DOI:
10.1039/C0NR00749H
(Paper)
Nanoscale, 2011,
3, 1166-1170
Alginate stabilized silver nanocube–Rh6G composite as a highly selective mercury sensor in aqueous solution†
Received
6th October 2010
, Accepted 6th December 2010
First published on 14th January 2011
Abstract
Alginate-stabilized silver nanocubes are synthesized via a reduction method and are characterized by UV-Vis and fluorescence spectroscopies, SEM, AFM and HRTEM analyses. A silver nanocubes (Ag NCbs) based sensor for detecting Hg2+ ions in aqueous solution has been developed using Rh6G as an external spectroscopic probe. Using this system, Hg2+ ions (as low as 1 × 10−10 mol L−1) are recognized in aqueous media via a colorimetric method with very high selectivity and sensitivity over other metal ions namely Fe2+, Zn2+, Pb2+, Cu2+, Sn2+, Cd2+, Ni2+, and Co2+.
1. Introduction
Integration of “green chemistry” principles into nanoscience and nanotechnology has attracted considerable attention during the past decade. The choice of an environmentally benign solvent and the use of a multipurpose reagent that serves the purposes of reducing, capping and dispersing agents are some of the key issues that may be addressed in green synthesis of nanomaterials.1Silver nanoparticles continue to arouse intense scientific interest, due to their potential in designing novel materials for applications in a variety of areas including catalysis,2 electronics,3 photonics,4 optoelectronics,5 sensing6 and pharmaceuticals.7 Meanwhile, the synthesis of noble metal nanomaterials by biomolecules such as amino acids,8 microorganisms9 and biopolymers10 has also drawn lot of interest.
Control over shape selective preparation of metal nanoparticles11 (MNPs) is essential for the fundamental understanding and practical applications of their nanoscale properties.12 Also the controllable preparation of nanocrystals with different shapes and exposed surfaces is very important and challenging. Silver has an array of properties that could be tuned or enhanced through the nanoscale control of morphology and significant research efforts have been invested into shape selection and control of AgNPs. In addition to single-crystalline cubes13 and octahedra,14 diverse silver nanoshapes, including pentagonal wires,15 right bipyramids,16 pentagonal bipyramids,17 nanobars and nanorices18 are reported. Currently, considerable attention has been focused on developing simple, inexpensive colorimetric sensors for the selective and rapid determination of toxic heavy metal ions, such as the Pb2+, Cd2+ and Hg2+ ions.19Mercury, in particular, is a widespread pollutant with distinct toxicological profiles and it exists in a variety of forms (metallic, ionic and as a part of organic/inorganic salts and complexes). Recently gold nanoparticles and rhodamine 6G based fluorescent sensors for mercury(II)20 are reported. However, gold is costlier and also previous reports use chelating ligands for binding mercury(II). Therefore, a convenient, rapid, simple and selective method for the sensing of Hg2+ is highly desirable. Herein, we report the shape selective synthesis of silver nanocubes in water using alginate as a stabilizer and successful investigation of selective sensing of mercury using rhodamine 6G (Rh6G) as a spectroscopic probe.
2. Experimental details
2.1 Chemicals
Sodium alginate, silver nitrate (99.99%) and Rh6G were purchased from Aldrich. Sodium borohydride (A.R.) and mercuric chloride were purchased from Merck. All other chemicals were used as received without further purification. Deionized water was used throughout the experiments.
2.2 Synthesis of alginate stabilized silver nanocubes
Silver nanocubes were synthesized by reducing silver nitrate (AgNO3) in sodium borohydride in the presence of sodium alginate. In a simple synthesis, 1 mL of 1% (w/v) sodium alginate (dissolved in 1% (v/v) acetic acid) solution in water and 1 mL of freshly prepared 1.0 × 10−4 M AgNO3 solution were mixed in a reaction tube at room temperature with vigorous stirring for about 15 min. After that, 1 mL of 0.1 M NaBH4 solution was added dropwise into the reaction tube with constant stirring at room temperature. After 15 min, the appearance of a yellow color in the solution indicated the formation of alginate stabilized silver nanocubes (alginate–Ag NCbs).
2.3 Adsorption of Rh6G on the surface of alginate silver nanocubes
Alginate–Ag NCbs with Rh6G were prepared in a simple process by adding 1 mL of Rh6G solution (1.0 × 10−5 mol L−1) to 1 mL of alginate–Ag NCbs solution (1.0 × 10−7 mol L−1, ε =5.4 × 106 L mol−1 cm−1, pH 4.4). The process of adsorption of Rh6G on the surface of alginate–Ag NCbs was equilibrated at room temperature only after 20 min. Control experiments show that there is no variation in pH and other parameters such as ionic strength for several days, indicating that there is no dissociation of Rh6G from the Ag NCbs under the present conditions.
2.4 Characterization of materials
The above materials were then characterized. UV-Visible absorption spectra were recorded by using the JASCO-Spectra Manager (V-550) in 1 cm path length quartz cuvette with a volume of 2 mL at room temperature. All fluorescence measurements were made on a Fluoromax-4 Spectrofluorometer (HORIBA JOBIN YVON) with excitation slit set at 2.0 nm bandpass and emission at 4.0 nm bandpass in 1 cm × 1 cm quartz cell. The scanning electron microscopic (SEM) measurements were carried out with JEOL-JSM-6390. Atomic Force Microscopy (AFM) images were recorded by using A-100 SGS. High resolution transmission electron microscopy (HRTEM) images were obtained using a Philips CM12 TEM operating at 200 kV. The electron diffraction pattern was also recorded for the selected area.
3. Results and discussion
The proposed sensing of Hg2+ ion with the present system is easily followed through its distinctive color changes. After the addition of NaBH4 to a solution of sodium alginate and AgNO3, the solution becomes light yellow in color within one minute, indicating the formation of alginate silver nanocubes through reduction. The intensity of yellow color goes on increasing until 5 min, after which it remains constant for another 15 min (Fig. S1a†). UV-Visible spectrum is recorded in the absorbance mode in the range 300–700 nm. The surface plasmon resonance peak appearing at 399 nm shows a broad band due to the uniform size distribution. The emission spectrum is also recorded for water based alginate silver nanocubes. The excitation wavelength is fixed at 410 nm and fluorescence emission occurs at 466 nm (Fig. S1b†).
3.1 Morphological analysis of the materials
The SEM morphology of the alginate silver nanocubes indicates the shape dependence of alginate silver nanocubes on the reaction conditions. When the concentration of AgNO3 is increased by a factor of the molar ratio between the repeating unit of sodium alginate and AgNO3, single-crystalline nanocubes of silver are obtained. Fig. 1(a) and (b) show scanning electron microscope (SEM) images of a typical sample of alginate silver nanocubes and indicate the large quantity and good uniformity that are achieved using this approach. These alginate silver nanocubes have a mean edge length of around 56 nm with a standard deviation of ∼9.5 nm. Fig. 1(c) and (d) show the smooth surface and perfectly arranged corners and edges of these nanocubes.
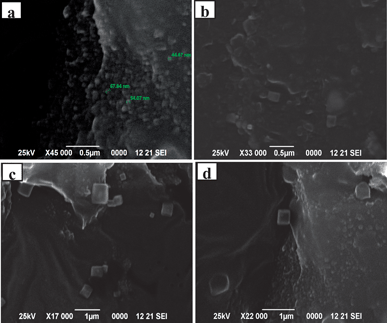 |
| Fig. 1
SEM images of alginate silver nanocubes: (a) and (b) indicate good uniformity and various sizes, (c) and (d) indicate smooth surface and individual perfect crystalline nanocubes. | |
Fig. 2(a) shows the high resolution transmission electron microscopy (HRTEM) image of highly dispersed alginate stabilized Ag nanocubes. The 2D lattice fringe of the HRTEM image is found to be 0.2 nm (Fig. 2(b)) and the particles size was around 20–30 nm. Fig. 2(c) indicates the well crystalline cubic form of nano-Ag. HRTEM images clearly show the edges of the cubes. Fig. 2(d) shows the corresponding selected area electron diffraction (SAED) pattern, obtained by directing the incident electron beam perpendicular to one of the square facets of the cube. The square spot array is indexed to four clear diffraction rings that are assignable to diffractions from (111), (200), (220), and (311) planes of the fcc nano-silver.
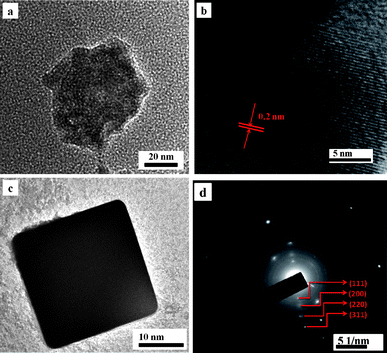 |
| Fig. 2 (a) HRTEM images of highly dispersed alginate stabilized Ag nanocubes. (b) The 2D lattice fringes of the HRTEM image. (c) HRTEM image of smooth surface and individual perfect crystalline nanocube. (d) Selected area diffraction pattern of alginate silver nanocubes in corresponding planes. | |
AFM is further used to define the morphology of alginate silver nanocubes and the data (Fig. S2†) show that the surface roughness of nano-silver is about 17 nm. Once again, the square forms of the cross-section of the silver nanocubes self-arranged in a similar direction which is clearly seen in the two-dimensional (2D) AFM image (Fig. S2a†). The AFM image analysis shows that the average edge length (side length) and height of the cubic nanoparticles are in the range 10–17 nm, respectively. From the height profile of the three-dimensional (3D) AFM image (Fig. S2b†) the average out-of-plane thickness of pure alginate silver nanocubes is found to be about 5 nm.
3.2
Detection of
mercury(II) ions with alginate silver nanocube–Rh6G sensor
Besides the two basic characteristics such as the sensitivity and selectivity of a fluorescence sensor, the sensor response time to analytes and its stability are very crucial for practical applications of alginate–Ag NCbs based sensor for Hg2+ ion. As indicated in Scheme 1, the sensing mechanism occurs via two steps: in step (i), Rh6G dye molecule is bound to the surface of Ag NCbs and in step (ii), Hg2+ ions displace the bound Rh6G molecule from the surface of Ag NCbs. The absorbance and emission spectra of free Rh6G and those of Ag NCbs bound Rh6G in the absence and presence of Hg2+ ions are shown in Fig. 3. In Fig. 3, the SPR band (a) of alginate bound Ag nanocube (399 nm) shows a decrease in intensity when Rh6G binds to it (b). Addition of Hg2+ ion causes a slight blue shift of the surface-plasmon absorption band (c).
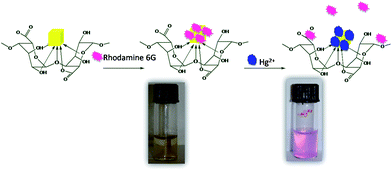 |
| Scheme 1 Sensing mechanism of mercury(II) ions. | |
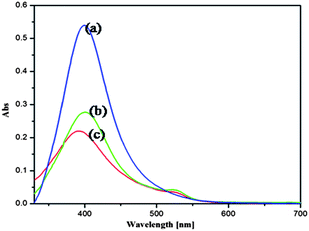 |
| Fig. 3
UV-Vis
absorbance spectra of (a) alginate–Ag NCbs, (b) alginate–Ag NCbs–Rh6G composite in the absence of Hg2+ and (c) alginate–Ag NCbs–Rh6G composite in the presence of Hg2+ ion. | |
This is explained as follows: the Hg2+ ions are reduced by silver nanocubes to form a mercury layer around alginate–silver nanocubes, resulting in a moderate broadening and blue shift of the SPR band. The generated mercury atoms on the silver surface release Rh6G to form a solid amalgam-like structure, which could be accounted for the slight blue shift of the surface-plasmon absorption band of alginate–silver nanocubes.
The emission spectrum of free Rh6G exhibits a peak at 551 nm (Fig. 4(a)) which is greatly diminished (Fig. 4(b)) when it is bound to alginate–silver nanocubes. Addition of Hg2+ releases Rh6G restoring its original emission intensity (Fig. 4(c)). These features strongly support the proposed Scheme 1, for Hg2+ sensing.
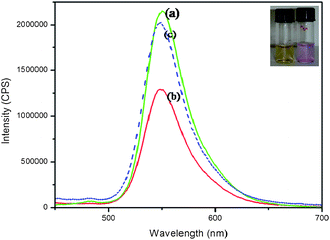 |
| Fig. 4
Fluorescence spectra of (i) Rh6G (1.0 × 10−5 mol L−1), (ii) alginate–Ag NCbs–Rh6G composite in the absence of Hg2+ and (iii) alginate–Ag NCbs–Rh6G composite in the presence of Hg2+ ions (1.0 × 10−5 mol L−1). | |
It is interesting to note that this system acts as a visual colorimetric sensor and exhibits remarkable selectivity for Hg2+ over other metal ions. After the addition of 1.0 × 10−5 mol L−1HgCl2 to a solution of Rh6G with alginate–Ag NCbs, the observed fluorescence restoration of the sensor is associated with a concomitant color change from yellow to purple indicating Rh6G moiety is liberated from the surface of alginate–Ag NCbs.
The response of various environmentally relevant metal ions such as Hg2+, Fe2+, Zn2+, Pb2+, Cu2+, Sn2+, Cd2+, Ni2+ and Co2+ at a concentration of 1.0 × 10−5 mol L−1 is given in Fig. 5. Only Hg2+ shows a significant color change, whereas all other metal ions remain light yellow without any perceptible color change. It is also remarkable to note that [Hg2+] as low as 1.0 × 10−10 mol L−1 is sensed by this system by its perceptible color change.
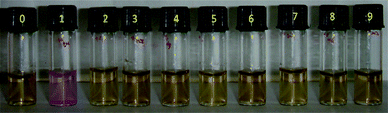 |
| Fig. 5
Alginate–silver nanocubes (1.0 × 10−7 mol L−1) with bound Rh6G (1.0 × 10−5 mol L−1) solution (pH 4.4) in the presence of various metal ions. From left: 0, control; 1, Hg2+; 2, Fe2+; 3, Zn2+; 4, Pb2+; 5, Cu2+; 6, Sn2+; 7, Cd2+; 8, Ni2+; 9, Co2+ (1.0 × 10−5 mol L−1). | |
From the AFM image of (Fig. S3†) alginate–Ag NCbs with bound Rh6G system in the absence and presence of mercury(II) ions, the thickness of alginate–Ag NCbs with Rh6G is estimated to be about 10–17 nm and after the addition of Hg2+, the thickness increases to about 20–30 nm indicating that Hg2+ ion is adsorbed on the alginate–Ag NCbs surface.
Experimental results also show that the Rh6G concentration produces a large effect on the fluorescence background of the sensor and its sensitivity toward Hg2+ ions. The amount of Rh6G molecules released from the alginate–Ag NCbs surface due to the binding of the Hg2+ ions is estimated at different concentrations of Hg2+ ions. As shown in Fig. 6, the fluorescence intensity of the sensor (1.0 × 10−7 mol L−1alginate–Ag NCbs and 1.0 × 10−5 mol L−1Rh6G) is restored in the presence of 1.0 × 10−5Hg2+ ions.
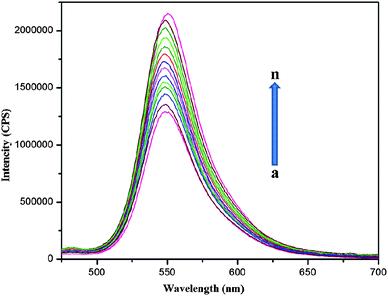 |
| Fig. 6 Fluorescence response of alginate–Ag NCbs with Rh6G at different concentrations of Hg2+ in aqueous solution (5.0 × 10−11 to 1.0 × 10−5 mol L−1). (a) Blank; (b) 5.0 × 10−11; (c) 1.0 × 10−10; (d) 5.0 × 10−10; (e) 1.0 × 10−9; (f) 5.0 × 10−9; (g) 1.0 × 10−8; (h) 5.0 × 10−8; (i) 1.0 × 10−7; (j) 5.0 × 10−7; (k) 1.0 × 10−6; (l) 5.0 × 10−6; (m) 1.0 × 10−5; (n) Rh6G 1.0 × 10−5 mol L−1. | |
The fluorescence intensity is increased linearly with the increase in the concentration of Hg2+ from 5.0 × 10−11 to 1.0 × 10−5 mol L−1. From Fig. 7, the value of linearly dependent coefficient (R2) was found to be 0.99547 and the detection limit is 5.0 × 10−11 mol L−1.
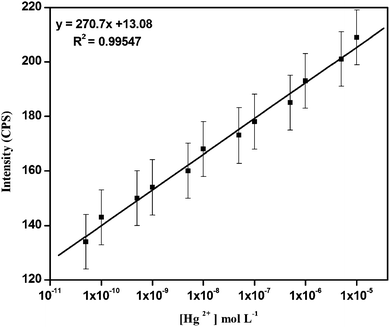 |
| Fig. 7 Plot of fluorescence intensity change (triplicate) of varied concentration of Hg2+ from 5.0 × 10−11 to 1.0 × 10−5 mol L−1. (λex = 470 nm, λem = 548 nm, slit: 2 nm/4 nm). | |
4. Conclusion
Shape selective silver nanocubes are synthesized in aqueous medium using alginate as a stabilizer and are characterized by UV-Vis and fluorescence spectroscopies, SEM, AFM and HRTEM analyses. Rh6G dye is added to the above and these alginate–Ag NCbs with Rh6G based nanosensor show remarkable and selective colorimetric sensing for mercury(II) ions in water.
Acknowledgements
Financial assistance from University Grants Commission (for UPE programme to Madurai Kamaraj University) is gratefully acknowledged.
References
-
(a) P. Raveendran, J. Fu and S. L. Wallen, J. Am. Chem. Soc., 2003, 125, 13940 CrossRef CAS;
(b) P. Raveendran, J. Fu and S. L. Wallen, Green Chem., 2006, 8, 34 RSC.
- N. R. Jana, T. K. Sau and T. Pal, J. Phys. Chem. B, 1999, 103, 115 CrossRef.
- G. N. R. Tripathi, J. Am. Chem. Soc., 2003, 125, 1178 CrossRef CAS.
- W. Wang and S. A. Asher, J. Am. Chem. Soc., 2001, 123, 12528 CrossRef CAS.
- Y. Cui and C. M. Lieber, Science, 2001, 291, 851 CrossRef CAS.
- A. D. McFarland and R. P. Van Duyne, Nano Lett., 2003, 3, 1057 CrossRef CAS.
- E. Ulkur, O. Oncul, H. Karagoz, E. Yeniz and B. Celikoz, Burns, 2005, 31, 874 CrossRef.
-
(a) Y. Saito, J. J. Wang, D. A. Smith and D. N. Batchelder, Langmuir, 2002, 18, 2959 CrossRef CAS;
(b) Y. Shao, Y. D. Jin and S. J. Dong, Chem. Commun., 2004, 1104 RSC;
(c) J. Yang, L. Lu, H. Wang, W. Shi and H. Zhang, Cryst. Growth Des., 2006, 6, 2155 CrossRef CAS.
-
(a) A. Ahmad, S. Senapati, M. I. Khan, R. Kumar and M. Sastry, Langmuir, 2003, 19, 3550 CrossRef CAS;
(b) P. Mukherjee, A. Ahmad, D. Mandal, S. Senapati, S. R. Sainkar, M. I. Khan, R. Parishcha, P. V. Ajaykumar, M. Alam, R. Kumar and M. Sastry, Nano Lett., 2001, 1, 515 CrossRef CAS.
-
(a) J. He, T. Kunitake and A. Nakao, Chem. Mater., 2003, 15, 4401 CrossRef CAS;
(b) T. K. Sarma and A. Chattopadhyay, Langmuir, 2004, 20, 3520 CrossRef CAS;
(c) A. V. Singh, B. M. Bandgar, M. Kasture, B. L. V. Prasad and M. Sastry, J. Mater. Chem., 2005, 15, 5115 RSC;
(d) R. Brayner, T. Coradin, M. J. Vaulay, C. Mangeney, J. Livage and F. Fievet, Colloids Surf., A, 2005, 256, 191 CrossRef CAS.
-
(a) F. Kim, S. Connor, H. Song, T. Kuykendall and P. D. Yang, Angew. Chem., Int. Ed., 2004, 43, 3673 CrossRef CAS;
(b) Y. G. Sun and Y. N. Xia, Science, 2002, 298, 2176 CrossRef CAS.
-
(a) Y. Yin and A. P. Alivisatos, Nature, 2005, 437, 664 CrossRef CAS;
(b) C. Burda, X. B. Chen, R. Narayanan and M. A. El-Sayed, Chem. Rev., 2005, 105, 1025 CrossRef CAS;
(c) M. P. Pileni, J. Phys. Chem. C, 2007, 111, 9019 CrossRef CAS.
- Y. Xia and S. E. Skrabalak, Nat. Protoc., 2007, 2, 2182 Search PubMed.
- J. W. Yoo, S. M. Lee, H. T. Kim and M. A. El-Sayed, Bull. Korean Chem. Soc., 2004, 25, 3.
- Y. Sun, B. Gates, B. Mayers and Y. Xia, Nano Lett., 2002, 2, 165 CrossRef CAS.
- B. J. Wiley, Y. J. Xiong, Z. Y. Li, Y. D. Yin and Y. A. Xia, Nano Lett., 2006, 6, 765 CrossRef CAS.
- X. Zhang, M. Tsuji, S. Lim, N. Miyamae, M. Nishio, S. Hikino and M. Umezu, Langmuir, 2007, 23, 6372 CrossRef CAS.
- B. J. Wiley, Y. C. Chen, J. M. McLellan, Y. J. Xiong, Z. Y. Li, D. Ginger and Y. N. Xia, Nano Lett., 2007, 7, 1032 CrossRef CAS.
-
(a) J. Liu and Y. Lu, J. Am. Chem. Soc., 2003, 125, 6642 CrossRef CAS;
(b) C. T. Chen and W. P. Huang, J. Am. Chem. Soc., 2002, 124, 6246 CrossRef CAS;
(c) S. Deo and H. A. Godwin, J. Am. Chem. Soc., 2000, 122, 174 CrossRef CAS;
(d) M. Choi, M. Kim, K. D. Lee, K. N. Han, I. A. Yoon, H. J. Chung and J. Yoon, Org. Lett., 2001, 3, 3455 CrossRef CAS;
(e) J. E. Marino, J. C. Resendiz, N. H. Disteldorf, S. Fischer and P. J. Stang, Org. Lett., 2004, 6, 651 CrossRef CAS;
(f) R. T. Bronson, D. J. Michaelis, R. D. Lamb, G. A. Husseini, P. B. Farnsworth, M. R. Linford, R. M. Izatt, J. S. Bradshaw and P. B. Savage, Org. Lett., 2005, 7, 1105 CrossRef CAS;
(g) X. Peng, J. Du, J. Fan, J. Wang, Y. Wu, J. Zhao, S. Sun and T. Xu, J. Am. Chem. Soc., 2007, 129, 1500 CrossRef CAS;
(h) A. Coskun and E. U. J. Akkaya, J. Am. Chem. Soc., 2006, 128, 14474 CrossRef CAS;
(i) A. B. Descalzo, M. R. Martınez, R. Radeglia, K. Rurack and J. Soto, J. Am. Chem. Soc., 2003, 125, 3418 CrossRef CAS.
-
(a) C. C. Huang and H. T. Chang, Anal. Chem., 2006, 78, 8332 CrossRef CAS;
(b) J. Chen, A. Zheng, A. Chen, Y. Gao, C. He, X. Kai, G. Wa and Y. Chen, Anal. Chim. Acta, 2007, 599, 134 CrossRef CAS.
Footnote |
† Electronic supplementary information (ESI) available: Additional information concerning UV-Vis and emission spectra of alginate–Ag NCbs, 2D and 3D AFM images of alginate–Ag NCbs, 3D AFM image of alginate–Ag NCbs–Rh6G with the absence and presence of Hg2+. See DOI: 10.1039/c0nr00749h |
|
This journal is © The Royal Society of Chemistry 2011 |
Click here to see how this site uses Cookies. View our privacy policy here.