-linear least-squares fitting afforded a monomer reactivity ratio rManEMA of 1.00 and rHEMA of 0.50 (Fig. 3). Unexpectedly, ManEMA showed higher reactivity than HEMA in radical polymerization, despite the bulky sugar substituent, especially at lower feed composition of ManEMA. This high reactivity of ManEMA may be caused by a solvent effect, the so-called bootstrap effect,43 which results in high reactivity of a polar monomer in a less polar solvent. For example, Fernández-Monreal et al. reported a solvent effect on the radical copolymerization of HEMA with tert-butyl acrylate.44 They indicated that the monomer reactivity ratio of HEMA depended significantly on the polarity and hydrogen-bond forming ability of the solvent. The monomer reactivity ratio of HEMA is quite high in non-polar solvents owing to the selective solvation by HEMA of HEMA units around the growing chain end. In our case, ManEMA acts as a polar and hydrogen-bond-forming monomer in DMF, a relatively polar but aprotic solvent.
Run no. |
f
ManEMA
b
|
Yield (%) |
F
ManEMA
c
|
M
n × 10−4 (Mw/Mn)d |
[ManEMA + HEMA]0 = 0.7 M, [AIBN]0 = 10 mM, 60 °C, 1 h.
Mole fraction of ManEMA in the monomer feed.
Mole fraction of ManEMA unit in the copolymer, determined by 1H NMR spectroscopy.
Determined by aqueous SEC using a pullulan standard.
Not determined.
[ManEMA]0 = 0.5 M, [AIBN]0 = 10 mM, 60 °C, 24 h.
|
1 |
0.20 |
22 |
0.32 |
n.d.e |
2 |
0.30 |
19 |
0.34 |
1.90 (2.27) |
3 |
0.40 |
27 |
0.46 |
5.29 (2.06) |
4 |
0.50 |
17 |
0.56 |
7.97 (2.04) |
5 |
0.60 |
34 |
0.63 |
8.84 (2.01) |
6 |
0.70 |
23 |
0.74 |
13.4 (1.82) |
7 |
0.80 |
29 |
0.80 |
14.4 (1.84) |
8 |
1 |
36 |
1 |
17.1 (1.84) |
9f |
1 |
97 |
1 |
2.76 (2.46) |
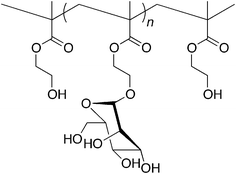 |
| Chart 1 Structure of successive sequence of n ManEMA residues | |
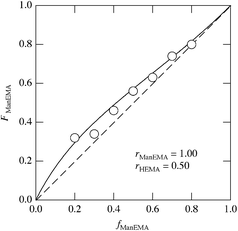 |
| Fig. 3 Composition curve in the radical copolymerization of ManEMA with HEMA in DMF at 60 °C. The solid line is the best fit to the integration form of the Mayo–Lewis equation. | |
Multivalent ligand nature of poly(ManEMA-co-HEMA)
Concanavalin A (ConA) is a well-known lectin having one α-D-mannopyranose recognition domain, and forms a homotetramer at neutral pH. Hence, a multivalent ligand displaying α-D-mannopyranose forms aggregates when mixed with ConA. In order to estimate the kinetics of clustering, we employed a turbidimetric assay in HEPES buffer (pH = 7.4) containing 150 mM NaCl and 1 mM CaCl2 (hereinafter referred to as HBS) according to the reported procedure.40 The concentration of ConA tetramer in HBS was adjusted to be 1 μM. The clustering reaction was initiated by rapid injection of HBS solution of poly(ManEMA-co-HEMA) at 25 °C, and the absorbance at 420 nm was monitored. The final concentration of ManEMA units was adjusted to be 50 μM in all cases. Fig. 4a shows the time courses of the absorbance at 420 nm as a function of reaction time. The absorbance at 420 nm increased quite rapidly in the early stage of reaction (within 60 s) and then increased gradually (for small FManEMA value polymers) or reached a plateau level (for large FManEMA value polymers). The affinity of poly(ManEMA-co-HEMA) for ConA was evaluated in terms of the initial clustering rate (ki/ΔA420s−1). The ki value was evaluated as the slope of the tangent at the early stage (A420 = 0.04), and is plotted as a function of the FManEMA value in Fig. 5. Although the concentration of ManEMA units was constant, the ki values increased with an increase of the FManEMA values of poly(ManEMA-co-HEMA). The chain length effect on the clustering rate45 can be ruled out because no difference in time course of absorbance change was found between two poly(ManEMA)s with the Mn value of 17.1 × 104 (run no. 8) and 2.76 × 104 (run no. 9) as shown in Fig. 4b. This behavior is quite similar to that reported by Kiessling et al.,40 and is known as the cluster glycoside effect.46 Thus, poly(ManEMA-co-HEMA) displays multiple α-D-mannopyranose moieties, and functions as a typical multivalent ligand of ConA.
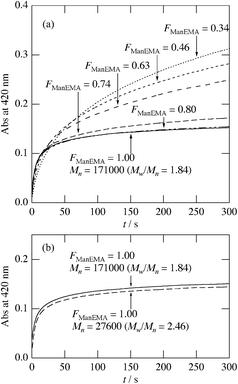 |
| Fig. 4 Time course of optical density at 420 nm in mixtures of concanavalin A and poly(ManEMA-co-HEMA) at 25 °C. The concentration of concanavalin A was 1 μM. The concentration of mannose residue was adjusted to be 50 μM for each poly(ManEMA-co-HEMA). | |
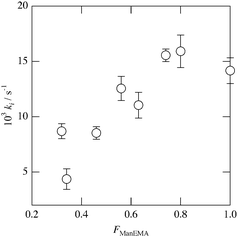 |
| Fig. 5 Initial rate (ki/s−1) of absorbance change as a function of the mole fraction of ManEMA unit in poly(ManEMA-co-HEMA). | |
Toone et al. synthesized dendritic ligands having one, two, three, four and six α-D-mannopyranose moieties, and studied their binding behavior to ConA by means of hemagglutination inhibition, enzyme-linked lectin assay, and isothermal titration microcalorimetry.47,48 They found a significant cluster glycoside effect for tetra- and hexavalent ligands, but not for mono-, di- and trivalent ones. In the case of our glycopolymer, it should be noted that one polymer chain must have a greater number of α-D-mannopyranose moieties than six, even at low FManEMA values. Therefore, the gradual change of the ki values with the FManEMA values suggests that α-D-mannopyranose units which are well separated from each other cannot exert a cluster glycoside effect. This phenomenon can be explained by statistical and/or chelation mechanism of multivalent ligands.49,50 Hence, the distribution of ManEMA units in the polymer chain must be taken into consideration. Assuming the terminal model, the distribution of ManEMA units can be calculated on the basis of the reactivity ratio rManEMA and the feed composition fManEMA. The ratio of the ManEMA units forming a successive sequence of n ManEMA units (Chart 1) to the total ManEMA units (denoted as Xn) can be calculated by means of eqn (1).
in which
P11 is the conditional probability of successive propagation of ManEMA units calculated as follows.
| 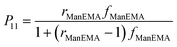 | (2) |
The Xn value represents the probability of ConA binding on a successive sequence of n ManEMA units, if all ManEMA has equal ability to bind to ConA. Fig. 6 shows the calculated Xn value distribution for the fManEMA values of 0.2, 0.4, 0.6 and 0.8. The peak of the Xn distribution shifts gradually to larger n value with increase of the fManEMA value. A very high fManEMA value is required to form successive ManEMA sequences comparable with Toone's dendritic multivalent ligand. This description in terms of successive monomer sequences shows characteristics similar to those seen with low-molecular-weight multivalent ligands.
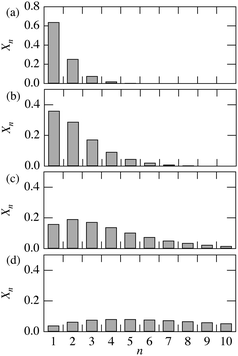 |
| Fig. 6 Calculated fraction of ManEMA units forming successive sequences of n ManEMA residues (Xn) for fManEMA = 0.2 (a), 0.4 (b), 0.6 (c) and 0.8 (d). Xn values are defined in eqn (1). | |
Cellular internalization of fluorescently labeled poly(ManEMA)
In order to explore the biocompatibility of ManEMA, cellular internalization and cytotoxicity of poly(ManEMA) were examined in HeLa cells. In order to visualize cellular internalization of glycopolymer, we synthesized styrene derivatives having fluorescent coumarin moieties, 7-(N-(4-vinylbenzyl)amino)-4-trifluoromethylcoumarin (C151St). Fluorescently labeled poly(ManEMA), poly(ManEMA-co-C151St), was prepared by radical copolymerization of ManEMA with C151St in DMF at 60 °C (Scheme 3). Remaining fluorescent monomer C151St in the crude product was carefully removed by gel filtration on Sephadex G-15 and G-50 columns, and lyophilization of a purified aqueous solution of the polymer afforded a light-green solid. The UV-vis spectrum of the polymer in dimethyl sulfoxide was identical to that of C151St, and the mole fraction of C151St unit was determined to be 5.9 × 10−3 on the basis of the absorbance at 394.5 nm (Fig. 7). Aqueous SEC of the polymer suggested Mn of 2.59 × 104 and Mw/Mn of 2.49. In addition, aqueous SEC with a UV-vis detector afforded essentially the same trace as that recorded with the RI detector, suggesting uniform labeling by C151St. Cellular internalization was examined in HeLa cells (1 × 104cells per well) incubated in the presence of poly(ManEMA-co-C151St) (5 mg per well) for 21 hours at 37 °C, and then co-incubated with LysoTracker Red for an additional 1 hour to stain endo/lysosomal compartments. Fig. 8 shows the fluorescence images of poly(ManEMA) and LysoTracker Red in HeLa cells taken with a confocal laser scanning microscope using an excitation wavelength of 364 and 543 nm, respectively. LysoTracker Red fluorescence showed a clear punctate pattern. Poly(ManEMA-co-C151St) fluorescence showed a more diffuse pattern, but with similar localization to that of LysoTracker Red, suggesting that poly(ManEMA-co-C151St) is taken up via the endocytotic pathway. The function of the mannose moieties of the glycopolymer on the cellular internalization is still unknown. However there is some supporting evidence of mannose-mediated cellular internalization. For example, Kuo et al. reported that the infection of HeLa cells by Chlamydia trachomatis, in which high-mannose type glycoprotein attaches to host cells, is inhibited by addition of mammalian mannose-binding lectin.51 Cytotoxicity of poly(ManEMA-co-C151St) was also evaluated on the basis of NADH dehydrogenase activity of cells after incubation for 24 hours. The cell survival rate was determined to be 95.7 ± 1.7%, compared to the cells with no drug treatment. Therefore, poly(ManEMA-co-C151St) can be taken up by HeLa cells without damage to the cells. Hence, ManEMA appears to be a very promising glycomonomer for use in medicinal applications, such as preparation of synthetic gene carriers. Work along this line is in progress in our laboratory.
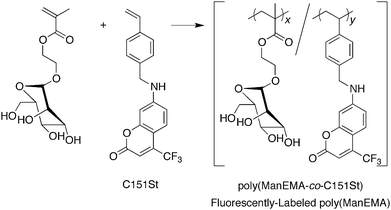 |
| Scheme 3 Preparation of fluorescently labeled poly(ManEMA). Reagents and conditions: AIBN, DMF, N2, 60 °C. | |
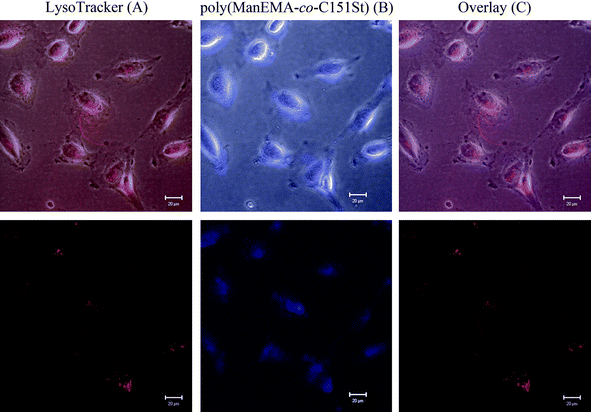 |
| Fig. 8 Confocal microscopic images of HeLa cells after treatment with poly(ManEMA-co-C151St) (5 mg mL−1) and LysoTracker Red. Upper and lower panels are bright-field and fluorescence images, respectively. | |
Conclusions
Stereochemically pure O-α-D-mannopyranosylated 2-hydroxyethyl methacrylate, ManEMA, was successfully prepared in three steps from D-mannose. ManEMA can be isolated as a dry white solid and is much more stable than the O-β-D-glucopyranosylated compound. These characteristics are favorable for development of the polymer chemistry of ManEMA. O-α-D-Mannopyranosylated poly(2-hydroxyethyl methacrylate), poly(HEMA), was prepared by conventional radical copolymerization of ManEMA with HEMA in DMF at 60 °C. The monomer reactivity ratios rManEMA and rHEMA were determined to be 1.00 and 0.50, respectively. The bootstrap effect may account for the higher reactivity of ManEMA as compared with HEMA. The interaction of poly(ManEMA-co-HEMA) with concanavalin A (ConA) was tested by turbidimetric assay. The clustering rate increased with increasing mole fraction of ManEMA units (FManEMA) in poly(ManEMA-co-HEMA), even at a constant concentration of ManEMA unit. This is a well-known phenomenon: the so-called cluster glycoside effect. A terminal model of the polymerization suggested that successive sequences of more than six or seven ManEMA units are essential for the cluster glycoside effect to occur. This is in good agreement with reported findings for dendritic multivalent ligands. Biocompatibility of ManEMA was established by examination of cellular internalization and cytotoxicity of fluorescently labeled poly(ManEMA) in HeLa cells. Confocal laser scanning microscope imaging revealed cellular internalization of poly(ManEMA) via the endocytotic pathway without apparent damage to the cells. Thus, ManEMA is a promising glycomonomer for medicinal application.
Acknowledgements
We thank Mr Shohei Katao at NAIST for X-ray crystallography. This work was supported by a Grant-in-Aid for Scientific Research (#21550156) and Kyoto-Nanotechnology Network of the Ministry of Education, Culture, Sport, Science and Technology (MEXT) of the Japanese Government.
Notes and references
- A. Helenius and M. Aebi, Science, 2001, 291, 2364–2369 CrossRef CAS.
- D. Vestweber and J. E. Blanks, Physiol. Rev., 1999, 79, 181–213 CAS.
- L. D. Powell and A. Varki, J. Biol. Chem., 1995, 270, 14243–14246 CrossRef CAS.
- A. Narumi and T. Kakuchi, Polym. J., 2008, 40, 383–397 CrossRef CAS.
- S. G. Spain, M. I. Gibson and N. R. Cameron, J. Polym. Sci., Part A: Polym. Chem., 2007, 45, 2059–2072 CrossRef CAS.
- Y. Miura, J. Polym. Sci., Part A: Polym. Chem., 2007, 45, 5031–5036 CrossRef CAS.
- T. M. Reineke, J. Polym. Sci., Part A: Polym. Chem., 2006, 44, 6895–6908 CrossRef CAS.
- V. Ladmiral, E. Melia and D. M. Haddleton, Eur. Polym. J., 2004, 40, 431–449 CrossRef CAS.
- Y. Miura, K. Yasuda, K. Yamamoto, M. Koike, Y. Nishida and K. Kobayashi, Biomacromolecules, 2007, 8, 2129–2134 CrossRef CAS.
- M. Ahmed, Z. Deng and R. Narain, ACS Appl. Mater. Interfaces, 2009, 1, 1980–1987 CrossRef CAS.
- K. I. P. J. Hidari, T. Murata, K. Yoshida, Y. Takahashi, Y. Minamijima, Y. Miwa, S. Adachi, M. Ogata, T. Usui, Y. Suzuki and T. Suzuki, Glycobiology, 2008, 18, 779–788 CrossRef CAS.
- K. Merrett, W. Liu, D. Mitra, K. D. Camm, C. R. McLaughlin, Y. Liu, M. A. Watsky, F. Li, M. Griffith and D. E. Fogg, Biomaterials, 2009, 30, 5403–5408 CrossRef CAS.
- M. L. López Donaire, J. Parra-Cáceres, B. Vázquez-Lasa, I. García-Álvarez, A. Fernández-Mayoralas, A. López-Bravo and J. San Román, Biomaterials, 2009, 30, 1613–1626 CrossRef CAS.
- X. Wang, X. Geng, L. Ye, A. Zhang and Z. Feng, React. Funct. Polym., 2009, 69, 857–863 CrossRef CAS.
- I. Tranoudis and N. Efron, Contact Lens Anterior Eye, 2004, 27, 193–208 Search PubMed.
- A. W. Lloyd, R. G. A. Faragher and S. P. Denyer, Biomaterials, 2001, 22, 269–785 CrossRef.
- H.-N. Lim, B. Yu, J. I. Lim, J.-S. Ahn and Y.-K. Lee, Mater. Des., 2009, 30, 3996–4002 CrossRef CAS.
- D. Horák, J. Kroupová, M. Šlouf and P. Dvořák, Biomaterials, 2004, 25, 5249–5260 CrossRef CAS.
- J. S. Belkas, C. A. Munro, M. S. Shoichet, M. Johnston and R. Midha, Biomaterials, 2005, 26, 1741–1749 CrossRef CAS.
- H. Kitano, Y. Takahashi, K. Mizukami and K. Matsuura, Colloids Surf., B, 2009, 70, 91–97 CrossRef CAS.
- S. R. S. Ting, E. H. Min, P. Escalé, M. Save, L. Billon and M. H. Stenzel, Macromolecules, 2009, 42, 9422–9434 CrossRef CAS.
- X. Wang, X. Geng, L. Ye, A.-Y. Zhang and Z.-G. Feng, React. Funct. Polym., 2009, 69, 857–863 CrossRef CAS.
- V. Vázquez-Dorbatt, Z. P. Tolstyka, C.-W. Chang and H. D. Maynard, Biomacromolecules, 2009, 10, 2207–2212 CrossRef CAS.
- R. M. Broyer, G. M. Quaker and H. D. Maynard, J. Am. Chem. Soc., 2008, 130, 1041–1047 CrossRef CAS.
- L. Bech, B. Lepoittevin, A. E. Achhab, E. Lepleux, L. Teulé-Gay, C. Boisse-Laporte and P. Roger, Langmuir, 2007, 23, 10348–10352 CrossRef CAS.
- V. Vázquez-Dorbatt and H. D. Maynard, Biomacromolecules, 2006, 7, 2297–2302 CrossRef CAS.
- T.-Y. Guo, P. Liu, J.-W. Zhu, M.-D. Song and B.-H. Zhang, Biomacromolecules, 2006, 7, 1196–1202 CrossRef CAS.
- S. G. Spain, L. Albertin and N. R. Cameron, Chem. Commun., 2006, 4198–4200 RSC.
- S. S. Gupta, K. S. Raja, E. Kaltgrad, E. Strable and M. G. Finn, Chem. Commun., 2005, 4315–4317 RSC.
- M. Ambrosi, A. S. Batsanov, N. R. Cameron, B. G. Davis, J. A. K. Howard and R. Hunter, J. Chem. Soc., Perkin Trans. 1, 2002, 45–52 RSC.
- T. Serizawa, S. Yasunaga and M. Akashi, Biomacromolecules, 2001, 2, 469–475 CrossRef CAS.
- H. Kitano, Y. Ishino and K. Yabe, Langmuir, 2001, 17, 2312–2316 CrossRef CAS.
- R. Nishida, T. Ishikawa and S. Saito, Chem. Lett., 2001, 394–395 CrossRef CAS.
- S. Kitazawa, M. Okumura, K. Kinomura and T. Sakakibara, Chem. Lett., 1990, 1733–1736 CAS.
- F. P. Schwarz, K. Puri and A. Surolia, J. Biol. Chem., 1991, 266, 24344–24350 CAS.
- S. S. Diebold, C. Plank, M. Cotton, E. Wagner and M. Zenke, Somatic Cell Mol. Genet., 2002, 27, 65–74 Search PubMed.
- L. Dong, S. Xia, Y. Luo, H. Diao, J. Zhang, J. Chen and J. Zhang, J. Controlled Release, 2009, 134, 214–220 CrossRef CAS.
- J. A. Watt and S. J. Williams, Org. Biomol. Chem., 2005, 3, 1982–1992 RSC.
- A. Altomare, G. Cascarano, C. Giacovazzo, A. Guagliardi, M. Burla, G. Polidori and M. Camalli, J. Appl. Crystallogr., 1994, 27, 435 CrossRef.
- C. W. Cairo, J. E. Gestwicki, M. Kanai and L. L. Kiessling, J. Am. Chem. Soc., 2002, 124, 1615–1619 CrossRef.
- The vinyl carbon (C11) and the methyl carbon (C12) are present in a mixture of s-cis and s-trans conformations, so the precise structure of the 2-isopropenyl group cannot be reliably determined.
- F. R. Mayo and F. M. Lewis, J. Am. Chem. Soc., 1944, 66, 1594–1601 CrossRef CAS.
- H. J. Harwood, Makromol. Chem., Macromol. Symp., 1987, 10/11, 331–354 Search PubMed.
- C. Fernández-Monreal, G. Martínez, M. Sánchez-Chaves and E. L. Madruga, J. Polym. Sci., Part A: Polym. Chem., 2001, 39, 2043–2048 CrossRef CAS.
- M. Kanai, K. H. Mortell and L. L. Kiessling, J. Am. Chem. Soc., 1997, 119, 9931–9932 CrossRef CAS.
- J. J. Lundquist and E. J. Toone, Chem. Rev., 2002, 102, 555–578 CrossRef CAS.
- J. B. Corbell, J. J. Lundquist and E. J. Toone, Tetrahedron:
Asymmetry, 2000, 11, 95–111 CrossRef CAS.
- S. M. Dimick, S. C. Powell, S. A. McMahon, D. N. Moothoo, J. H. Naismith and E. J. Toone, J. Am. Chem. Soc., 1999, 121, 10286–10296 CrossRef CAS.
- J. E. Gestwicki, C. W. Cairo, L. E. Strong, K. A. Oetjen and L. L. Kiessling, J. Am. Chem. Soc., 2002, 124, 14922–14933 CrossRef CAS.
- L. L. Kiessling, J. E. Gestwicki and L. E. Strong, Curr. Opin. Chem. Biol., 2000, 4, 696–703 CrossRef CAS.
- A. F. Swanson, R. A. B. Ezekowitz, A. Lee and C.-C. Kuo, Infect. Immun., 1998, 66, 1607–1612 CAS.
|
This journal is © The Royal Society of Chemistry 2011 |
Click here to see how this site uses Cookies. View our privacy policy here.