DOI:
10.1039/C0PY00320D
(Paper)
Polym. Chem., 2011,
2, 970-974
Colloidal silver deposition onto functionalized polystyrene microspheres
Received
27th September 2010
, Accepted 4th January 2011
First published on 3rd February 2011
Abstract
This paper presents a facile method for the preparation of silver/polystyrene (Ag/PS) composite microspheres. PS microspheres with carboxyl and nitrile groups on the surfaces were synthesized via a two-step dispersion copolymerization of styrene, itaconic acid, and acrylonitrile in ethanol–water media. Ag/PS composite microspheres were prepared successively by addition of AgNO3 aqueous solution to the dispersion, absorbing to the surfaces of functional PS microspheres, and then reduction of Ag+ ions to silver nanoparticles by aqueous hydrazine hydrate. The results showed that Ag nanoparticles with size of about 50 nm were located on the shell of PS microspheres due to the combined interactions between the carboxyl and nitrile groups of PS microspheres and the in situ formed silver nanoparticles. The as-prepared Ag/PS microspheres showed good catalytic properties.
Introduction
In recent years, Ag/polymer composites have attracted much interest in view of their practical applications or potential applications in the fields of sensors,1 optical,2,3 and catalysis.4,5Polystyrene (PS) as a kind of inert support has widely been used to prepare nanocomposite microspheres. There exit several methods for the deposition of colloidal metal particles onto the surface of functionalized PS microspheres, such as simple adsorption of preformed colloids,6–8in situ metal reduction and electrostatic deposition,9–12 and layer-by-layer (LbL) assembly.13 Among these methods, electrostatic deposition has been proved to be an effective method to attaching metal nanoparticles onto polymer microspheres.
However, electrostatic repulsion between the nanoparticles and the absence of affinity between nanoparticles and polymer microspheres has proven to be the major difficulties in producing Ag/polymer composites. In order to improve the affinity between Ag nanoparticles and polymer microspheres, various functional groups such as carboxyl, amine, hydroxy, nitrile, sulfate, and thiol groups, have been used to improve the interactions between nanoparticles and polymer microspheres.8–12 Kim et al.8 synthesized silver/polymer colloidal composites from surface-functional porous polymer microspheres by taking advantage of the interactions between nitrile group and Ag nanoparticles. Akash et al.9 fabricated Ag/polymer composite microspheres from poly(N-isopropylacrylamide)-coated PS microspheres by taking advantage of the interactions between the amide groups of poly(N-isopropylacrylamide) and silver particles. Attractive interactions among carboxylic acid groups and silver cations have been widely used to the adsorption of Ag+ onto functional microspheres and the in situ formation of silver particles to prepare silver/polymer composite microspheres.10 Owing to the electrostatic interaction between Ag+ and carboxyl groups on polymer substrate, Cheng et al.10 fabricated Ag/polymer composite microspheres. To the best of our knowledge, functional PS microspheres with carboxylic acid and nitrile groups on the surfaces have not been used as polymer substrates to fabricate Ag/polymer composite microspheres. Inspired by the above interesting and valuable results, we investigated the feasibility of preparation of functional PS microspheres with carboxyl and nitrile groups on the surfaces, and further anchoring Ag nanoparticles onto the shell of final composite microspheres by their affinity interaction with carboxyl and nitrile groups.
Herein, we report the synthesis of functional PS composite microspheres via a two-step copolymerization of styrene, acrylonitrile, and itaconic acid. Then Ag/PS composite microspheres were obtained by taking full advantage of the carboxyl–Ag+ electrostatic adsorption and nitrile–silver nanoparticles affinity effects. The catalytic properties were studied as well.
Experimental
Raw materials
Styrene (St) and acrylonitrile (AN) were purified by distillation under reduced pressure before use. 2,2′-Azobis(isobutyronitrile) (AIBN) was purified by recrystallization in ethanol and kept refrigerated until use. Silver nitrate (AgNO3, 99.5%), polyvinylpyrrolidone (PVP, MW ≈ 30,000), itaconic acid (ITA), and hydrazine hydrate (85 wt%) were purchased in their reagent and used as received. NaBH4 and methylene blue (MB) were used as received. Other reagents of analytical grade were utilized without further purification. All reagents were purchased from Shanghai Chem. Reagent Co. (China). Deionized water was used for all experiments.
Preparation of Ag/PS composite microspheres
PS microspheres with carboxyl and nitrile groups on the surfaces were synthesized via a two-step dispersion polymerization in ethanol–water media using PVP as dispersant. The PS microspheres were prepared with all the ingredients kept unchanged but the variation of ITA as shown in Table 1, and were used as template particles to prepare Ag/PS composite microspheres. Briefly, 5.0 g of St, 0.10 g of AIBN, 2.0 g of PVP, 0.05–0.20 g of ITA, 4 g of water, and 76 g of ethanol were charged into a 250 mL four-necked vessel. The reaction system was deoxygenated by bubbling with nitrogen gas for about 30 min at room temperature, and the polymerization took place at 75 °C. Carboxyl modified PS microspheres were obtained after the polymerization was carried out for 3.5 h (Scheme 1a). The mixtures of St/AN (5.0/5.0, g/g) were added dropwise within 1 h and then the reaction was carried out for another 6.0 h to obtain PS microspheres with carboxyl and nitrile groups on the surfaces (Scheme 1b). The deposition of Ag nanoparticles onto functional PS microspheres was carried out by the reduction of AgNO3. After the addition of AgNO3 solution at room temperature (Scheme 1c), Ag+ ions were absorbed on the shells due to the affinity interaction with carboxyl and nitrile group. Hydrazine solution (22 wt%, 10.0 g) was dropped slowly into the above aqueous dispersion under vigorous stirring. The in situreduction reaction of Ag+ was carried out for 2 h to yield Ag/PS microsphere dispersion with a slightly dark yellow appearance (Scheme 1d).
Table 1 Synthetic conditions of Ag/PS composite microspheres
Entry |
PS microsphere |
Functional PS microspheres |
Ag/PS composite microspheres |
St/g |
ITA/g |
AIBN/g |
PVP/g |
H2O/g |
Ethanol/g |
St/g |
AN/g |
AgNO3/g |
Hydrazine/g |
One-step dispersion copolymerization of St, ITA, and AN.
|
1 |
5.0 |
0.05 |
0.10 |
2.0 |
4.0 |
76.0 |
5.0 |
5.0 |
0.3 |
10.0 |
2 |
5.0 |
0.10 |
0.10 |
2.0 |
4.0 |
76.0 |
5.0 |
5.0 |
0.3 |
10.0 |
3 |
5.0 |
0.15 |
0.10 |
2.0 |
4.0 |
76.0 |
5.0 |
5.0 |
0.3 |
10.0 |
4 |
5.0 |
0.20 |
0.10 |
2.0 |
4.0 |
76.0 |
5.0 |
5.0 |
0.3 |
10.0 |
5a |
5.0 |
0.20 |
0.10 |
2.0 |
4.0 |
76.0 |
— |
5.0 |
— |
— |
6 |
5.0 |
0.20 |
0.10 |
2.0 |
4.0 |
76.0 |
5.0 |
— |
0.3 |
10.0 |
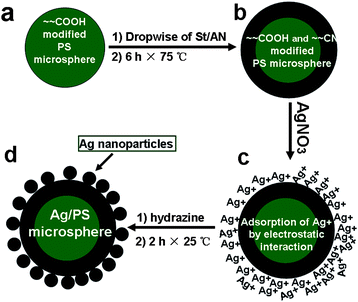 |
| Scheme 1 Schematic illustration for the preparation of Ag/PS microspheres using a two-step dispersion copolymerization. (a) Carboxyl modified PS microspheres were prepared with St and ITA as monomers. (b) Carboxyl and nitrile groups modified PS microspheres were formed after St and acrylonitrile were added dropwise. (c) Ag+ was adsorbed on the shells after the addition of AgNO3 solution because of their electrostatic interactions with carboxyl groups. (d) Ag nanoparticles were obtained by in situreduction of Ag+ ions at room temperature and Ag/PS microspheres were fabricated by the assistance of electrostatic interactions between Ag and nitrile groups. | |
Catalytic properties of Ag/PS microspheres
To investigate the catalytic properties of the Ag/PS composite microspheres, 5 mL of as-prepared Ag/PS suspension was purified by centrifugation three times and redispersed in 200 mL water. 10 mL dye MB solution was added in the above aqueous suspension and then injected with 1 mL of NaBH4 solution under stirring. The catalytic properties of Ag/PS composite microspheres were investigated by monitoring the variation in optical absorption of the dye with a UV-vis spectrometer.
Characterization
Samples for FT-IR characterization were dried at 60 °C under vacuum for 24 h, dispersed in KBr matrices, and measured in the wavenumber range from 4000 to 400 cm−1 at a resolution of 4 cm−1 using a Nicolet Nexus-870 FT-IR spectrophotometer. Samples for transmission electron microscopy (TEM) were prepared by drying a drop of the dilute dispersion of composite microspheres onto a carbon-coated copper grid. Analysis was conducted using a Hitachi H-800 electron microscope operating at 100 kV. UV-vis transmittance spectra were recorded over the range 200–1000 nm on a Shimadzu U-3101PC spectrophotometer, equipped with a custom-built sample holder for angle-resolved UV-vis transmittance measurements. The average particle size and size distribution of Ag nanoparticles were determined using light scattering equipment (Zetasizer 3000 HSA, Malvern Company). UV-vis transmittance spectra were recorded over the range 200–1000 nm on a Shimadzu U–3101PC spectrophotometer, equipped with a custom-built sample holder for angle-resolved UV-vis transmittance measurements in aqueous media. The powder X-ray diffraction (XRD) was performed on Rigaku Geigerflex diffractometer with Cu-Kα radiation (λ = 0.15418 nm), and the 2θ angle varied from 16° to 80° at a scanning rate of 1°/min.
Results and discussion
Synthesis of functional PS and Ag/PS composite microspheres
Itaconic acid (ITA) and acrylonitrile (AN) were used as functional monomers to prepare functional PS microspheres viadispersion polymerization. Traditional one-step dispersion polymerization often leads to particles with very narrow size distribution. However, dispersion polymerization was actually highly sensitive to small changes in the reaction system even when only a small amount of a second monomer is added.14–16 Yang et al.17 studied the dispersion copolymerization of styrene with acrylic acid (AA). They found that with the increasing amount of AA in the monomer mixture, the microsphere size increased and the microsphere size distribution became broader. Winnik and co-workers18–20 successfully prepared different functional monodispersions by delaying the addition of various comonomers to the reaction for dispersion copolymerization of styrene, and they called their methodology as “two-stage” dispersion polymerization. To confirm the important role of two-step dispersion polymerization on the dispersity of final PS composite microspheres, we have tried the one-step synthesis of functional microspheres as a control experiment. The other components including AN were kept unchanged but using a one-step dispersion copolymerization process as shown in Entry 5 of Table 1. Compared with that of Entry 4 in Table 1, the size of the resulting PS microspheres prepared from Entry 5 is about 1.0 μm and the obtained microspheres were polydisperse (by TEM observation). So we adopted the two-stage dispersion polymerization method by delaying the addition of AN monomers.
Structure of Ag/PS composite microspheres
After the copolymerization of ITA and styrene in the first stage, the carboxylic acid groups were mostly located on the surface of PS microspheres because of their hydrophilic nature. Monomers of St and AN were added dropwise in the second stage of polymerization, the hydrophobic St and AN tended to diffuse into seeded functional PS microspheres and polymerized. The carboxylic acid may be buried in the interior, located on the surface, or remain in the serum phase, which can be distinguished by conductometric titration.21 The amount of carboxyl groups was determined by conductometric titration by the aqueous solution of NaOH. Quantitative information of the carboxyl contents was acquired from the titration plots.21 The results showed that with the increase of ITA amount from 0.05, 0.10, 0.15, to 0.20 g, the carboxyl contents on the surface of microspheres increased with nearly the same proportion, i.e., from 0.109, 0.202, 0.311, up to 0.362 mmol g−1.
The incorporation of nitrile groups onto the surfaces of PS microspheres was confirmed by FT-IR spectroscopy measurements. Curves 1, 2, and 3 in Fig. 1a show FT-IR spectra of PS, functional PS, and Ag/PS microspheres, respectively. Compared with the IR spectrum of PS microspheres in curve 1, the presence of typical peak of nitrile groups (2237 cm−1) in curve 2 of functional PS microspheres confirmed the copolymerization of St and AN. The spectral features of the silvered microspheres (curve 3) were overall very similar to those of unsilvered ones (curve 2). The partial enlarged view of Fig. 1a is shown in Fig. 1b, which gives more detailed information. The shape of the amide carbonyl stretch absorption of PVP is centered at 1662 cm−1 for un-silvered PS microspheres (curves 1 and 2) and shifted to 1672 cm−1 (curve 3) for silvered ones.22 The increase in intensity of the N–H stretching vibration band (near 3434 cm−1) and the N–H bending vibration band (1672 cm−1) appeared in the spectrum of the silvered microspheres as shown in Fig. 1b, which was due to the interactions between the metal atoms and nitrogen functions of the polymer support.9,22 Therefore, we can confirm the presence of silver nanoparticles in the composite microsphere.
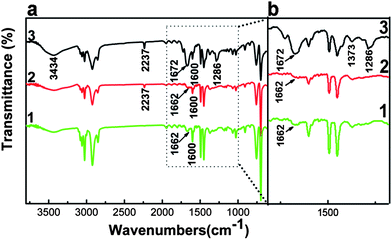 |
| Fig. 1 (a) Typical IR spectra of PS (1), functional PS (2), and Ag/PS composite microspheres (3); (b) a partial enlarged view of (a). | |
Morphology of composite microspheres
The dispersion polymerization is an attractive method for producing micron-size polymer microspheres in a single batch process.23 Traditional one-step dispersion polymerization often leads to microspheres with very narrow size distribution. However, dispersion polymerization was highly sensitive to small changes in the reaction system when even only small amount of functional monomer is added.15,17 We have tried the one-step synthesis of functional microspheres and the size distribution of the obtained microsphere became broader. Fig. 2a and b shows the TEM images of the functional PS microspheres and Ag/PS composite microspheres, respectively. The functional PS microspheres are spherical in shape and nearly monodisperse in size with a diameter of about 1.0 μm (Fig. 2a). After the addition of monomers of St and AN in the second step of dispersion copolymerization, the functional microspheres had an increased average size of 1.7 μm with lower monodispersity. The as-prepared PS particles were negatively charged due to the functional comonomer, ITA. The positively charged Ag+ ions were attracted onto the surface of PS particles by electrostatic interaction at first, and then the Ag+ ions were in situ reduced to zerovalent sliver nanoparticles by hydrazine. Due to the affinity effects of carboxyl groups, Ag nanoparticles grew and were located on the shell of functional PS microspheres. Furthermore, the polar head groups (nitrile groups) on the surface of the polymer microspheres had a high affinity for metal nanoparticles,which provided preferentially sufficient deposition sites for Ag particles.8,24,25 Controlled experiments without the addition of acrylonitrile were carried out to confirm the effects of nitrile groups as shown in Entry 6 of Table 1. The results showed that Ag particles were located not only on the surfaces of functional PS microspheres, but isolated from surfaces of polymer microspheres and existed as free particles in the aqueous medium. Without adding acrylonitrile, some Ag+ ions nucleated in the aqueous medium and grew into free triangular nanoplates. Due to the combined interactions of carboxyl and nitrile groups, Ag/polymer microspheres were obtained successfully with silver particles of about 50 nm being immobilized on the shell of PS microspheres as shown in Fig. 3. The result is in good agreement with conventional observations.26,27
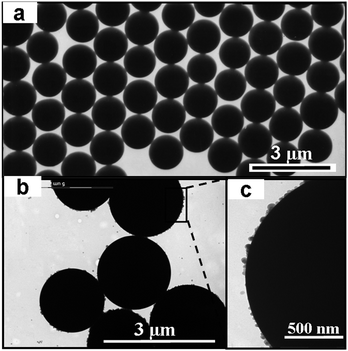 |
| Fig. 2
TEM images of typical functional PS microspheres (a), Ag/PS composite microspheres (b), and a magnified TEM image (c) showing the shell details of Ag/PS composite microspheres. | |
An obvious silver plasmon absorption band at ca. 414 nm28 was observed for the dispersion of silvered composite microspheres (Fig. 4b). However, the supernatant obtained from the dialyzed microsphere suspension was colorless and showed no absorption band at ca. 414 nm (Fig. 4a). These results indicate that all the formed Ag nanoparticles were adsorbed on the surfaces of PS microspheres.
XRD patterns of Ag/PS composite microspheres
The typical XRD patterns of the as-prepared composite microspheres were shown in Fig. 5. The exhibited peaks at 2θ angles of 38.3°, 44.4°, 66.4°, 77.7°, and 81.6° corresponding to the reflections of (111), (200), (220), (311), and (222) crystal plane of the fcc structure of Ag (JCPDS No. 04–0783), respectively. It confirmed that Ag nanoparticles with crystalinity could be obtained successfully by reducing AgNO3 using hydrazine hydrate as reducing agent.
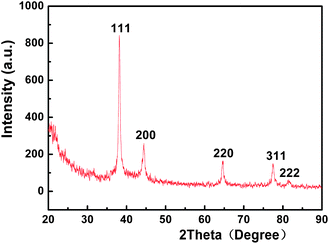 |
| Fig. 5
XRD patterns of Ag/PS composite microspheres. | |
Catalytic properties of Ag/PS composite microspheres
The Ag/polymer composite microspheres have been used as catalysts10,29 and antibacterial materials because of easy recycling and access to the active site of the noble metal particles.30,31Ag nanoparticles can serve as an electron relay in the oxidant–reductant system, which can find use in catalytic reducing of methylene blue by NaBH4.10
Fig. 6 shows the catalytic properties of Ag/PS composite microspheres under ambient conditions. The characteristic peaks of MB are located at 665 nm and 613 nm. With the redox reaction proceeding, the concentration of MB can be monitored by the absorbance maximum (λmax) by using UV-vis spectrometer. As curve g in Fig. 6 displayed, the reduction of MB with NaBH4 finished after 30 min. It should be noted that without the addition of Ag/PS microspheres, the color of NaBH4 and MB solution remained unchanged. After the addition of Ag/PS microspheres, the color of mixture became pale gradually and vanished eventually. The nucleophile NaBH4 can donate electrons to silver particles, and the electrophile dyes would capture electrons from silver nanoparticles. Thus the silver nanoparticles acted as an electron relay for catalytic reducing of MB by NaBH4.32,33
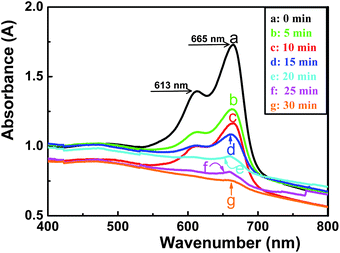 |
| Fig. 6
UV-vis spectra of MB reduced by NaBH4 and a catalyst of Ag/PS as functions of reaction time. (a)–(g) corresponds to MB solution with Ag/PS microspheres for 0, 5, 10, 15, 20, 25, and 30 min, respectively. | |
Conclusions
In summary, surface-functionalized PS microspheres were synthesized by a two-step dispersion copolymerization of styrene, itaconic acid, and acrylonitrile. Ag/PS composite microspheres were obtained by taking full advantage of adsorption interactions between carboxyl and nitrile groups of PS microspheres and the in situ formed Ag nanoparticles. It was found that the surface characteristics of the supporting microspheres played an important role in the deposition of Ag nanoparticles on the composite microspheres and Ag/PS microspheres showed the catalytic abilities in the oxidation-reduction reaction of methylene blue by NaBH4.
Acknowledgements
This work was supported by the National Natural Science Foundation of China (No. 50573070, 21044006, 50773073, and 51073146) and the program for Changjiang Scholars and Innovative Research Team at the University of Science and Technology of China.
Notes and references
- A. Choudhury, Sens. Actuators, B, 2009, 138, 318 CrossRef.
- R. Zeng, M. Z. Rong, M. Q. Zhang, H. C. Liang and H. M. Zeng, Appl. Surf. Sci., 2002, 187, 239 CrossRef CAS.
- A. Sezer, U. Gurudas, B. Collins, A. Mckinlay and D. B. Bubb, Chem. Phys. Lett., 2009, 477, 164 CrossRef CAS.
- S. Jana, S. Pande, S. Panigrahi, S. Praharaj, S. Basu, A. Pal and T. Pal, Langmuir, 2006, 22, 7091 CrossRef CAS.
- S. Jana, S. K. Ghosh, S. Nath, S. Pande, S. Praharaj, S. Panigrahi, S. Basu, T. Endo and T. Pal, Appl. Catal., A, 2006, 313, 41 CrossRef CAS.
- W. Zhao, Q. Y. Zhang, J. P. Zhang and H. P. Zhang, Polym. Compos., 2009, 30, 891 Search PubMed.
- J. H. Lee, M. A. Mahmoud, V. Sitterle, J. Sitterle and J. C. Meredith, J. Am. Chem. Soc., 2009, 131, 5048 CrossRef CAS.
- J. W. Kim, J. E. Lee, S. J. Kim, J. S. Lee, J. H. Ryu, J. Kim, S. H. Han, I. S. Chang and K. D. Suh, Polymer, 2004, 45, 4741 CrossRef CAS.
- C. W. Chen, M. Q. Chen, T. Serizawa and M. Akash, Adv. Mater., 1998, 10, 1122 CrossRef CAS.
- X. J. Cheng, S. C. Tjong, Q. Zhao and R. K. Y. Li, J. Polym. Sci., Part A: Polym. Chem., 2009, 47, 4547 CrossRef CAS.
- J. W. Kim, J. E. Lee, J. H. Ryu, J. S. Lee, S. J. Kim, S. H. Han, I. S. Chang, H. H. Kang and K. D. Suh, J. Polym. Sci., Part A: Polym. Chem., 2004, 39, 2551 CrossRef.
- L. C. Santa de Maria, J. D. C. Souza, M. R. M. P. Aguiar, S. H. Wang, J. L. Mazzei, I. Felzenszwalb and S. C. Amico, J. Appl. Polym. Sci., 2008, 107, 1879 CrossRef.
- A. G. Dong, Y. J. Wang, Y. Tang, N. Ren, W. L. Yang and Z. Gao, Chem. Commun., 2002,(4), 350 RSC.
- P. Li, J. Xu, Q. Wang and C. Wu, Langmuir, 2000, 16, 4141 CrossRef CAS.
- C. K. Ober and K. P. Lok, Polym. Sci., 1990, 35, 87 Search PubMed.
- D. Horák, J. Polym. Sci., Part A: Polym. Chem., 1995, 33, 2329 CrossRef CAS.
- W. L. Yang, D. Yang, J. H. Hu, C. C. Wang and S. K. Fu, J. Polym. Sci., Part A: Polym. Chem., 2001, 39, 555 CrossRef CAS.
- J. S. Song, F. Tronc and M. A. Winnik, Polymer, 2006, 39, 8318 CAS.
- J. S. Song and M. A. Winnik, Macromolecules, 2005, 38, 8300 CrossRef CAS.
- J. S. Song, L. Chagal and M. A. Winnik, Macromolecules, 2006, 39, 5729 CrossRef CAS.
- A. Slistan-Grijalva, R. Herrera-Urbina, J. F. Rivas-Silva, M. Ávalos-Borja, F. F. Castillón-Barraza and A. Posada-Amarillas, Mater. Res. Bull., 2008, 43, 90 CrossRef CAS.
- K. Zhang, Q. Fu, J. H. Fan and D. H. Zhou, Mater. Lett., 2005, 59, 3682 CrossRef CAS.
- S. Kawaguchi and K. Ito, Adv. Polym. Sci., 2005, 175, 299 CAS.
- A. Dokoutchaev, J. T. James, S. C. Koene, S. Pathak, G. K. Surya and M. E. Thompson, Chem. Mater., 1999, 11, 2389 CrossRef CAS.
- T. Teranish, I. Kiyokawa and M. Miyake, Adv. Mater., 1998, 10, 596 CrossRef CAS.
- R. Griffith Freeman, K. C. Grabar, K. J. Allison, R. M. Bright, J. A. Davis, A. P. Guthrie, M. B. Hommer, M. A. Jackson, P. C. Smith, D. G. Walter and M. J. Natan, Science, 1995, 267, 1929.
- K. C. Grabar, R. Griffith Freeman, M. B. Hommer and M. J. Natan, Anal. Chem., 1995, 67, 735 CrossRef CAS.
- T. Ung, M. Giersig, D. Dunstan and P. Mulvaney, Langmuir, 1997, 13, 1773 CrossRef CAS.
- D. Lee, R. E. Cohen and M. F. Rubner, Langmuir, 2005, 21, 9651 CrossRef CAS.
- F. Wen, W. Q. Zhang, G. W. Wei, Y. Wang, J. Z. Zhang, M. C. Zhang and L. Q. Shi, Chem. Mater., 2008, 20, 2144 CrossRef CAS.
- Y. Lu, Y. Mei, M. Schrinner, M. Ballauff, M. W. MÖller and M. W. Breu, J. Phys. Chem. C, 2007, 111, 7676 CrossRef CAS.
- N. R. Jana, T. K. Sau and T. Pal, J. Phys. Chem. B, 1999, 103, 115 CrossRef.
- N. R. Jana and T. Pal, Langmuir, 1999, 15, 3458 CrossRef CAS.
|
This journal is © The Royal Society of Chemistry 2011 |
Click here to see how this site uses Cookies. View our privacy policy here.