DOI:
10.1039/C1MD00144B
(Concise Article)
Med. Chem. Commun., 2011,
2, 973-976
A method for estimating the risk of drug-induced phototoxicity and its application to smoothened inhibitors†
Received
6th June 2011
, Accepted 25th July 2011
First published on 18th August 2011
Abstract
The energy difference between the frontier-orbital HOMO–LUMO gaps calculated for the ground state of marketed oral drugs correlated with their observed phototoxicity. This molecular descriptor, together with their maximal molar absorptivity for UV light above 290 nm, can be used to predict phototoxicity risks. This is demonstrated for the phototoxicity mitigation of 1-piperazinyl phthalazines, a class of smoothened inhibitors.
Introduction
Drug-induced phototoxicity is recognized as the adverse effect of a topical or systemic medication caused by exposure to light.1 Phototoxicity reactions, sometimes also described as photosensitivity reactions, can be categorized as either photoirritant, photogenotoxic or photoallergic reactions. However, they have in common that a drug with distribution to skin or eye becomes reactive in the presence of light inducing undesirable side-effects. A large number of marketed drugs have been reported to cause phototoxicity.1a This includes commonly used drugs such as antimicrobials of the fluoroquinolone2 and COMPOUND LINKS
Read more about this on ChemSpider
Download mol file of compoundtetracycline3 classes or non-steroidal anti-inflammatory drugs4 (NSAIDs), particularly 2-arylpropionic acid derivatives such as COMPOUND LINKS
Read more about this on ChemSpider
Download mol file of compoundnaproxen, COMPOUND LINKS
Read more about this on ChemSpider
Download mol file of compoundbenoxaprofen and COMPOUND LINKS
Read more about this on ChemSpider
Download mol file of compoundketoprofen. Although it is possible to minimise the adverse reaction from sun exposure by means of sunscreen, protective clothing and eyewear, phototoxicity introduces a serious limitation in the use of a medication. Within a compound class, a drug with a high incidence of phototoxicity will find reduced therapeutic use compared to one with a low incidence as demonstrated for the fluoroquinolone antimicrobials.5 In rare cases, drugs have even been withdrawn because of photosensitivity effects that appeared after more widespread clinical use.6 Clearly, identifying drug candidates without a phototoxicity liability is highly desirable and is a significant advantage in a competitive market environment.
The phototoxic potential of a drug candidate can be evaluated clinically by human volunteer testing7 or preclinically in animal models such as the murine UV-local lymph node assay (UV-LLNA).8 These tests allow the evaluation of the phototoxic liability of a compound during development, but faster and less resource-intensive approaches are required at the early phase of the drug discovery process. Among the in vitro prediction tools are systems such as the reactive oxygen species (ROS) assay9 and the 3T3 neutral red uptake (NRU) phototoxicity test in mouse fibroblasts.10 However, these assays might not be routinely available with a high throughput and fast turn-around time during early drug discovery. A major advantage of an in silico method is that it allows the evaluation of the phototoxic potential of a virtual compound before it has been synthesized. One expert system based on the chemical structures of known phototoxins has been developed.11 However, the limitation of rule-based expert systems is that they cannot extrapolate beyond the known phototoxic compounds. In this article we present a simple method combining the calculation of the frontier orbital HOMO–LUMO energy gap with measuring the UV absorption of a compound to estimate the risk of phototoxicity. This new method is applied in the lead optimization of 1-piperazinyl phthalazines, a class of smoothened (Smo) inhibitors developed as novel anticancer agents for the treatment of Hedgehog-dependent tumors.12
Results and discussion
The hardness of a molecule is defined as the gap in energies between the HOMO and LUMO frontier orbitals.13 Pearson notes that “the most stable structure has the largest HOMO–LUMO energy gap.” Phototoxicity requires activation of a molecule by UV-visible light. Therefore, the magnitude of a molecule's HOMO–LUMO gap should have some relationship to the potential for phototoxicity. Evidence for this hypothesis has been provided by Mekenyan et al. in their study on the photoinduced acute toxicity of polycyclic aromatic hydrocarbons (PAH).14 They showed that the phototoxic effect of PAH to aquatic organisms can be described by using the energy gap of the frontier orbitals as a relevant molecular descriptor. The HOMO–LUMO gap of the ground state was used as it relates to light absorption and molecular stability.15 Their work resulted in a bell-shaped relationship between HOMO–LUMO gap and phototoxicity with large and small energy gaps leading to lower toxicity. This was explained that large gaps (>7.1 eV for PAH) lead to stable, non-absorbing and non-phototoxic chemicals whereas PAHs with HOMO–LUMO gaps less than 5.8 eV are unlikely to be stable in COMPOUND LINKS
Read more about this on ChemSpider
Download mol file of compoundwater and degrade too rapidly to enable phototoxicity to aquatic organisms. This work has been later confirmed using a larger set of PAHs and various aquatic organisms by two other research groups.16 Independently from our work, Ringeissen et al. recently expanded the analysis of HOMO–LUMO gaps to a set of 56 chemicals including drugs, fragrances and sunscreens with 3T3 NRU in vitro phototoxicity data.17 This work identified a wide phototoxicity window from 6.5 to 8.6 eV.
Calculating this molecular descriptor using the semi-empirical AM1 method for a set of 745 marketed oral drugs and classifying these drugs as “non”, “low” or “medium/high” phototoxic compounds based on literature reports of clinical occurrence of phototoxicity, we identified a correlation between experimental phototoxicity and HOMO–LUMO energy gap (Table 1).18 This is particularly remarkable as our analysis is based on systemic drugs, but without any consideration for the differences in drug distribution to skin or eye. Attempts to further improve the phototoxicity discrimination by using a second descriptor related to drug distribution such as lipophilicity were not successful. As already observed by Verdel et al. both drugs with highly lipophilic as well as hydrophilic character can result in phototoxic effects.19 Of the 54 medium/high phototoxicity drugs, 82% were correctly predicted to be phototoxic (“likely or possible”) using only HOMO–LUMO gap energy. A drug with a HOMO–LUMO gap between 6.7 and 7.7 eV has ∼50% chance for medium/high phototoxicity in human whereas a HOMO–LUMO gap greater than 8.1 eV lessens significantly the chances of phototoxicity: Only 1.6% of the drugs in this range showed medium/high phototoxicity. Drugs with an energy gap of less than 6.7 eV are very rare and a prediction is not possible based on this insufficient data set.
Table 1 Correlation of HOMO–LUMO gap energy with reported human phototoxicity for oral drugs binned into three categoriesa,b
Prediction |
HOMO–LUMO gap range (eV) |
High/medium phototoxicity |
Low phototoxicity |
Non phototoxic |
Each cell contains the number of oral drugs in the category.
See ESI for details of classification.†
|
inconclusive |
< 6.7 |
1 |
1 |
2 |
likely |
6.7–7.7 |
28 |
7 |
23 |
possible |
7.7–8.1 |
16 |
18 |
71 |
unlikely |
> 8.1 |
9 |
80 |
489 |
To complement the calculated energy gaps as a molecular descriptor we turned our attention to absorption of UV light as this can be measured in most analytical laboratories with minimal resources. Of particular interest was the molar absorptivity (also: molar extinction coefficient, MEC, ε) as this value is an indicator for the intensity of light absorption, a prerequisite for any light-induced toxicity. As UV radiation between 200 and 290 nm is largely filtered by the earth's atmosphere and is not a cause of drug reactions we used the molar absorptivity at the maximal absorption between 290 and 400 nm as a descriptor. Phototoxicity could be also induced by the visible spectrum (400–700 nm) of sun light but our analysis is restricted to the UV range due to the unavailability of UV-vis spectra for a larger number of drugs. Considering the described approach this technical limitation is likely not problematic as most mechanisms of phototoxic drugs require the more energetic UV light.1a A closer inspection of the 409 marketed drugs with a reported UV spectra showed that the lowest ε for a phototoxic drug in the “medium/high” category is around 2540 M−1 cm−1 for COMPOUND LINKS
Read more about this on ChemSpider
Download mol file of compoundketoprofen (at the cut-off wavelength of 290 nm in COMPOUND LINKS
Read more about this on ChemSpider
Download mol file of compoundmethanol). Compounds with a ε < 2500 M−1 cm−1 have therefore not shown an associated risk of clinically relevant phototoxicity based on the known drug set. However, this cut-off also eliminates 41% (125/307) of all non-phototoxic oral drugs (Table 2). A recent study analyzing only well-known phototoxins concluded that for drugs with an absorption maxima within the 290–320 nm range and a ε > 20000 M−1 cm−1 photosensitivity reactions were significantly more frequently reported.19
Table 2 Comparison of molar absorptivity with a cut-off of 2500 M−1 cm−1 for reducing the risk of phototoxicitya,b
Prediction |
ε (M−1cm−1) |
High/medium phototoxicity |
Low phototoxicity |
Non phototoxic |
Each cell contains the number of oral drugs in the category.
See ESI for details of classification.†
|
likely |
> 2500 |
33 |
42 |
125 |
unlikely |
< 2500 |
0 |
27 |
182 |
Based on our analysis of oral drugs we came to the conclusion that compounds which have conformers with a HOMO–LUMO gap between 6.7 and 8.1 eV are predicted to have a higher risk of phototoxicity. Moreover, if such compounds are associated with molar absorptivity values above 2500 M−1 cm−1 further investigation is warranted.
It should be noted that our method tends to overpredict clinical risks of phototoxicity, particularly if a compound shows poor distribution into skin and eye or the later drug will only be given at low doses. However, the value of this tool is to provide guidance in early drug discovery on how to move to chemical space with low or no phototoxicity risks.
Application to smoothened inhibitors
Next, we tested the newly developed prediction method for a class of Smo inhibitors based on the previously published 1-piperazinyl 4-benzylphthalazine 1 which is a potent inhibitor of the Hedgehog pathway with in vivo efficacy (Fig. 1).12 This compound was tested in the in vitro 3T3 NRU phototoxicity assay and showed a positive signal. In this assay the phototoxic potential of the test compounds is determined by comparing the differences in cytotoxicity (EC50 values) of compound-treated cell cultures in the presence (+Irr) or absence (−Irr) of light. The phototoxic potential is expressed by the Photo Irritation Factor (PIF) determined by comparing the EC50 (−Irr) to the EC50 (+Irr). 1 had an EC50 (−Irr) value of 126 μM and an EC50 (+Irr) value of 8.6 μM resulting in a PIF of 15. A PIF > 5 is considered a positive phototoxic signal, a PIF > 2 or < 5 is considered “probable phototoxicity” and a PIF < 2 predicts “no phototoxicity”.10 We used this value as a starting point to reduce the phototoxicity potential of our compounds. The design of new target compounds was aimed at validating the usefulness of this method for compound optimization and therefore involved compounds covering a range in HOMO–LUMO gaps within the chemical space of Smo active compounds. Our design idea was based on the assumption that extending the sp2-conjugation in the molecule should lower the gap while truncating the bicyclic phthalazine core should increase the gap (Fig. 1). The HOMO–LUMO gap in the actual compounds 2–8 in Table 3 ranged from 7.43 eV to 8.41 eV, covering the phototoxicity prediction “likely”, “possible” and “unlikely”. The UV-visible spectra of compounds 2 to 8 showed that all of them absorb light with local absorption maxima at or above 290 nm and molar extinction coefficients > 2500 M−1 cm−1, indicating that there is an elevated potential for phototoxicity based on our analysis of marketed drugs. However, one of them (compound 4) has an ε > 20000 M−1 cm−1 indicating a higher risk of drug-induced phototoxicity.19 Testing of the compounds in the 3T3 NRU assay resulted in severe in vitro phototoxicity for compounds with a HOMO–LUMO energy gap below 7.7 eV (compounds 2, 3, 4) whereas compounds with a gap energy above 7.7 eV (compounds 5 to 8) showed no or more moderate in vitro phototoxicity. Particularly, a comparison of compound pairs 2/5 and 3/8 demonstrated that an increase in the HOMO–LUMO gap by truncating the extended aromatic system of the phthalazine core has a beneficial effect on in vitro phototoxicity. As compound 8 with a PIF of 7 despite the large HOMO–LUMO gap of 8.41 eV showed, the in silico tool is not suitable for a quantitative prediction but should be rather used for estimating a trend. Overall, the experimental data from the 3T3 NRU assay demonstrated that the HOMO–LUMO gap can successfully be used in reducing phototoxicity in an actual drug discovery program. It provided us with COMPOUND LINKS
Read more about this on ChemSpider
Download mol file of compound4,5-dimethylpyridazine (as in compound 6) and cyclopenta[d]-pyridazines (as in compounds in 7 and 8) with no or strongly reduced in vitro phototoxicity. These scaffolds were used to substitute COMPOUND LINKS
Read more about this on ChemSpider
Download mol file of compoundphthalazine in Smo inhibitors, ultimately resulting in a clinical development candidate.
Table 3 Optimizing in vitro phototoxicity using the in silico prediction tool based on HOMO–LUMO energy gaps
|
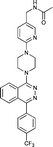
|
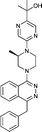
|
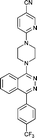
|
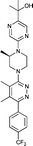
|
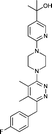
|
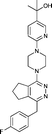
|
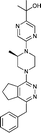
|
Compound no. |
2
|
3
|
4
|
5
|
6
|
7
|
8
|
Method of calculating the energy gap is described in the ESI.†
Details of the method of acquiring UV-visible spectra and profiling compounds in the 3T3 NRU assay are described in the ESI.†
|
HOMO–LUMO [eV]a |
7.43 |
7.51 |
7.57 |
7.79 |
7.94 |
8.11 |
8.41 |
UV
max
[nm]b |
309 |
337 |
290 |
290 |
305 |
304 |
337 |
ε [M−1 cm−1]b |
13000 |
9780 |
28400 |
6670 |
4840 |
6000 |
5260 |
PIF
(3T3 NRU)b |
> 59 |
150 |
> 760 |
18 |
1.2 |
1 |
7 |
Conclusions
We developed a simple method derived from an analysis of marketed drugs using calculated HOMO–LUMO energy gaps of the ground state and the measured UV-spectra of low molecular weight compounds to estimate the risk of drug-induced phototoxicity. The in silico component of this method can be used prospectively in the design of new compounds during phototoxicity mitigation. In combination with the UV-spectra it is useful as part of an early testing paradigm for phototoxicity and can be used to triage compounds for subsequent in vitro assays such as the 3T3 NRU phototoxicity test. We demonstrated this strategy by successfully reducing the phototoxicity risk of 1-piperazinyl-phthalazines, a class of Smo inhibitors. This optimization contributed to the discovery of a second generation smoothened inhibitor currently undergoing clinical evaluation.
Acknowledgements
The authors thank the Analytical Sciences group of NIBR, Cambridge for recording UV-visible spectra and Monika Spielmann for performing the in vitro 3T3 NRU phototoxicity assays of smoothened inhibitors 2 to 8.
Notes and references
- For reviews on drug-induced phototoxicity see:
(a) D. E. Moore, Drug Saf., 2002, 25, 345 CrossRef CAS
;
(b) K. R. Stein and N. S. Scheinfeld, Expert Opin. Drug Saf., 2007, 6, 431 CrossRef CAS
;
(c) S. Onoue, Y. Seto and S. Yamada, Curr. Drug Saf., 2009, 4, 123 CrossRef CAS
.
- J. Ferguson, Photochem. Photobiol., 1995, 62, 954 CrossRef CAS
.
- J. A. Wiebe and D. E. Moore, J. Pharm. Sci., 1977, 66, 186 CrossRef CAS
.
- I. E. Kocheva, Arch. Dermatol., 1989, 125, 824 CAS
.
- B. A. Lipsky, B. Miller, R. Schwartz, D. C. Henry, T. Nolan, A. McCabe, D. J. Magner and G. H. Talbot, Clin. Ther., 1999, 21, 675 CrossRef CAS
.
- Food and Drug Administration, Federal Reg., 1999, 64, 10944 Search PubMed
.
- J. Ferguson, Photodermatol., Photoimmunol. Photomed., 2002, 18, 262 CrossRef CAS
.
-
(a) H. W. Vohr, B. Homey, H. C. Schuppe and H. Kind, Photodermatol., Photoimmunol. Photomed., 1994, 10, 57 CAS
;
(b) P. Ulrich, B. Homey and H. W. Vohr, Toxicology, 1998, 125, 149 CrossRef CAS
;
(c) P. Ulrich, J. Streich and W. Suter, Arch. Toxicol., 2001, 74, 733 CrossRef CAS
;
(d) N. Neumann, A. Blotz, G. Wasinska-Kempka, M. Rosenbruch, P. Lehmann, A. J. Ahr and H. W. Vohr, J. Photochem. Photobiol., B, 2005, 79, 25 CrossRef CAS
.
-
(a) S. Onoue and Y. Tsuda, Pharm. Res., 2006, 23, 156 CrossRef CAS
;
(b) S. Onoue, N. Igarashi, S. Yamada and Y. Tsuda, J. Pharm. Biomed. Anal., 2008, 46, 187 CrossRef CAS
.
- H. Spielmann, M. Balls, J. Dupuis, W. J. Pape, G. Pechovitch, O. de Silva, H.-G. Holzhuetter, R. Clothier, P. Desole, F. Gerberick, M. Liebsch, W. W. Lovell, T. Maurer, U. Pfannenbecker, J. M. Potthast, M. Csato, D. Sladowski, W. Steiling and P. Brantom, Toxicol. in Vitro, 1998, 12, 305 CrossRef CAS
.
-
(a) M. D. Barratt, J. V. Castell, M. A. Miranda and J. J. Langowski, J. Photochem. Photobiol., B, 2000, 58, 54 CrossRef CAS
;
(b) M. D. Barratt, ATLA, 2004, 32, 511 CAS
.
- K. Miller-Moslin, S. Peukert, R. K. Jain, M. A. McEwan, R. Karki, L. Llamas, N. Yusuff, F. He, Y. Li, Y. Sun, M. Dai, L. Perez, W. Michael, T. Sheng, H. Lei, R. Zhang, J. Williams, A. Bourret, A. Ramamurthy, J. Yuan, R. Guo, M. Matsumoto, A. Vattay, W. Maniara, A. Amaral, M. Dorsch and J. F. Kelleher III, J. Med. Chem., 2009, 52, 3954 CrossRef CAS
.
- R. G. Pearson, Acc. Chem. Res., 1993, 26, 250 CrossRef CAS
.
-
(a) O. G. Mekenyan, G. T. Ankley, G. D. Veith and D. J. Call, SAR QSAR Environ. Res., 1994, 2, 237 CrossRef CAS
;
(b) O. G. Mekenyan, G. T. Ankley, G. D. Veith and D. J. Call, SAR QSAR Environ. Res., 1995, 4, 139 CrossRef CAS
.
- The study by Mekenyan et al., SAR and QSAR in Environmental Research 1994, 2, 237 showed that the simpler and better defined calculations for the ground states provide a similar result as calculations of the hypothetical HOMO–LUMO gap for excited states (first singlet and triplet states) and provides a thorough discussion of the various approaches.
-
(a) F. A. L. Ribeiro and M. M. C. Ferreira, THEOCHEM, 2005, 719, 191 CrossRef
;
(b) M. A. Lampi, J. Gurska, X. D. Huang, G. Dixon and B. M. Greenberg, Environ. Toxicol. Chem., 2007, 26, 406 CrossRef CAS
.
- S. Ringeissen, L. Marrot, R. Note, A. Labarussiat, S. Imbert, M. Todorov, O. Mekenyan and J. R. Meunier, Toxicol. in Vitro, 2011, 25, 324 CrossRef CAS
.
- Mekenyan et al., Chemosphere 1994, 28, 567 demonstrated in their work on the phototoxicity of PAH to aquatic organisms that AM1 and PM3 calculations for the HOMO–LUMO gap of the ground state provided the same qualitative results. In regards to model performance it is possible that an assessment of frontier orbital energy could be more accurate if using ab initio methods. However, to rapidly screen larger sets of compounds we chose a semi-empirical method.
- B. M. Verdel, P. C. Souverein, R. H. B. Meyboom, S. H. Kardaun, H. G. M. Leufkens and A. C. G. Egberts, Pharmacoepidemiol. Drug Saf., 2009, 18, 602 CrossRef CAS
.
Footnotes |
† Electronic supplementary information (ESI) available: Contents include characterization for compounds 2–8, information on the marketed oral drugs database and the classification into three phototoxicity categories, the method of calculating the HOMO–LUMO energy gap, information on the UV spectroscopic data obtained from the literature, the method of recording UV spectra and the experimental details for the 3T3 NRU phototoxicity assay. See DOI: 10.1039/c1md00144b |
‡ Present address: MIT Lincoln Laboratory, 244 Wood Street, Lexington, MA 02420, USA. E-mail: E-mail: william.egan@ll.mit.edu; Tel: (+1) 781 981 1849 |
|
This journal is © The Royal Society of Chemistry 2011 |
Click here to see how this site uses Cookies. View our privacy policy here.