Dye-loaded zeolite L @silica core-shell composite functionalized with europium(III) complexes for dipicolinic acid detection†
Received
7th September 2010
, Accepted 25th October 2010
First published on 12th November 2010
Abstract
Novel core-shell composites have been developed by immobilization of non-luminescent europium(III) complexes onto the surface of silica shells that are coated on the surface of luminescent dye-loaded zeolite L nano-crystals. The obtained core-shell composites were used for the ratiometric detection of dipicolinic acid (DPA) molecules. The dyes located in the channels of the zeolite L host are protected from any interaction with the environment of the particles and therefore provide a stable reference signal which can eliminate the need for instrument-specific calibration curves for DPA quantification in an analyte.
Introduction
Coating nanostructures with shells represents probably the simplest and most versatile strategy for increasing the diversity and complexity of a nanostructure system.1Silica has long been used as the coating for a wide variety of nanostructures such as silica-coated superparamagnetic metal oxides,1bquantum dots,1cgold,1dcarbon nanotubes,1e organic polymers,1f and MOFs1g to improve their technological application in catalysis, photonics, electronics, optics, and biomedicine. Various approaches including sol–gel and microemulsion methods have been reported in the past.2–4 The silica shell coated on nanostructure surfaces endowed the nanocomposites several advantages, for example it can enhance the stability and bio-compatibility of the core particles, and can allow modification of the composite particlesvia the co-condensation of siloxy-derived molecules.1g
Zeolite L is a crystalline porous material of one-dimensional channels arranged in a hexagonal structure. The channels have a smallest diameter of about 0.71 nm, the largest internal diameter is about 1.26 nm. The distance between the centers of two neighbouring channels is 1.84 nm. Their one-dimensional channels can be filled with suitable guests such as dyes, lanthanide complexes, and metal clusters.5 Geometrical constraints imposed by the host structure lead to supramolecular organization of the guests in the channels. The rigid framework of zeolite can prevent the molecules from chemical attack, photobleaching or thermal decomposition.5c,6 Nevertheless, leakage of guest molecules is often observed when the system is used and handled in a solution where the guest molecules can be soluble.5 Coating dye-loaded zeolite L with a silica shell through a combination of polyelectrolyte layer-by-layer technique and TEOS hydrolysis and condensation can prevent the leakage of the dyes from the channels.7 In addition, bright, luminescent, multicolour crystals with simultaneous isotropic and anisotropic intense luminescence can be observed if dyes were encapsulated into the silica shells.
We have recently reported the coating of a silica shell on lanthanide complex-loaded zeolite L to obtain a novel luminescent core-shell composite via the same method. The core-shell composite was further functionalized with terbium(III) complexes for the detection of dipicolinic acid (DPA).8Terbium(III) has been thought of as an ideal lanthanide ion to detect DPA due to its bright luminescence, long decay time and high enhancement ratio.9,10Nevertheless, the strong interference from the second-order scattering peak at about 540 nm (excitation with a 270 wavelength) makes it difficult to directly observe the change in the maximum terbium emission intensity at 544 nm.11 278 nm is therefore often used to excite the sensor to decrease the interference, which hence lowers the sensitivity of terbium-based sensor towards DPA molecules.1g,10cEuropium(III)-based sensors can overcome such drawback. In addition, europium(III) has several other advantages including larger Stoke shift and a red emission. Lu et al. recently report the detection of DPA with europium(III) complexes immobilized on silica particles.11
Herein, we describe a new core-shell composite with a luminescent dye-loaded zeolite L nano-crystal core and a silica shell with europium(III) complexes immobilized for luminescence detection of dipicolinic acid (DPA). The advantages of using zeolite L instead of silica as the core are:12 (1) zeolite L is crystalline rather than amorphous, (2) the size of the zeolite L crystals can be easily tuned in the size range of 30 nm up to about 10
000 nm, and (3) it can prevent the self-aggregation of dyes and superimpose a specific organization (e.g., the anisotropic intense luminescence) with a high concentration of monomers.
Results and discussion
Zeolite L nano-crystals with a size of about 30 nm, which tend to agglomerate into larger clusters of 80–100 nm, are used in this study13Coumarin 1 was inserted into the channels of the nano-crystals before coating the silica shell via the gas diffusion method as described in ref. 5b to check the effect of silica shell on blocking the entrance of channel and to be used as an internal calibration in the luminescence sensing for DPA. The silica shell was coated on the Coumarin 1-loaded zeolite L crystals (ZL(Coumarin 1)) according to the previously described procedure.7,8 The obtained core-shell composite (ZL(Coumarin 1)@SiO2) shows strong blue color emission upon radiation under 254 nm. In order to investigate the blockage of channel entrance by the silica shell, UV/Vis measurements of supernatant solution of ZL(Coumarin 1) and ZL(Coumarin 1)@SiO2 in ethanol after 1 h of sonication were taken and the absorption spectra are shown in Fig. 1. As seen from Fig. 1a, a strong and broad band in the absorption spectrum of supernatant solution of ZL(Coumarin 1) peaking at 370 nm can be observed and can be ascribed to absorption of dyes released from the channels of nano-crystals whose entrances are open to allow for the release of guest molecules, no leakage of dye molecules from the channels can be observed as revealed by Fig. 1b, which shows the absorption spectrum of supernatant solution of ZL(Coumarin 1)@SiO2. This might be that the core-shell modification was made by first coating the zoelite with PAH (poly(allylamine hydrochloride)) followed by addition of the silica shell. The cationic groups of PAH stick to the negatively-charged channel entrances and block them. Such stopcock functions have been well described recently.12a The silica shell stabilized the so-formed composite and provides a good basis for further surface modification of the particles.
To illustrate the utility of the ZL(Coumarin 1)@SiO2 composite, we have functionalized the silica surface of the luminescent core-shell composite with a Eu(EDTA)(H2O)3 complex according to the procedure described in ref. 11, as shown in Fig. 2. It has been well recognized that three water molecules are coordinated to Eu3+ ions in the complex. The modified core-shell composite (ZL(Coumarin 1)@SiO2–Eu-EDTA) can be used as luminescence probes for anthrax and other bacteria spores by complexing to DPA that is a major constituent of many spore-forming bacteria. The dyes located in the channels of the zeolite L host are protected from any interaction with the environment of the particles and therefore provide a stable reference signal, which might then eliminate the need for instrument-specific calibration curves for quantifying the amount of DPA in an unknown sample, and also avoids the need of correcting for dilution.10 The core-shell composite (ZL(Coumarin 1)@SiO2–Eu-EDTA) thus can be used as a ratiometric sensor because DPA can coordinate to Eu3+ ions and sensitize the luminescence of Eu3+ ions.
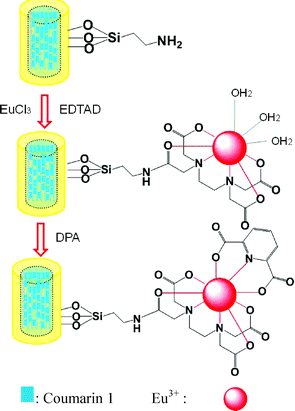 |
| Fig. 2 Illustration of the functionalization of ZL(Coumarin 1)@SiO2 with europium complexes and the principle for DPA detection. | |
ZL(Coumarin 1)@SiO2–Eu-EDTA only shows luminescence of the dye Coumarin 1 because the Eu(EDTA)(H2O)3 moiety is essentially non-emissive upon excitation at 273 nm. As DPA was added to an ethanolic dispersion of ZL(Coumarin 1)@SiO2–Eu-EDTA, line-like emission bands at 580, 594, 614, 649, and 696 nm, respectively, corresponding to the deactivation of the Eu3+ excited stated (5D0) to the ground state (7FJ, J = 0, 1, 2, 3, 4), with the hypersensitive transition 5D0→7F2 as the most prominent line (Fig. 3). This is because DPA can coordinate to the Eu(EDTA)(H2O)3 moiety and acts as sensitizer, which is the so-called “antenna effect”.14DPA absorbs UV light and efficiently transfers the absorbed energy to Eu3+ ions (intermolecular energy transfer), ultimately resulting in sensitized red emission of Eu3+ ions.
To evaluate the sensitivity of the core-shell composite used as a sensor for DPA, fluorescence titrations are conducted in the presence of ZL(Coumarin 1)@SiO2–Eu-EDTA (1 mg mL−1). As seen in Fig. 4, the intensity of Eu3+ emission is highly sensitive and increases upon the addition of increasing concentration of DPA, whereas no obvious emission change is observed for the reference dye Coumarin 1 whose luminescence band is located at about 460 nm. The Coumarin 1 molecules inside the channels of nanocrystal encapsulated in the silica are thus able to be used as an internal calibration since they are protected from the external influence. In the range from 0.1 μM to 10 μM, the maximum emission intensity at 615 nm linearly increases with the DPA concentration increasing (R2 = 0.99; ESI, Fig. 1S†). Even at very low concentration of DPA (0.02 μM), the sharp line of Eu3+ ions at 614 nm is still clearly visible (ESI, Fig. 2S†). In addition, the lifetime of 5D0 excited level of Eu3+ ion increases as the DPA concentration increases, as shown in Table 1, which could be due to the coordination of DPA to the Eu3+ ions and the displacement of the coordinating water molecules from the first coordination sphere of Eu3+ ions since the water molecules in the first coordination sphere provide an efficient route for the radiationless deactivation.15 The number of coordinated water molecules could be estimated according to the reported procedure according to the emission spectra and the lifetime of the Eu3+ first excited level.16 The number of water molecules is estimated to be 0.167 when the concentration of DPA is about 15 μM, implying all of the water has been repelled out from the first coordination sphere of Eu3+ due to the coordination of DPA.
Table 1 The Eu3+5D0 lifetime of ZL(Coumarin 1)@SiO2–Eu-EDTA in the presence of DPA
DPA concentration (μM) |
0.15 |
1.5 |
15 |
Eu3+5D0 lifetime (ms) |
0.87 |
1.13 |
1.34 |
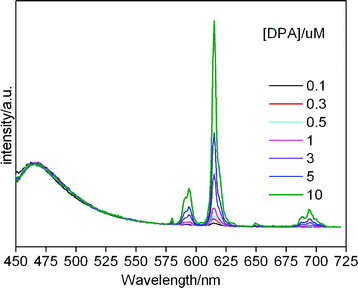 |
| Fig. 4 Luminescence of core-shell composites upon addition of different concentration of DPA. | |
Conclusions
In conclusion, we have reported the new luminescent core-shell composite composed of a dye-loaded zeolite L nano-crystal and a silica shell with non-luminescent europium complexes immobilized on the surface. The luminescent dyes loaded in the channels of microcrystal encapsulated in the silica are thus can be used as an internal calibration since they are free of leakage from inside the channels and are protected from the external influence. The immobilized europium complexes can become luminescent in the presence of dipicolinic acid (DPA) because DPA molecules can sensitize the luminescence of Eu3+ ions. The luminescent core-shell composites can thus be used as an ideal sensor for detection of DPA at nanomolar concentrations.
Experimental
Materials
Pure zeolite L crystals (100 nm) were synthesized and characterized as described previously.17 The potassium exchanged form was used. EuCl3·6H2O was obtained by dissolving Eu2O3 in hydrochloric acid (37.5%). 3-Aminopropyltriethoxysilane and Coumarin 1 were purchased from Aldrich. EDTA dianhydride was prepared to according to the reported method.11
Preparation of ZL(Coumarin 1)
ZL microcrystals were degassed and dried for 2 h at 333 K and then kept in contact with a calculated amount of coumarin 1 vapor at 383 K for 24 h. The resulting material (ZL(Coumarin 1)) was washed with EtOH for three times in order to remove only physically adsorbed coumarin 1, and dried at 40 °C in vacuum for 12 h. The loading of Coumarin 1 in zeolite L (p) is around 0.1; p is the ratio between the occupied and the total number of equivalent site in a zeolite L crystal as described in ref. 5b.
Preparation of ZL(Coumarin 1)@SiO2 and ZL(Coumarin 1)@SiO2–Eu-EDTA
ZL(Coumarin 1)@SiO2 was prepared according to the reported procedure and described as follows: ZL(Coumarin 1) (10 mg) was dispersed in deionized water (10 mL) and added dropwise to the aqueous PAH previously sonicated for 20 min. Stirring was continued for 3 h and the mixture was centrifuged at 8000 rpm for 20 min and redispersed in deionized water, ammonia was then added under vigorous stirring, and finally TEOS was added. The mixture was allowed to react for 4 h and was then centrifuged. The resulting material (ZL(Coumarin 1)@SiO2) was dried overnight. ZL(Coumarin 1)@SiO2–Eu-EDTM was prepared as follows. 0.6 mL of 3-aminopropyltriethoxysilane was added into the suspension of ZL(Coumarin 1)@SiO2 (100 mg) in 10 mL of THF under N2 atmosphere, the mixture was stirred and heated at 60 °C for 24 h, the amino-modified ZL(Coumarin 1)@SiO2 was recovered by centrifugation and washed for three times with ethanol and deionized water. The obtained core-shell composites (100 mg) were redispersed by sonication in bicarbonate buffer (15 mL, 0.1 m), EDTA dianhydride (300 mg) was added and stirred for 4 h, the as prepared core-shell composites were separated by centrifugation and then washed four times with bicarbonate buffer and two times with deionized water. Subsequently, the core-shell composites were re-dispersed in aqueous solution of EuCl3 by sonication and stirred for 5 h. The products (ZL(Coumarin 1)@SiO2–Eu-EDTA) were finally collected by centrifugation and washed with deionized water several times to remove residual EuCl3.
An aqueous solution of dipicolinic acid (DPA) sodium salt was added to the suspension of ZL(Coumarin 1)@SiO2–Eu-EDTA in ethanol (1 mg mL−1), the concentration DPA was incrementally increased from 0.05 to 15 μM. After each addition, the sample was excited at 273 nm and the emission spectrum was recorded.
Characterization
SEM images were obtained from a FE-SEM (Hitachi S-4300) at an acceleration voltage of 10 kV. UV/Vis spectra were recorded on a VARIAN CARY 50 UV/Vis spectrophotometer. The steady-state luminescence spectra measurements were measured on an Edinburgh Instruments FS920P spectrometer, with a 450 W xenon lamp as the steady-state excitation source, a double excitation monochromator (1800 lines mm−1), an emission monochromator (600 lines mm−1), and a semiconductor cooled Hamamatsu RMP928 photomultiplier tube.
Acknowledgements
This work is financially supported by the National Natural Science Foundation of China (No. 20871040, No. 20901022), Program for New Century Excellent Talents in University (NCET-09-0113), Tianjin Natural Science Foundation (09JCYBJC05700), the Scientific Program of Hebei Province (08965110D) and Hebei Province Natural Science Foundation for Distinguished Young Scholars (No. B2010000034).
Notes and references
-
(a) Y. Lu, J. McLellan and Y. Xia, Synthesis and crystallization of hybrid spherical colloids composed of polystyrene cores and silica shells, Langmuir, 2004, 20, 3464–3470 CrossRef CAS;
(b) Y. Lu, Y. Yin, B. T. Mayers and Y. Xia, Modifying the surface properties of superparamagnetic iron oxide nanoparticles through a sol–gel approach, Nano Lett., 2002, 2, 183–186 CrossRef CAS;
(c) S. T. Selvan, P. K. Patra, C. Y. Ang and J. Y. Ying, Synthesis of silica-coated semiconductor and magnetic quantum dots and their use in the imaging of live cells, Angew. Chem., Int. Ed., 2007, 46, 2448–2452 CrossRef CAS;
(d) L. M. LizMarzan, M. Giersig and P. Mulvaney, Synthesis of nanosized gold-silica core-shell particles, Langmuir, 1996, 12, 4329–4335 CrossRef CAS;
(e) Q. Fu, C. Lu and J. Liu, Selective coating of single wall carbon nanotubes with thin SiO2 layer, Nano Lett., 2002, 2, 329–332 CrossRef CAS;
(f) P. Jiang, J. F. Bertone and V. L. Colvin, A lost-wax approach to monodisperse colloids and their crystals, Science, 2001, 291, 453–457 CrossRef CAS;
(g) W. J. Rieter, K. M. L. Taylor and W. B. Lin, Surface modification and functionalization of nanoscale metal–organic frameworks for controlled release and luminescence sensing, J. Am. Chem. Soc., 2007, 129, 9852–9853 CrossRef CAS.
- L. M. Liz-Marz_n and P. Mulvaney, The assembly of coated nanocrystals, J. Phys. Chem. B, 2003, 107, 7312–7326 CrossRef CAS.
- Z. L. Wang, R. P. P. Gao, J. L. Gole and J. D. Stout, Silica nanotubes and nanofiber arrays, Adv. Mater., 2000, 12, 1938–1940 CAS.
- R. Koole, M. M. van Schooneveld, J. Hilhorst, C. D. Donega, D. C. ťHart, A. van Blaaderen, D. Vanmakekelbergh and A. Meijerink, On the incorporation mechanism of hydrophobic quantum dots in silica spheres by a reverse microemulsion method, Chem. Mater., 2008, 20, 2503–2512 CrossRef CAS.
-
(a) G. Calzaferri and K. Lutkouskaya, Mimicking the antenna system of green plants, Photochem. Photobiol. Sci., 2008, 7, 879–910 RSC;
(b) G. Calzaferri, S. Huber, H. Maas and C. Minkowski, Host–guest antenna materials, Angew. Chem., Int. Ed., 2003, 42, 3732–3758 CrossRef CAS;
(c) Y. Wang, H. R. Li, Y. Feng, H. J. Zhang, C. Gion and T. Z. Ren, Orienting zeolite L microcrystals with a functional linker, Angew. Chem., Int. Ed., 2010, 49, 1434–1438 CAS;
(d) Y. G. Wang, H. R. Li, L. J. Gu, Q. Y. Gan, Y. N. Li and G. Calzaferri, Thermally stable luminescent lanthanide complexes in zeolite L, Microporous Mesoporous Mater., 2009, 121, 1–6 CrossRef CAS;
(e) M. M. Tsotsalas, K. Kopka, G. Luppi, S. Wagner, M. P. Lwa, M. Schafers and L. De Cola, Encapsulating 111In in nanocontainers for scintigraphic imaging: synthesis, characterization, and in vivo biodistribution, ACS Nano, 2010, 4, 342–348 CrossRef CAS.
-
(a) M. Pauchard, A. Devaux and G. Calzaferri, Dye-loaded zeolite L sandwiches as –artificial antenna systems for light transport, Chem.–Eur. J., 2000, 6, 3456–3470 CrossRef CAS;
(b) D. Brühwiler and G. Calzaferri, Molecular sieves as host materials for supramolecular organization, Microporous Mesoporous Mater., 2004, 72, 1–23 CrossRef;
(c) G. Calzaferri, D. Brühwiler, S. Megelski, M. Pfenniger, M. Pauchard, B. Hennessy, H. Maas, A. Devaux and U. Graf, Playing with dye molecules at the inner and outer surface of zeolite L, Solid State Sci., 2000, 2, 421 CrossRef.
- A. Guerrero-Martinez, S. Fibikar, I. Pastoriza-Santos, L. M. Liz-Marz_n and L. De Cola, Fluorescent anisotropic zeolite L core – isotropic silica shell containers, Angew. Chem., Int. Ed., 2009, 48, 1266–1270 CrossRef CAS.
- H. R. Li, W. J. Cheng, Y. Wang, B. Y. Liu, W. J. Zhang and H. J. Zhang, Surfacemodification and functionalization of microporous hybrid material for luminescence sensing, Chem.–Eur. J., 2010, 16, 2125–2130 CrossRef CAS.
-
(a) K. Hanaoka, Ki. Kikuchi, H. Kojima, Y. Urano and T. Nagano, Selective detection of zinc ions with novel luminescent lanthanide probes, Angew. Chem., Int. Ed., 2003, 42, 2996–2999 CrossRef CAS;
(b) J. Massue, S. J. Quinn and T. Gunnlaugsson, Lanthanide luminescent displacement assays: the sensing of phosphate anions using Eu(III)-cyclen-conjugated gold nanoparticles in aqueous solution, J. Am. Chem. Soc., 2008, 130, 6900–690 CrossRef CAS.
-
(a) H. Tsukube and S. Shinoda, Lanthanidecomplexes in molecular recognition and chirality sensing of biological substrates, Chem. Rev., 2002, 102, 2389–2404 CrossRef CAS;
(b) S. Pandya, J. Yu and D. Parker, Engineering emissive europium and terbium complexes for molecular imaging and sensing, Dalton Trans., 2006, 2757–2766 RSC;
(c) K. M. L. Taylar and W. B. Lin, Designing metal–organic frameworks for catalytic applications, J. Mater. Chem., 2009, 19, 6418–6422 RSC.
- K. L. Ai, B. H. Zhang and L. H. Lu, Europium-based fluorescence nanoparticle rensor for Rapid and ultrasensitive Detection of an Anthrax Biomarker, Angew. Chem., Int. Ed., 2009, 48, 304–308 CrossRef CAS.
-
(a) D. Brühwiler, G. Calzaferri, T. Torres, J. H. Ramm, N. Gartmann, L.-Q. Dieu, I. López-Duarte and M. V. Martínez-Díaz, Nanochannels for supramolecular organization of luminescent guests, J. Mater. Chem., 2009, 19, 8040–8067 RSC;
(b) R. Q. Albuquerque, J. Kühni, P. Belser and L. De Cola, On the Reversible Photoisomerization of spiropyran-modified zeolite L single crystals, ChemPhysChem, 2010, 11, 575–578 CrossRef CAS.
- Y. G. Wang, H. R. Li, B. Y. Liu and W. J. Zhang, Luminescene properties of nanozeolite L grafted with terbium organic complex, Mater. Lett., 2008, 62, 3167–3170 CrossRef CAS.
- J.-C. Bünzli and C. Piguet, Taking advantage of luminescent lanthanide ions, Chem. Soc. Rev., 2005, 34, 1048–1077 RSC.
- K. Binnemans, P. Lenaerts, K. Driesen and C. Goerller-Walrand, A luminescent tris(2-thenoyltrifluoroacetonato)europium(III) complex covalently linked to a 1,10-phenanthroline-functionalised sol–gel glass, J. Mater. Chem., 2004, 14, 191–195 RSC.
- S. C. Nunes, V. de Zea Bermudez, M. M. Silva, M. J. Smith, D. Ostrovskii, R. A. Sá Ferreira, L. D. Carlos, J. Rocha, A. Gonçalves and E. Fortunato, Sol–Gel Derived Potassium-Based Di-Ureasils for Smart Windows, J. Mater. Chem., 2007, 17, 4239–4248 RSC.
- S. Megelski and G. Calzaferri, Tuning the size and shape of zeolite L-based inorganic–organic host–guest composites for optical antenna systems, Adv. Funct. Mater., 2001, 11, 277–286 CrossRef CAS.
Footnote |
† Electronic supplementary information (ESI) available: Fig. 1S: Emission intensity at 615 nm of the core-shell composite as a function of DPA concentration; Fig. 2S: Emission spectrum of the core-shell composites upon addition of 0.05 μM of DPA. See DOI: 10.1039/c0pp00273a |
|
This journal is © The Royal Society of Chemistry and Owner Societies 2011 |