DOI:
10.1039/C1MD00033K
(Concise Article)
Med. Chem. Commun., 2011,
2, 524-530
Synthesis and evaluation of protein conjugates of GM3 derivatives carrying modified sialic acids as highly immunogenic cancer vaccine candidates†
Received
3rd February 2011
, Accepted 15th March 2011
First published on 18th April 2011
Abstract
GM3, a sialylated trisaccharide antigen expressed by a number of tumors, is an attractive target in the design of therapeutic cancer vaccines. However, a serious problem associated with GM3 is that it is poorly immunogenic. To overcome this problem for the development of GM3-based cancer vaccines, four GM3 derivatives, including 5′-N-p-methylphenylacetyl, 5′-N-p-methoxyphenylacetyl, 5′-N-p-acetophenylacetyl and 5′-N-p-chlorophenylacetyl GM3, were synthesized and then coupled to a carrier protein, keyhole limpet haemocyanin (KLH). The resultant glycoconjugates were evaluated as vaccines in mouse and compared to the KLH conjugate of 5′-N-phenylacetyl GM3 (GM3NPhAc), a highly immunogenic GM3 derivative that was previously investigated as a vaccine candidate. All of the four new GM3 derivatives were proved to be more immunogenic than GM3NPhAc and elicit very strong T cell-dependent immune responses desirable for cancer immunotherapy. It was concluded that the new GM3 derivatives can form promising vaccine candidates that may be used to combine with cell glycoengineering for cancer immunotherapy.
Introduction
GM3 is a sialylated trisaccharide antigen (Fig. 1) richly expressed by a number of tumors, such as melanoma.1 Therefore, GM3 is an important molecular target in the development of novel diagnostic and therapeutic strategies for cancers, including therapeutic cancer vaccines.2–4 However, since GM3 is also expressed on some normal cells,5 though in low concentrations, as a self-antigen GM3 is poorly immunogenic and cannot elicit T cell-mediated immunity that is necessary for cancer immunotherapy. A common strategy employed to deal with this problem is covalently coupling GM3 to an immunogenic carrier protein, such as keyhole limpet haemocyanin (KLH).6 Though this strategy has resulted in vaccines that are in clinical trial,7,8 it received limited success with GM3. To overcome this problem as well as the poor immunogenicity of tumor-associated carbohydrate antigens (TACAs) as a general issue, our laboratory has recently explored a novel immunotherapeutic strategy,9–13 which combines vaccination using a synthetic vaccine derived from a TACA derivative and treatment with a sugar precursor to bioengineer the expression of the artificial TACA derivative in place of the native TACA on cancer cells. Evidently, for each such immunotherapy, there must be a vaccine made of a TACA derivative that can elicit antigen-specific T cell-mediated immune response. With this regard, we have previously designed and synthesized a series of GM3 derivatives containing unnatural sialic acids and linked them to KLH.9 Immunological studies of the conjugates showed that N-phenylacetyl GM3 (GM3NPhAc, Fig. 1) was the most immunogenic among all of the derivatives investigated thus far and that GM3NPhAc could elicit robust T cell-mediated immune response.9 Consequently, GM3NPhAc was proved to be a promising vaccine candidate.
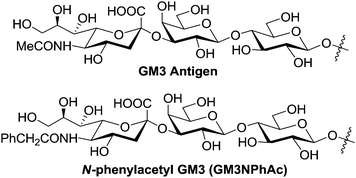 |
| Fig. 1 The structures of GM3 and GM3NPhAc. | |
The present work aims to investigate the influence of further chemical modifications of the sialic acid residue of GM3NPhAc on its immunological properties and identify derivatives that can generate more potent vaccines. For this purpose, we designed and synthesized four novel 5′-N-p-substituted phenylacetyl derivatives of GM3 antigen, including 5′-N-p-methylphenylacetyl GM3 (GM3NMePhAc), 5′-N-p-methoxyphenylacetyl GM3 (GM3NMeOPhAc), 5′-N-p-acetophenylacetyl GM3 (GM3NAcPhAc) and 5′-N-p-chlorophenylacetyl GM3 (GM3NClPhAc), coupled them to KLH to form conjugates 1b–e (Fig. 2), and examined the immunological properties of 1b–e in mouse. The newly designed GM3 derivatives contain a weakly electron-donating methyl group, a strongly electron-donating methoxyl group, a strongly electron-withdrawing acetyl group and a mildly electron-withdrawing chlorine atom attached to the phenyl group of GM3NPhAc, respectively. In the meanwhile, we also synthesized the corresponding human serum album (HSA) conjugates 2b–e and used them as coating antigens in the evaluation of immune responses elicited by 1b–e. The KLH and HSA conjugates of GM3NPhAc, 1a and 2a which were previously described,9 were used as controls in this research.
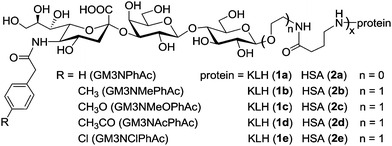 |
| Fig. 2 Structures of the GM3 derivatives investigated and their protein conjugates. | |
Results and discussion
Synthesis of the designed GM3 derivatives and their protein conjugates
Synthesis of the designed GM3 derivatives and their protein conjugates 1b–e and 2b–e is outlined in Scheme 1. After glycosyl donor 3 and acceptor 4 were prepared according to a reported procedure,9 their glycosylation reaction was carried out by Boons' protocol14 to afford the desired α-linked trisaccharide 5 in a good yield (63%). The α-configuration of COMPOUND LINKS
Read more about this on ChemSpider
Download mol file of compoundsialic acid in 5 was determined by its uniquely downfield-shifted NMR signal of H-3e (δ 2.69, compared to δ 2.42 of β-linked COMPOUND LINKS
Read more about this on ChemSpider
Download mol file of compoundsialic acid) and by comparison of the NMR spectra of 5 with the reported.9 Thereafter, a pentenoyl linker employed to couple the antigens to carrier proteins was introduced upon reduction of the azido group in 5 to form a primary amine and selective acylation of the amino group with COMPOUND LINKS
Read more about this on ChemSpider
Download mol file of compoundpentenoic anhydride. Then, the product 6 was treated with a 0.5 N aq. solution of COMPOUND LINKS
Read more about this on ChemSpider
Download mol file of compoundNaOH to remove all protecting groups including the N-trifluoroacetyl group. The exposed amino group was selectively acylated in COMPOUND LINKS
Read more about this on ChemSpider
Download mol file of compoundMeOH by reacting with p-methylphenylacetic, p-methoxyphenylacetic, p-acetophenylacetic and p-chlorophenylacetic anhydrides, respectively, to give the GM3 derivatives 7b–e. These products were purified on a Biogel P2 column using deionized COMPOUND LINKS
Read more about this on ChemSpider
Download mol file of compoundwater as eluent, and the identity and purity of 7b–e were proved by NMR spectrometry and HR MS.
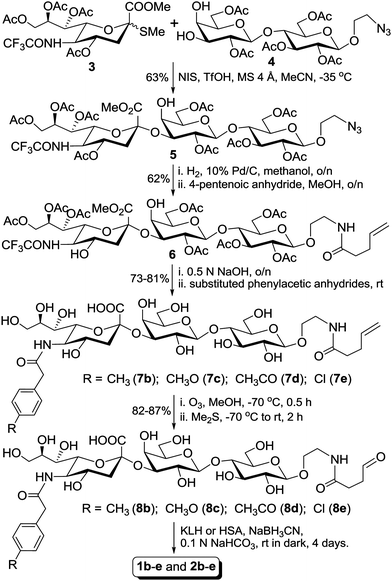 |
| Scheme 1 | |
Finally, 7b–e were linked to carrier proteins by a two-step procedure,15 including treatment of 7b–e with COMPOUND LINKS
Read more about this on ChemSpider
Download mol file of compoundozone in COMPOUND LINKS
Read more about this on ChemSpider
Download mol file of compoundmethanol to oxidize the carbon–carbon double bond of the linker and then coupling the resultant aldehydes 8b–e to KLH or HSA by reductive amination carried out in a 0.1 N NaHCO3 buffer in the presence of NaBH3CN. The aldehydes 8b–e were purified on a Biogel P2 column, and their 1H NMR spectra clearly showed the disappearance of the carbon–carbon double bond signals and appearance of the aldehyde signals at 8–9 ppm and/or the hydrated aldehyde signals at about 5.2 ppm. Glycoconjugates 1b–e and 2b–e were purified on a Biogel A0.5 column, and they were the first eluted components from the column and were easily separated from the corresponding aldehydes 8b–e that were used in excess. Column fractions that showed positive results in assays for both COMPOUND LINKS
Read more about this on ChemSpider
Download mol file of compoundsialic acid and protein were combined, dialyzed against COMPOUND LINKS
Read more about this on ChemSpider
Download mol file of compoundwater, and then freeze-dried to afford 1b–e and 2b–e as white fluffy solids. The antigen loading levels of these glycoconjugates were determined by analyzing their COMPOUND LINKS
Read more about this on ChemSpider
Download mol file of compoundsialic acid contents as described in the literature.16 It was revealed that KLH and HSA conjugates 1b–e and 2b–e contained ca. 3–5% and 6–8% (w/w) of the corresponding carbohydrate antigens, respectively, which were comparable to that obtained with 1a and 2a.9
Immunological evaluation of glycoconjugates 1a–e
KLH conjugates 1a–e were investigated as vaccines in C57BL/6 mouse, with the immune responses evaluated by enzyme-linked immunosorbent assays (ELISAs) of antigen-specific antibodies in the mouse antisera. The immunization of animals and the ELISA experiments were performed according to the protocols reported previously.9 In brief, each group of 5 mice were immunized intramuscularly (i.m.) with a total of 0.1 mL of the emulsion of 1a–e (containing 3 µg of carbohydrate antigen) and Titermax Gold adjuvant on day 0, 14, 21 and 28, respectively. Mice were bled on day −1 prior to the initial immunization and on day 27 and day 37 after immunization. Blood samples collected at each time point were clotted to prepare antisera used for ELISA. For ELISA, HSA conjugates 2a–e were utilized as capture antigens to coat plates for the detection of antibodies specific for the corresponding carbohydrate antigen, and alkaline phosphatase labeled anti-mouse kappa antibody and anti-mouse IgM and IgG antibodies were employed as the secondary antibodies for the analysis of the titers of total antibody and antibody isotypes, including IgM, IgG1, IgG2a, and IgG3 antibodies, respectively. The antibody titer, defined as the serum dilution number at which the optical density (OD) of an ELISA experiment reaches 0.5, was obtained from the best-fit line by plotting the OD values against the serum dilution numbers.
Our ELISA results revealed that the antibody titers of the day 37 antisera were globally higher than that of the day 27 antisera, reflecting the general trend of enhanced immune response to recurring exposure to the same antigen. Here, only the results of the day 37 antisera are presented and discussed in detail. Fig. 3 shows the total antibody titers of the pooled antisera of all 5 immunized mice with regard to each specific antigen. Clearly, all of the new conjugates 1b–e, no matter whether they have electron-donating groups or electron-withdrawing groups attached to the phenyl ring of the N-acyl group, elicited much higher (67–96%) antibody titers than 1a, and the differences between 1a and all of the new GM3 derivatives 1b–e were statistically very significant (P < 0.01 for the t test). These results suggest that the p-substituted phenylacetyl derivatives of GM3 antigen are more immunogenic than GM3NPhAc.
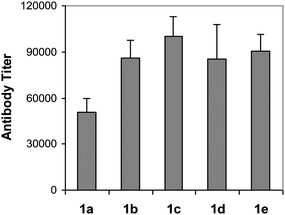 |
| Fig. 3
Antigen-specific total antibody titers in the day 37 antisera obtained from mice inoculated with conjugates 1a–e. Each column represents the average antibody titer in the pooled antisera of five replicate animals. For ELISA, the corresponding HSA conjugates 2a–e were employed as the capture antigens for plate coating, and goat anti-antibodies were used to detect antibodies bound to the plates. The antibody titer differences between all of the new GM3 derivatives 1b–e and GM3NPhAc 1a were statistically very significant (P < 0.01 for the t test). | |
The titers of various antibody isotypes in the day 37 antiserum of each immunized mouse were individually analyzed by ELISA, and the results are shown in Fig. 4. The conjugates of all four new GM3 derivatives elicited uniformly high titers of IgG1 antibodies in all of the mice, while the titers of other antibody isotypes, including IgM, IgG2a and IgG3 antibodies, were relatively low. Moreover, the IgG1 antibody titers corresponded to the total antibody titers well, which also suggested that the elicited antibody responses were mainly IgG1. The production of IgG1 antibodies is important and useful for cancer immunotherapy because this indicates T cell involvement in the immune response and potentially improved immunological memories, antibody maturation and cell-mediated immunity and cytotoxicity.12,17 These results further indicate that glycoconjugates 1b–e are promising vaccine candidates.
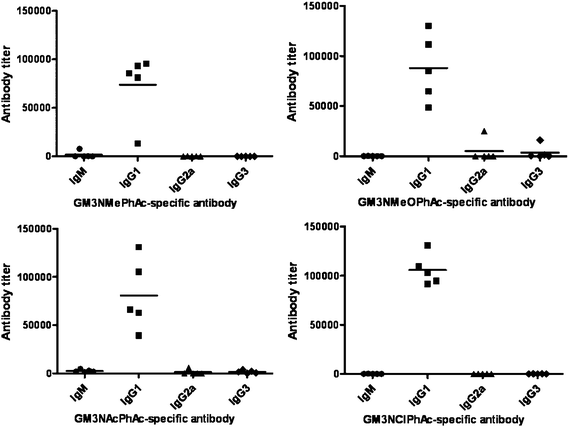 |
| Fig. 4 Titers of various isotypes of antigen-specific antibodies in the day 37 antisera obtained from mice inoculated with conjugates 1b–e, respectively, as determined by ELISA. Each dot represents the ELISA result of an individual mouse, and each black line represents the average antibody level of that group of five mice. | |
Finally, we analyzed the cross-reactivity of the antisera of 1b–e to natural GM3 and to all of the synthetic GM3 derivatives discussed above, including GM3NPhAc, GM3NMePhAc, GM3NMeOPhAc, GM3NAcPhAc and GM3NClPhAc, and the results are shown in Fig. 5. In these experiments, the ELISA plates were first coated with conjugates 2a–e and GM3-HSA, respectively, and then treated with the day 37 antisera obtained with 1b–e. All of the antisera had essentially no reactivity to natural GM3, suggesting that 1b–e would cause no or very low autoimmune reactions if utilized as cancer vaccines. On the other hand, it was found that all of the antisera had certain levels of cross-reactivity to GM3NPhAc and other non-self GM3NPhAc analogs. The highest cross-reactivity was observed with the antiserum of 1b (GM3NMePhAc) to GM3NClPhAc, the antiserum of 1c (GM3NMeOPhAc) to GM3NAcPhAc, the antiserum of 1d (GM3NAcPhAc) to GM3NMeOPhAc, and the antiserum of 1e (GM3NClPhAc) to GM3NMePhAc. These results suggest that the phenylacetyl group in GM3NPhAc and its analogs, as well as the steric bulk of the substituents attached to the phenyl group, may have played an important role in defining the immune reactions and the antibody specificity.
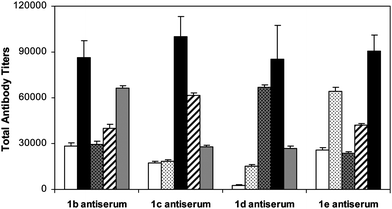 |
| Fig. 5 Titers of reactive antibodies in the day 37 antisera of 1b–e to GM3NPhAc-HSA ( , 2a), GM3NMePhAc-HSA ( , 2b), GM3NMeOPhAc-HSA ( , 2c), GM3NAcPhAc-HSA ( , 2d) and GM3NClPhAc-HSA ( , 2e), respectively. The black bars ( ) highlight titers of antibodies of the antisera reacted to self-antigens. In these experiments, total antibody titers were obtained from pooled antisera of all five mice immunized with the indicated conjugates. | |
Briefly, in this research we synthesized four new derivatives of GM3, including GM3NMePhAc, GM3NMeOPhAc, GM3NAcPhAc and GM3NClPhAc, and their KLH and HSA conjugates, 1b–e and 2b–e. The immunological properties of 1b–e were evaluated in C57BL/6 mouse and compared to that of GM3NPhAc-KLH (1a). All of the four new derivatives were more immunogenic than GM3NPhAc and elicited very strong T cell-dependent immune responses. It is interesting to notice that the induced antibodies were mainly IgG1. The elevated levels of IgG1 antibodies and relatively low levels of IgM antibodies suggest the switching of antibody production, thereby T cell-mediated immunity,12,17 which is highly desirable for cancer immunotherapy. It was further revealed that the antisera of 1b–e did not have cross-reactivity to natural GM3, but exhibited moderate to strong cross-reactivity to GM3NPhAc and other non-self GM3 analogs. These results indicate that conjugates 1b–e are promising vaccine candidates potentially useful for the novel cancer immunotherapeutic strategy that combines synthetic vaccines with cancer cell glycoengineering. The most valuable vaccine for cancer immunotherapy is to be determined after the results of ongoing in vivo studies of their antitumor activity are available. Conclusively, chemical modification of sialic acid residues in sialo-TACAs can significantly improve their immunogenicity to generate potent vaccine candidates.
Experimental section
General methods
NMR spectra were recorded on a 400 or 500 MHz instrument with chemical shifts reported in ppm (δ) in reference to Me4Si if not specified otherwise and coupling constants (J) in hertz (Hz). High resolution electron spray ionization mass spectra (HR ESI MS) were obtained with a Waters Micromass-LCTPremier-XE mass spectrometer, and COMPOUND LINKS
Read more about this on ChemSpider
Download mol file of compoundmatrix-assisted laser desorption ionization time of flight (MALDI-TOF) MS were obtained with a Bruker Ultraflex instrument. Thin layer chromatography (TLC) was performed on COMPOUND LINKS
Read more about this on ChemSpider
Download mol file of compoundsilica gel GF254 plates with detection by COMPOUND LINKS
Read more about this on ChemSpider
Download mol file of compoundphosphomolybdic acid in COMPOUND LINKS
Read more about this on ChemSpider
Download mol file of compoundEtOH or 1% H2SO4 in COMPOUND LINKS
Read more about this on ChemSpider
Download mol file of compoundEtOH. Molecular sieves were dried under high vacuum at 170–180 °C for 6–10 h just before use. Commercial anhydrous solvents and other reagents were used without further purification. GM3NPhAc conjugates 1a and 2a and the HSA conjugate of GM3 were previously prepared by our laboratory.9
2-Azidoethyl (methyl 4,7,8,9-tetra-O-acetyl-3,5-dideoxy-5-trifluoroacetamido-D-glycero-α-D-galacto-non-2-ulopyranosylonate)-(2 → 3)-(2,6-di-O-acetyl-β-D-galactopyranosyl)-(1 → 4)-2,3,6-tri-O-acetyl-D-glucopyranoside (5).
A mixture of glycosyl donor 3 (1.0 g, 1.69 mol), acceptor 4 (500 mg, 0.805 mmol), and activated molecular sieves (4 Å, 2.0 g) in anhydrous COMPOUND LINKS
Read more about this on ChemSpider
Download mol file of compoundacetonitrile (5.0 mL) was stirred at rt for 24 h under argon. After the mixture was cooled to −35 °C, NIS (764 mg, 3.38 mmol) was added, and 30 min later, COMPOUND LINKS
Read more about this on ChemSpider
Download mol file of compoundTfOH (74.5 µL, 0.17 mmol) was added. The mixture was kept at −35 °C for 1 h and then diluted with COMPOUND LINKS
Read more about this on ChemSpider
Download mol file of compounddichloromethane (DCM). The solid material was filtered off and washed with DCM. The combined filtrates were washed with aqueous NaS2O3 (20%) and COMPOUND LINKS
Read more about this on ChemSpider
Download mol file of compoundwater. The organic phase was dried over Na2SO4 and concentrated in vacuum. COMPOUND LINKS
Read more about this on ChemSpider
Download mol file of compoundSilica gel column chromatography of the residue afforded the desired trisaccharide 5 (410 mg, 63%). 1H NMR (COMPOUND LINKS
Read more about this on ChemSpider
Download mol file of compoundCDCl3, 400 MHz): δ 6.46 (d, 1H, J = 8.0 Hz, NH), 5.51–5.49 (m, 1H, H-8″), 5.36 (dd, 1H, J = 8.8, 2.4 Hz, H-7″), 5.17 (t, 1H, J = 8.8 Hz, H-2), 5.00–4.98 (m, 1H, H-2′), 4.94 (d, 1H, J = 8.8 Hz, H-1), 4.94–4.90 (m, 1H, H-4″), 4.54 (d, 1H, J = 8.4 Hz, H-1′), 4.46–4.44 (m, 1H, H-6), 4.42–4.40 (m, 1H, H-9″), 4.30–4.27 (m, 1H, H-3′), 4.23 (d, 1H, J = 6.8 Hz, H-1′), 4.19–4.15 (m, 1H, H-6), 4.08–4.04 (m, 1H, H-9″), 4.03–4.00 (m, 1H, H-5″), 3.99–3.95 (m, 1H, –OCH2CH2N3), 3.87–3.82 (m, 1H, H-3), 3.79 (s, 3H, –OCH3), 3.83–3.79 (m, 1H, H-5′), 3.70–3.68 (m, 1H, –OCH2CH2N3), 3.67–3.63 (m, 1H, H-5), 3.62–3.59 (m, 1H, H-4), 3.50–3.44 (m, 1H, –OCH2CH2N3), 3.38 (bs, 1H, H-4′), 3.29–3.25 (m, 1H, –OCH2CH2N3), 2.69 (dd, 1H, J = 9.2, 4.8 Hz, H-3″e), 2.44 (bs, 1H, OH), 2.21, 2.13, 2.09, 2.09, 2.08, 2.05, 2.03, 2.03, 2.01 (9s, 9 × 3H, OAc), 1.78–1.84 (m, 1H, H-3″a). 19F NMR (COMPOUND LINKS
Read more about this on ChemSpider
Download mol file of compoundCDCl3): δ −76.61 (s). 13C NMR (COMPOUND LINKS
Read more about this on ChemSpider
Download mol file of compoundCDCl3, 100 MHz): δ 171.2, 170.9, 170.9, 170.8, 170.7, 170.2, 170.0, 169.7, 168.4, 101.1, 100.7, 97.1, 76.5, 74.1, 73.2, 73.0, 71.9, 71.9, 71.7, 69.8, 68.8, 67.3, 67.1, 62.9, 62.4, 53.5, 50.7, 50.0, 37.8, 21.6, 21.1, 21.0, 20.9, 20.9, 20.8, 20.7. MALDI-TOF MS Calcd for C44H59F3N4NaO28 [M + Na]+: 1171.3; found: 1171.3.
N-2-O-[(Methyl 4,7,8,9-tetra-O-acetyl-3,5-dideoxy-5-trifluoroacetamido-D-glycero-α-D-galacto-non-2-ulopyranosylonate)-(2 → 3)-(2,6-di-O-acetyl-β-D-galactopyranosyl)-(1 → 4)-2,3,6-tri-O-acetyl-D-glucopyranosyl]-ethyl 4-pentenamide (6).
After a solution of 5 (140 mg, 0.122 mmol) in CH3OH (4.0 mL) was stirred with Pd/C (100 mg) under a H2 atmosphere at rt for 2 h, COMPOUND LINKS
Read more about this on ChemSpider
Download mol file of compound4-pentenoic anhydride (50 µL, 0.244 mmol) and COMPOUND LINKS
Read more about this on ChemSpider
Download mol file of compoundtriethyl amine (50 µL) were added. The mixture was stirred at rt overnight, and then the solid material was filtered off. The filtrate was condensed under reduced pressure. The residue was purified by column chromatography to afford 6 (91 mg, 62%, 2 step overall) as a white solid. 1H NMR (COMPOUND LINKS
Read more about this on ChemSpider
Download mol file of compoundCDCl3, 400 MHz): δ 6.96 (d, 1H, J = 9.6 Hz, NH), 6.00 (t, 1H, J = 5.2 Hz, NH), 5.84–5.74 (m, 1H, CH
CH2), 5.52–5.48 (m, 1H, H-8″), 5.36 (dd, 1H, J = 8.8, 2.4 Hz, H-7″), 5.15 (t, 1H, J = 9.2 Hz, H-2), 5.07–5.02 (m, 1H, H-2′), 4.98 (d, 1H, J = 8.0 Hz H-1), 4.97–4.85 (m, 1H, H-4″), 4.55 (d, 1H, J = 8.0 Hz, H-1′), 4.47–4.44 (m, 1H, H-6), 4.42–4.38 (m, 1H, H-9″), 4.27 (dd, 1H, J = 9.6, 2.4 Hz, H-3′), 4.22 (d, 1H, J = 5.6 Hz, H-1′), 4.18–4.14 (m, 1H, H-6), 4.10–4.07 (m, 1H, H-9″), 4.04–4.02 (m, 1H, H-5″), 4.01–3.94 (m, 1H, –OCH2CH2N3), 3.83–3.78 (m, 1H, H-3), 3.77 (s, 3H, –OCH3), 3.77–3.75 (m, 1H, H-5′), 3.70–3.67 (m, 1H, –OCH2CH2N3), 3.66–3.62 (m, 1H, H-5), 3.60–3.57 (m, 1H, H-4), 3.46–3.41 (m, 1H, –OCH2CH2N3), 3.39–3.37 (m, 1H, –OCH2CH2N3), 3.37 (d, 1H, J = 3.6 Hz, H-4′), 2.71–2.66 (m, 1H, H-3″e), 2.38–2.33 (m, 2H, –CH2CH2CH
CH2), 2.26–2.24 (m, 2H, –CH2CH
CH2), 2.21, 2.12, 2.08, 2.07, 2.07, 2.04, 2.02, 2.02, 1.99 (9s, 9 × 3H, OAc), 1.78 (t, 1H, J = 12.8 Hz, H-3″a). 19F NMR (COMPOUND LINKS
Read more about this on ChemSpider
Download mol file of compoundCDCl3): δ −76.58 (s). 13C NMR (COMPOUND LINKS
Read more about this on ChemSpider
Download mol file of compoundCDCl3, 100 MHz): δ 172.4, 170.7, 170.6, 170.5, 170.4, 169.8, 169.8, 169.6, 169.4, 168.1, 136.8, 76.0, 73.7, 72.9, 72.8, 71.6, 69.5, 69.2, 68.3, 68.0, 67.0, 66.8, 62.6, 62.1, 53.2, 49.7, 39.1, 37.5, 35.6, 29.5, 21.3, 20.8, 20.7, 20.6, 20.5, 20.5, HR ESI MS (m/z) Calcd. for C49H67F3N2NaO29 [M + Na]+: 1227.3679; found: 1227.3717.
General procedure for the synthesis of compounds 7b–e
Compound 6 (30 mg, 0.025 mmol) was dissolved in 0.5 N aq. COMPOUND LINKS
Read more about this on ChemSpider
Download mol file of compoundNaOH (2.0 mL), and the solution was stirred at rt for 10 h. After neutralization and condensation under reduced pressure, the crude product was directly used for the acylation. To a solution of the resultant amine (20 mg, 0.03 mmol) in 2.5 mL of COMPOUND LINKS
Read more about this on ChemSpider
Download mol file of compoundMeOH and 0.5 mL of COMPOUND LINKS
Read more about this on ChemSpider
Download mol file of compoundNaOH (0.5 N) was added 0.1 mL of an acyl anhydride dropwise in an ice-water bath. After the reaction is finished (in 6 h) as indicated by TLC, the mixture was condensed under reduced pressure. The residue was purified on a Biogel P-2 column with COMPOUND LINKS
Read more about this on ChemSpider
Download mol file of compoundH2O as the eluent. Fractions containing the expected product were combined and freeze-dried to afford compound 7b–e after lyophilization as a white solid.
N-2-O-[3,5-Dideoxy-5-(p-methylphenylacetamido)-D-glycero-α-D-galacto-2-nonulopyranosylonic acid]-(2 → 3)-β-D-galactopyranosyl-(1 → 4)-D-glucopyranosyl-ethyl 4-pentenamide (7b).
1H NMR (D2O, 400 MHz): δ 7.23–7.21 (m, 4H, aromatic H), 5.86–5.76 (m, 1H, CH
CH2), 5.08–5.00 (m, 2H, CH
CH2), 4.50–4.46 (m, 2H), 4.08–4.06 (m, 2H), 3.97–3.90 (m, 3H), 3.83–3.37 (m, 20H), 3.32–3.27 (m, 2H), 2.73 (dd, 1H, J = 12.0, 4.0 Hz, H-3″e), 2.33–2.31 (m, 4H, COCH2CH2CH
CH2), 2.30 (s, 3H, PhCH3), 1.76 (t, 1H, J = 12.0 Hz, H-3″a). 13C NMR (D2O, 100 MHz): δ 176.7, 176.1, 174.1, 137.8, 137.3, 132.2, 129.8, 129.3, 115.9, 102.9, 102.5, 100.0, 78.5, 74.5, 73.1, 73.1, 72.1, 69.6, 68.9, 68.4, 68.4, 67.7, 62.9, 61.3, 60.3, 52.0, 42.5, 40.0, 39.5, 35.3, 29.6, 20.4. HR ESI MS (m/z) Calcd. for C37H55N2O20 [M − H]+: 847.3348; found: 847.3332.
N-2-O-[3,5-Dideoxy-5-(p-methoxyphenylacetamido)-D-glycero-α-D-galacto-2-nonulopyranosylonic acid]-(2 → 3)-β-D-galactopyranosyl-(1 → 4)-D-glucopyranosyl-ethyl 4-pentenamide (7c).
1H NMR (D2O, 400 MHz): δ 7.25 (d, 2H, J = 8.8 Hz, aromatic H), 6.97 (d, 2H, J = 8.0 Hz, aromatic H), 5.86–5.79 (m, 1H, CH
CH2), 5.09–5.00 (m, 2H, CH
CH2), 4.68–4.48 (m, 2H), 4.09–4.05 (m, 1H), 3.98–3.90 (m, 2H), 3.81 (s, 3H, PhOCH3), 3.79–3.27 (m, 24H), 2.73 (dd, 1H, J = 12.0, 4.0 Hz, H-3″e), 2.35–2.31 (m, 4H, COCH2CH2CH
CH2), 1.76 (t, 1H, J = 12.0 Hz, H-3′a). 13C NMR (D2O, 100 MHz): 176.7, 176.2, 174.2, 158.3, 137.3, 130.6, 127.9, 115.9, 114.7, 103.0, 102.6, 100.1, 78.6, 74.6, 73.2, 73.1, 72.1, 69.7, 68.9, 68.5, 68.4, 67.7, 63.0, 61.3, 60.3, 55.7, 52.0, 42.0, 40.1, 39.5, 35.3, 29.6. HR ESI MS (m/z) Calcd. for C37H55N2O21 [M − H]+: 863.3297; found: 863.3317.
N-2-O-[5-(p-Acetophenylacetamido)-3,5-dideoxy-D-glycero-α-D-galacto-2-nonulopyranosylonic acid]-(2 → 3)-β-D-galactopyranosyl-(1 → 4)-D-glucopyranosyl-ethyl 4-pentenamide (7d).
1H NMR (D2O, 400 MHz): δ 7.97 (d, 2H, J = 8.0 Hz, aromatic H), 7.45 (d, 2H, J = 8.0 Hz, aromatic H), 5.88–5.80 (m, 1H, CH
CH2), 5.09–5.01 (m, 2H, CH
CH2), 4.50–4.46 (m, 2H), 4.10–4.06 (m, 1H), 3.98–3.93 (m, 3H), 3.86–3.28 (m, 24H), 2.74 (dd, 1H, J = 12.0, 4.0 Hz, H-3″e), 2.65 (s, 3H, COCH3), 2.34–2.33 (m, 4H, COCH2CH2CH
CH2), 1.78 (t, 1H, J = 12.0 Hz, H-3′a). 13C NMR (D2O, 100 MHz): δ 203.9, 176.8, 175.0, 174.2, 141.6, 137.4, 135.7, 129.8, 129.4, 115.9, 103.0, 102.6, 78.6, 74.6, 73.1, 72.1, 69.7, 68.9, 68.5, 68.4, 67.8, 62.9, 61.3, 60.4, 52.1, 42.9, 40.1, 39.5, 35.3, 29.7, 26.6. HR ESI MS (m/z) Calcd. for C38H55N2O21 [M − H]+: 875.3297; found: 875.3315.
N-2-O-[5-(p-Chlorophenylacetamido)-3,5-dideoxy-D-glycero-α-D-galacto-2-nonulopyranosylonic acid]-(2 → 3)-β-D-galactopyranosyl-(1 → 4)-D-glucopyranosyl-ethyl 4-pentenamide (7e).
1H NMR (D2O, 400 MHz): δ 7.29 (d, 2H, J = 8.8 Hz, aromatic H), 7.01 (d, 2H, J = 9.2 Hz, aromatic H), 5.92–5.83 (m, 1H, CH
CH2), 5.12–5.04 (m, 2H, CH
CH2), 4.53–4.48 (m, 2H), 4.12–4.09 (m, 1H), 4.01–3.94 (m, 3H), 3.87–3.31 (m, 21H), 2.77 (dd, 1H, J = 12.0, 4.8 Hz, H-3″e), 2.37–2.35 (m, 4H, COCH2CH2CH
CH2), 1.80 (t, 1H, J = 12.0 Hz, H-3′a). 13C NMR (D2O, 100 MHz): δ 176.7, 175.5, 174.2, 137.3, 133.9, 132.8, 130.9, 129.1, 115.9, 102.9, 102.5, 100.1, 78.5, 74.6, 73.1, 72.1, 69.6, 68.9, 68.5, 68.4, 67.7, 62.9, 61.3, 60.3, 52.0, 42.2, 40.1, 39.5, 35.3, 29.6. HR ESI MS (m/z) Calcd. for C36H52ClN2O20 [M − H]+: 867.2802; found: 867.2805.
General procedure for the synthesis of compounds 8b–e
To a stirred solution of 7b–e (18 mg) in COMPOUND LINKS
Read more about this on ChemSpider
Download mol file of compoundMeOH (5 mL) at −78 °C, COMPOUND LINKS
Read more about this on ChemSpider
Download mol file of compoundozone was bubbled until a blue color appeared and remained at −78 °C for 0.5 h. After introducing nitrogen to remove the remaining ozone, Me2S (0.5 mL) was added at −78 °C. The resultant solutions were allowed to warm to rt over a period of 1 h and stand for another 1 h before they were condensed in vacuum. The crude products were purified by a Biogel P-2 column using distilled COMPOUND LINKS
Read more about this on ChemSpider
Download mol file of compoundwater as the eluent to give aldehydes 8b–e after lyophilization as white solids, which were used in the following conjugation reactions without further purification.
N-2-O-[3,5-Dideoxy-5-(p-methylphenylacetamido)-D-glycero-α-D-galacto-2-nonulopyranosylonic acid]-(2 → 3)-β-D-galactopyranosyl-(1 → 4)-D-glucopyranosyl-ethyl 4-oxo-butanamide (8b).
1H NMR (D2O, 400 MHz): δ 8.46 (bs, 1H, –CHO), 7.24–7.22 (m, 4H, aromatic H), 4.50–4.42 (m, 2H), 4.11–4.06 (m, 1H), 3.95–3.86 (m, 2H), 3.76–3.50 (m, 16H), 3.38–3.33 (m, 2H), 2.75 (dd, 1H, J = 12.0, 4.8 Hz, H-3″e), 2.46–2.40 (m, 4H, COCH2CH2CHO), 2.33 (s, 3H, PhCH3), 1.79 (t, J = 12.0 Hz, 1H, H-3′a). HR ESI MS (m/z) Calcd. for C36H53N2O21 [M − H]+: 849.3141; found: 849.3170.
N-2-O-[3,5-Dideoxy-5-(p-methoxyphenylacetamido)-D-glycero-α-D-galacto-2-nonulopyranosylonic acid]-(2 → 3)-β-D-galactopyranosyl-(1 → 4)-D-glucopyranosyl-ethyl 4-oxo-butanamide (8c).
1H NMR (D2O, 500 MHz): δ 8.39 (s, 1H, –CHO), 7.18 (d, J = 6.8 Hz, 2H, aromatic H), 6.90 (d, 2H, J = 6.8 Hz, aromatic H), 4.44–4.37 (m, 3H), 4.02–3.84 (m, 7H), 3.73 (s, 3H, OCH3), 3.71–3.38 (m, 33H), 2.65 (dd, 1H, J = 10.0, 3.6 Hz, H-3″e), 2.52–2.30 (m, 4H, COCH2CH2CHO), 1.68 (t, J = 9.2 Hz, 1H, H-3′a). HR ESI MS (m/z) Calcd. for C36H53N2O22 [M − H]+: 865.3090; found: 865.3101.
N-2-O-[5-(p-Acetophenylacetamido)-3,5-Dideoxy-D-glycero-α-D-galacto-2-nonulopyranosylonic acid]-(2 → 3)-β-D-galactopyranosyl-(1 → 4)-D-glucopyranosyl-ethyl 4-oxo-butanamide (8d).
1H NMR (D2O, 500 MHz): δ 8.35 (s, 1H, –CHO), 7.89 (d, 2H, J = 6.4 Hz, aromatic H), 7.37 (d, 2H, J = 6.8 Hz, aromatic H), 4.42–4.38 (m, 2H), 4.01–3.99 (m, 1H), 3.89–3.85 (m, 3H), 3.77–3.21 (m, 23H), 2.66 (dd, 1H, J = 12.0, 4.5 Hz, H-3″e), 2.57 (s, 3H, COCH3), 2.38–2.35 (m, 4H, COCH2CH2CHO), 1.69 (t, 1H, J = 12.0 Hz, H-3′a). MALDI-TOF MS (m/z) Calcd. for C37H54N2NaO22 [M + Na]+: 901.3; found: 901.3.
N-2-O-[5-(p-Chlorophenylacetamido)-3,5-dideoxy-D-glycero-α-D-galacto-2-nonulopyranosylonic acid]-(2 → 3)-β-D-galactopyranosyl-(1 → 4)-D-glucopyranosyl-ethyl 4-oxo-butanamide (8e).
1H NMR (D2O, 400 MHz): δ 8.45 (bs, 1H, –CHO), 7.41 (d, 2H, J = 8.0 Hz, aromatic H), 7.30 (d, 2H, J = 8.8 Hz, aromatic H), 4.70–4.47 (m, 2H), 4.01–3.99 (m, 1H), 3.89–3.85 (m, 3H), 3.77–3.21 (m, 23H), 3.96–3.52 (m, 21H), 3.35–3.33 (m, 2H), 2.76 (dd, 1H, J = 12.4, 4.8 Hz, H-3″e), 2.47–2.40 (m, 4H, COCH2CH2CHO), 1.79 (t, 1H, J = 12.0 Hz, H-3′a). HR ESI MS (m/z) Calcd. for C36H50ClN2O21 [M − H]+: 869.2595; found: 869.2624.
Procedure for the coupling between 8b–e and KLH or HSA
The solutions of 8b–e (7 mg), KLH or HSA (7 mg), and NaBH3CN (7 mg) in 0.1 M aq. NaHCO3 (0.1 mL, pH 7.5–8.0) were allowed to stand at rt in the dark for 4 days with occasional shaking. The reaction mixtures were then purified by a Biogel A 0.5 column using 0.1 M phosphate buffered saline (PBS) buffer (I = 0.1, pH = 7.8) as the eluent. The fractions containing the glycoconjugates, characterized by bicinchoninic acid (BCA) assay for proteins, were combined and dialyzed against distilled COMPOUND LINKS
Read more about this on ChemSpider
Download mol file of compoundwater for 2 days. They were lyophilized to give white powders of the expected glycoconjugates 1b–e and 2b–e (∼6 to 7 mg).
Analysis of the carbohydrate loading levels of the glycoconjugates 1b–e and 2b–e16
The solution of an exactly weighed glycoconjugate (0.35–0.6 mg) in distilled COMPOUND LINKS
Read more about this on ChemSpider
Download mol file of compoundwater (1.0 mL) was mixed with the resorcinol reagent (2.0 mL) and the mixture was heated in a boiling water bath for 30 min. After it was cooled to rt, was added an extraction solution (1-butanol acetate and COMPOUND LINKS
Read more about this on ChemSpider
Download mol file of compound1-butanol, 85
:
15 v/v, 3.0 mL). The mixture was shaken vigorously before it was allowed to stand still for ca. 10 min to allow the organic layer to separate well from the inorganic layer. The organic layer was transferred to a 1.0 cm cuvette, and its absorbance at 580 nm was determined by an UV-Vis spectrometer, using a blank extraction solution as the control. The COMPOUND LINKS
Read more about this on ChemSpider
Download mol file of compoundsialic acid content of the glycoconjugates was determined against a calibration curve created with the solution of an individual standard NeuNPhAc derivative analyzed under the same condition. The carbohydrate loading of each glycoconjugate was calculated according to the following equation.
Immunization of mouse
A total of 0.1 mL of the emulsion of 1a–e (containing 3 µg of carbohydrate antigen) and Titermax Gold adjuvant (Sigma Chemical, St Louis, MO) was intramuscularly injected to each group of five female C57BL/6 mice at the age of 6–8 weeks (Jackson Laboratories, Bar Harbor, ME) on day 0, 14, 21 and 28, respectively. The mice were bled prior to the initial immunization on day −1 and after immunization on day 27 and day 37. Blood samples collected at each time point were clotted to obtain antisera and stored at −80 °C before assays. All experiments with live animals were performed in compliance with the relevant laws and institutional guidelines, and the Institutional Animal Care and Use Committee of Wayne State University has approved the procedures (protocol number: A05-02-08) involved in the experiments.
Enzyme-linked immunosorbent assay (ELISA)
ELISA plates were treated respectively with 100 µL solution of conjugates 2a–e and GM3-HSA (2 µg mL−1) in the coating buffer (0.1 M bicarbonate, pH 9.6) overnight at 4 °C, followed by washing 3 times with PBS containing 0.05% Tween-20 (PBST). Individual or pooled antisera from mice inoculated with 1a–e were diluted 1
:
300 to 1
:
72
900 in serial half-log dilutions in PBS and incubated for 2 h at 37 °C in the coated ELISA plates 100 µL well−1. The plates were washed and then incubated with 1
:
1000 dilution of alkaline phosphatase linked goat anti-mouse kappa, IgM or IgG2a antibody or with 1
:
2000 dilution of alkaline phosphatase linked goat anti-mouse IgG1 or IgG3 antibody for 1 h at rt. Finally, the plates were washed and developed with 100 µL of PNPP solution (1.67 mg mL−1 in PNPP buffer) for 30 min at rt for colorimetric readout using a BioRad 550 plate reader at 405 nm wavelength. For titer analysis, optical density (OD) values were plotted against dilution numbers, and a best-fit line was obtained. The equation of this line was used to calculate the dilution number at which an OD value of 0.5 was achieved, and the antibody titer was calculated at the inverse of this dilution number.
Acknowledgements
This work was supported in parts by research grants from National Institutes of Health of USA (R01 CA95142) and National Natural Science Foundation of China (30728032).
References
- T. Tsuchida, R. E. Saxton, D. L. Morton and R. F. Irie, J. Natl. Cancer Inst., 1987, 78, 45–54 CAS.
- S. Hakomori and Y. Zhang, Chem. Biol., 1997, 3, 97–104 CrossRef.
- S. Hakomori, Adv. Exp. Med. Biol., 2001, 491, 369–402 CAS.
- R. J. Bitton, M. D. Guthmann, M. R. Babri, A. J. L. Carnero, D. F. Alonso, L. Fainboim and D. E. Gomez, Oncol. Rep., 2002, 9, 267–276 CAS.
- G. A. Nores, T. Dohi, M. Taniguchi and S. Hakomori, J. Immunol., 1987, 139, 3171–3176 CAS.
- P. O. Livingston, Immunol. Rev., 1995, 145, 147–156 CAS.
- D. Stipp, Fortune, 1998, 138, 168–176 Search PubMed.
- S. J. Danishefsky and J. R. Allen, Angew. Chem., Int. Ed., 2000, 39, 837–863 CrossRef CAS.
- Y. B. Pan, P. Chefalo, N. Nagy, C. V. Harding and Z. Guo, J. Med. Chem., 2005, 48, 875–883 CrossRef CAS.
- T. Liu, Z. Guo, Q. Yang, S. Sad and H. J. Jennings, J. Biol. Chem., 2000, 275, 32832–32836 CrossRef CAS.
- J. Wu and Z. Guo, Bioconjugate Chem., 2006, 17, 1537–1544 CrossRef CAS.
- Q. Wang, J. Zhang and Z. Guo, Bioorg. Med. Chem., 2007, 15, 7561–7567 CrossRef CAS.
- Q. Wang and Z. Guo, Curr. Opin. Chem. Biol., 2009, 13, 608–617 CrossRef CAS.
- C. D. Meo, A. V. Demchenko and G. J. Boons, J. Org. Chem., 2001, 66, 5490–5497 CrossRef CAS.
- J. Xue, Y. B. Pan and Z. W. Guo, Tetrahedron Lett., 2002, 43, 1599–1602 CrossRef CAS.
- L. Svennerholm, Biochim. Biophys. Acta, 1957, 24, 604–611 CrossRef CAS.
- A. Casadevall and L. A. Pirofski, Trends Immunol., 2003, 24, 474–478 CrossRef CAS.
|
This journal is © The Royal Society of Chemistry 2011 |