DOI:
10.1039/C0MD00247J
(Concise Article)
Med. Chem. Commun., 2011,
2, 308-314
Structure and antimicrobial properties of multivalent short peptides†
Received
3rd December 2010
, Accepted 18th January 2011
First published on 28th February 2011
Introduction
The emergence of multidrug-resistant (MDR) strains1–5 continues to threaten human health.6–8 Within the past 60 years, resistance to almost every widely used commercial antibiotic in either community-associated or hospital-acquired strains has appeared shortly after their introduction.9–11 The rapid acquisition of resistance reflects the tremendous diversity of resistance genes present in a population such as that of the human microbiome.12 Discovering new classes of antibiotics able to overcome MDR bacteria may thus entail a search for rare targets able to evade this enormous pool.13 Faced with this situation, peptides that are secreted in the host defense of many organisms, antimicrobial peptides or AMPs, have been considered as a possible class of antibiotics for treating infectious diseases.14–17
A wide variety of naturally occurring antimicrobial peptides (AMPs) are expressed in both the animal and plant kingdoms;18–22 they act in the innate and acquired immunity of vertebrates and are part of the humoral defense of invertebrates against infections from pathogenic microorganisms.23–26 Many efforts have been directed to develop natural AMPs as new antibacterial drugs14–17 with limited success to date.15 The main problems are that they are hemolytic, expensive to produce, and not orally bioavailable. Recently we described the design of cationic antimicrobial peptides with repeating sequences of COMPOUND LINKS
Read more about this on ChemSpider
Download mol file of compoundarginine and COMPOUND LINKS
Read more about this on ChemSpider
Download mol file of compoundtryptophan, (RW)n,27 based on the observation that short chains of R and W comprise a pharmacophore for AMPs derived from the natural COMPOUND LINKS
Read more about this on ChemSpider
Download mol file of compoundAMP.28,29 Our previous studies have demonstrated enhanced anti-bacterial activity by multivalent displays of dipeptides or tetrapeptides on different scaffolds.30,31 Natural AMPs such as polymyxins or gramicidins tend to display high antimicrobial potency, but cytotoxicity to the host cells. In this study, we report on the antimicrobial properties of multivalent displays of short peptide sequences (Fig. 1). These include multivalent dendrimeric displays with different sequences, repeats and number of branches, brush-like structures such as Ac-[GK(RWRW)]4/8-NH2, in which RWRW tetrapeptides are grafted onto linear scaffolds Ac-(GK)4/8-NH2, and polymeric displays, PMA-RWRW, constructed by linking various ratios of the tetrapeptide RWRW to polymaleic anhydride (PMA). As judged from values of the hemolytic index (HI) (HI is the ratio of HD50 to IC50; HD50, is the concentration of agent that lyses 50% red blood cells and IC50, the concentration of the agent that inhibit 50% of bacterial growth), (RW)4D emerged as the optimal anti-bacterial agent (Fig. 2). Further resistance studies show that the dendrimer (RW)4D and natural indolicidin from bovine neutrophils offer clear advantages over conventional antibiotics or disinfectants such as COMPOUND LINKS
Read more about this on ChemSpider
Download mol file of compoundciprofloxacin, COMPOUND LINKS
Read more about this on ChemSpider
Download mol file of compoundvancomycin, COMPOUND LINKS
Read more about this on ChemSpider
Download mol file of compoundchlorhexidine and gentamicin, against MDR bacterial strains tested. Both (RW)4D and indolicidin induce extremely low levels of bacterial resistance after 400 generations of growth relative to the conventional drugs tested (Fig. 3). The dendrimer is less cytotoxic than indolicidin, and more potent as an antimicrobial.
![(A) Synthetic scheme for constructing polydisperse multivalent PMA-RWRWs based on RWRW and PMA. Unreacted groups on the polymer are capped by carboxymethylation. (B) Structure of PMA-RWRWs with different grafting ratios. (C) Structures of Ac-[GK(RWRW)4/8]-NH2. (D) Multivalent dendrimer peptides in this study. (E) The structure of (RW)4D as a representative; structures of other dendrimer peptides are displayed with different sequences/repeats and number of branches as shown in (D).](/image/article/2011/MD/c0md00247j/c0md00247j-f1.gif) |
| Fig. 1
(A) Synthetic scheme for constructing polydisperse multivalent PMA-RWRWs based on RWRW and PMA. Unreacted groups on the polymer are capped by carboxymethylation. (B) Structure of PMA-RWRWs with different grafting ratios. (C) Structures of Ac-[GK(RWRW)4/8]-NH2. (D) Multivalent dendrimer peptides in this study. (E) The structure of (RW)4D as a representative; structures of other dendrimer peptides are displayed with different sequences/repeats and number of branches as shown in (D). | |
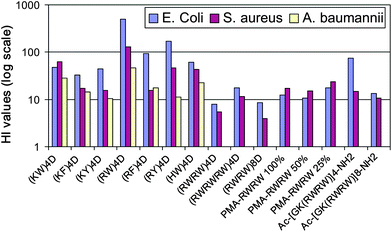 |
| Fig. 2 Comparison of hemolytic index of different multivalent AMPs. HI value is calculated as the ratio of HD50 (HI = HD50/IC50). Please note the logarithmic scale used in the plot. | |
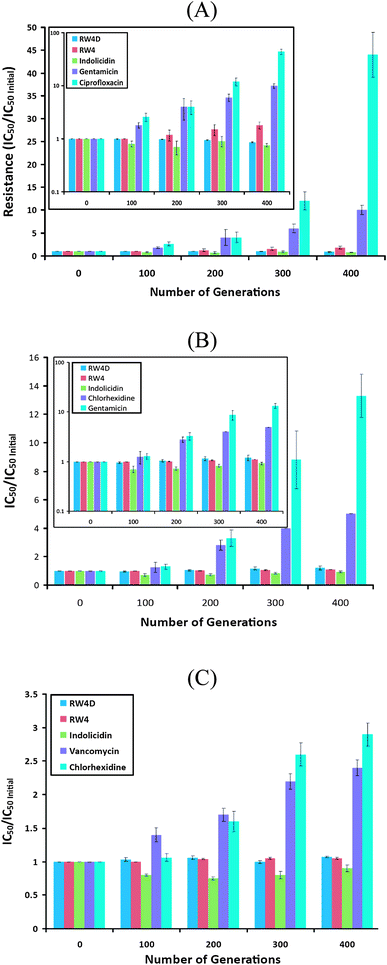 |
| Fig. 3 Development of resistance among different bacteria strains over 400 generations against (RW)4D and control antibacterial agents: (A) Gram-negative A. baumannii against (RW)4D, (RW)4, indolicidin, gentamicin and COMPOUND LINKS
Read more about this on ChemSpider
Download mol file of compoundciprofloxacin; (B) Gram-negative E. coli D31 against (RW)4D, (RW)4, indolicidin, COMPOUND LINKS
Read more about this on ChemSpider
Download mol file of compoundchlorhexidine and gentamicin; (C) Gram-positive S. aureus against (RW)4D, (RW)4, indolicidin, COMPOUND LINKS
Read more about this on ChemSpider
Download mol file of compoundvancomycin and COMPOUND LINKS
Read more about this on ChemSpider
Download mol file of compoundchlorhexidine. Note for figure 3A and 3B, the figure is re-plotted with a log scale as an inset in which the effects around the low value portion of y axis are emphasized. | |
Results
Antimicrobial activities of PMA-RWRW and Ac-[GK(RWRW)]4/8-NH2
In a previous study, we created multivalent antimicrobial agents by covalently linking antimicrobial tetrapeptides (RWRW and RRWW) onto reactive polymaleic anhydride (PMA) chains.30 In the initial study PMA chains containing ∼40 monomer units on average were fully grafted. However does the activity of multivalent COMPOUND LINKS
Read more about this on ChemSpider
Download mol file of compoundAMP require complete substitution with peptides? To answer the question, we tested 50% and 25% grafts of the same polymer, containing on average ∼20 and ∼10 RWRW units per chain, respectively (Table 1). The antibacterial activity of the latter products is roughly the same as that of the 100% grafted polymer if IC50 is expressed in micrograms per unit volume (Table 2). The fact that as few as ten RWRW units per chain retain full biological activity prompted us to investigate multivalent designs using a much shorter linear scaffold. Accordingly we constructed two multivalent AMPs Ac-[GK(RWRW)4/8]-NH2 (Fig. 1C) in which RWRW tetrapeptides were tethered to the COMPOUND LINKS
Read more about this on ChemSpider
Download mol file of compoundlysine side chains of linear scaffolds Ac-(GK)4/8-NH2. Antibacterial and hemolytic assays revealed that Ac-[GK(RWRW)4]-NH2 was more potent and less hemolytic than Ac-[GK(RWRW)8]-NH2 (Table 2). The IC50 and HD50 values showed that Ac-[GK(RWRW)4]-NH2 was more active than PMA-RWRW against Gram-negative bacteria, but less active than PMA-RWRW against Gram-positive bacteria.
Table 1 Molecular weight and monomer content in PMA-RWRW
PMA-RWRWs |
Molecular weight (Da) |
Monomer content |
Calculated |
Observed |
The peak average molecular weight (Mp).
|
PMA-RWRW (100% graft) |
33254 |
30500 |
39 |
PMA-RWRW (50% graft) |
20320 |
18600 |
19 |
PMA-RWRW (25% graft) |
13774 |
12600 |
10 |
Table 2 Summary of IC50/HD50 results for studied multivalent AMPs
Peptides |
IC50, μg mL−1 |
HD50, μg mL−1 |
E. coli |
S. aureus |
A. baumannii |
The IC50 results are the mean of three independent experiments performed in parallel.
HD50 determined from dose-response curve is peptide concentrations corresponding to 50% hemolysis.
|
(KW)4D |
30.6 |
23.3 |
52 |
1459.3 |
(KF)4D |
77 |
145 |
175 |
2500 |
(KY)4D |
84.4 |
240 |
360 |
3740 |
(RW)4D |
3.9 |
15 |
42 |
1961.8 |
(RF)4D |
30 |
180 |
160 |
2780 |
(RY)4D |
12.6 |
45.7 |
193 |
2151 |
(HW)4D |
30.6 |
43.3 |
82.7 |
1860.2 |
(RWRW)4D |
16.4 |
23.6 |
/ |
130.6 |
(RWRWRW)4D |
20.8 |
31.7 |
/ |
365.2 |
(RWRW)8D |
32.6 |
70.5 |
/ |
277.1 |
PMA-RWRW 100% |
34.1 |
24.6 |
/ |
425.7 |
PMA-RWRW 50% |
33.9 |
24.0 |
/ |
366.5 |
PMA-RWRW 25% |
34.4 |
25.0 |
/ |
598.4 |
Ac-[GK(RWRW)]4-NH2 |
15.0 |
75.4 |
/ |
1123 |
Ac-[GK(RWRW)]8-NH2 |
65.6 |
82.0 |
/ |
885.8 |
Antimicrobial activities of multivalent dendrimer peptides with different display of amino acids and different number of branches
Comparison of short linear scaffolds with dendrimers showed that the tetramer of dipeptides RW, (RW)4D, is more effective than linear constructs against both Gram-negative and Gram-positive bacteria.31 In contrast to the polymeric species, PMA-RWRW or PMA-RRWW, whose cytotoxicity increases with antimicrobial activity,30 (RW)4D has much lower hemolytic activity: its HI values are about 5 to 30 fold higher than those of PMA-RWRW/PMA-RRWW and 10 to 50 fold higher than those of indolicidin against S. aureus and E. coli, respectively.31 Analysis of the sequences of linear AMPs with extended structure reveals that they contain a high frequency of cationic and bulky non-polar amino acids, including not only COMPOUND LINKS
Read more about this on ChemSpider
Download mol file of compoundArg and Trp, but also Lys, Tyr, Phe, His and Pro.22,28,29,32–37 We therefore constructed six new dendrimers, on which different dipeptide combinations of cationic and hydrophobic amino acids are linked to a four-branched COMPOUND LINKS
Read more about this on ChemSpider
Download mol file of compoundlysine dendritic core. In addition to RW, these dipeptides include RF, RY, KW, KF, KY and HW as summarized in Fig. 1D and 1E.
The IC50 and HD50 values of these products are shown in Table 2. A general trend for the charged side chains indicates that COMPOUND LINKS
Read more about this on ChemSpider
Download mol file of compoundArg has higher activity against Gram-negative bacteria when compared to His and Lys. For the nonpolar side chains, Trp and Tyr display higher activity than Phe, with Trp being more potent than Tyr. A different trend can be seen for the hydrophobic side chains, in that all the peptides containing Trp have a low HD50 value: (KY)4D has the highest HD50 value and (KW)4D the lowest. The results indicate that (RW)4D has the best overall antimicrobial activity (Fig. 2).
While promising results are found for the dendrimer (RW)4D we need to determine the role of size of the tethered peptide units. To this end, RW, RWRW and RWRWRW were covalently attached to a four-branched COMPOUND LINKS
Read more about this on ChemSpider
Download mol file of compoundlysine dendritic core. In this set of dendrimers, the order of activities is (RWRWRW)4D < (RWRW)4D < (RW)4D; the RW dipeptide again offers the best choice in terms of antibacterial activity and selectivity (Table 2). To further test how the number of dendrimer branches affect their antibacterial activity, we constructed (RWRW)8D in which eight RWRW peptides are tethered to an eight-branched COMPOUND LINKS
Read more about this on ChemSpider
Download mol file of compoundlysine dendrimer core. It turns out (RWRW)4D is more active than (RWRW)8D against either Gram-negative or Gram-positive bacteria (Table 2). Thus unexpectedly branched arrays of relatively inert dipeptide sequences confer greater biological activity on the dendrimer product than more active tetrapeptides or hexapeptides. No linear arrangement is more active than (RW)4D.
Topological effects in multivalent scaffolds
If we compare constructs that contain the same number of RW monomers, (RWRW)4Dvs. Ac-[GK(RWRW)4]-NH2 and (RWRW)8Dvs. Ac-[GK(RWRW)8]-NH2, for example, we find that dendrimeric structures such as (RWRW)4D have a significantly lower IC50 value than Ac-[GK(RWRW)4]-NH2 for Gram-positive bacteria while the HI value for the latter is higher; dendrimeric (RWRW)8D has a significantly lower IC50 value than Ac-[GK(RWRW)8]-NH2 for Gram-negative bacteria while the HI for the latter is again higher. Comparing (RWRW)8D and Ac-[GK(RWRW)8]-NH2 to PMA-RWRW (25% graft, approximately 10 RWRW units per molecule), the relative activity for Gram-negative bacteria has the following order: Ac-[GK(RWRW)8]-NH2 < PMA-RWRW ≲ (RWRW)8D; while for Gram-positive bacteria the order is: Ac-[GK(RWRW)8]-NH2 ≲ (RWRW)8D < PMA-RWRW. The corresponding HI values are in the following order: (RWRW)8D < Ac-[GK(RWRW)8]-NH2 < PMA-RWRW. Overall PMA-RWRWs are more effective against Gram-positive relative to Gram-negative bacteria, consistent with the results for the monomeric peptide unit RWRW itself; in contrast to RWRW, Ac-[GK(RWRW)4/8]-NH2 and dendrimeric constructs are more effective against Gram-negative bacteria. Thus there appears to be no common mode of action for different constructs of monomeric peptides on scaffolds with different topology. Nevertheless, the dendrimer (RW) 4D offers the most effective display against either Gram-negative or Gram-positive bacteria as revealed by either IC50 or HI value (Table 2 and Fig. 2). Whether this is true for RW displays with different topology remains to be seen.
Since (RW)4D proved to be an effective dipeptide dendrimer, we subjected this agent to resistance studies in comparison with indolicidin, a natural antimicrobial peptide from bovine neutrophils.28 A linear analog of (RW)4D with the same composition of COMPOUND LINKS
Read more about this on ChemSpider
Download mol file of compoundArg and Trp, (RW)4 and COMPOUND LINKS
Read more about this on ChemSpider
Download mol file of compoundciprofloxacin, COMPOUND LINKS
Read more about this on ChemSpider
Download mol file of compoundvancomycin, COMPOUND LINKS
Read more about this on ChemSpider
Download mol file of compoundchlorhexidine and gentamicin were tested as controls. Acquisition of resistance to these agents was measured for two Gram-negative strains, E. coli D31 and A. baumannii, and a Gram-positive strain, S. aureus (Fig. 3). The development of resistance was monitored for 400 generations of serial transfers in the presence of sublethal concentrations of the agents.
All tested AMPs maintained potent inhibitory activity against the multi-drug resistant strains over the 400 generation period monitored. This is in marked contrast to conventional antibiotics or disinfectants. We observed a 43-fold increase in resistance of A. baumannii to COMPOUND LINKS
Read more about this on ChemSpider
Download mol file of compoundciprofloxacin, while COMPOUND LINKS
Read more about this on ChemSpider
Download mol file of compoundvancomycin resistance increased 2.5-fold in S. aureus over the time period tested. In the case of the disinfectant COMPOUND LINKS
Read more about this on ChemSpider
Download mol file of compoundchlorhexidine, a 5-fold and 2.5-fold increase in resistance of E. coli D31 and S. aureus were observed, respectively. E. coli (D31) and A. baumannii showed 13- and 8-fold increases in resistance to gentamicin, respectively. By contrast, indolicidin, (RW)4 and (RW)4D showed far lower levels of induced resistance; and (RW)4D displayed slightly lower levels of resistance as compared to its linear analog (RW)4 (Fig. 3). The levels of resistance observed demonstrate that indolicidin, (RW)4 and (RW)4D offer a clear advantage in comparison to the conventional antibiotics tested.
Discussion
Multivalent designs enhance COMPOUND LINKS
Read more about this on ChemSpider
Download mol file of compoundAMP activities, the effectiveness depending on the strain of bacteria and the structures of scaffolds
A wealth of data shows that COMPOUND LINKS
Read more about this on ChemSpider
Download mol file of compoundAMP activity is strongly concentration dependent. Formation of critically sized clusters appears essential for bacterial membrane lethality as revealed by the observation of a critical threshold concentration for known AMPs.38–40 Proposed mechanisms focus on formation of “pores” or vesicular structures that COMPOUND LINKS
Read more about this on ChemSpider
Download mol file of compoundlead finally to permeabilization of the bacterial membrane.41–45 Our motivation for multivalent designs to enhance COMPOUND LINKS
Read more about this on ChemSpider
Download mol file of compoundAMP activity originally derived from the idea that covalently pre-assembling COMPOUND LINKS
Read more about this on ChemSpider
Download mol file of compoundAMP monomers might facilitate nucleation of COMPOUND LINKS
Read more about this on ChemSpider
Download mol file of compoundAMP assemblies46–49 and thereby reduce the critical concentration for lysis. All the multivalent constructs of this study show enhanced antibacterial activity relative to the monomeric peptides.27 Another study has also reported enhanced membrane pore formation by multimeric/oligomeric antimicrobial peptide.50
A more important issue of direct relevance to the development of antibacterial drugs is whether or not a significant gain in bacterial killing effect can be achieved without increasing hemolysis. Thus PMA-RWRW's with different extents of grafting are poor candidates as antibacterial agents since hemolytic activity increases with the enhancement of antibacterial effects relative to the free monomeric peptides (Table 2). For Ac-[GK(RWRW)4/8]-NH2, IC50 and HD50 data show that Ac-[GK(RWRW)4]-NH2 might have potential as an antimicrobial agent selectively for Gram-negative bacteria. Among all the designed dendrimer AMPs of this study, (RW) 4D provides the best lead for antibacterial agent development. However we note that the effectiveness of different designed multivalent AMPs depends on the bacterial target (Gram-negative or Gram-positive), the length of the peptide, as well as the structure and topology of the scaffold. Thus shape and flexibility of scaffolds, the size and the composition of the monomeric units, and the effective valence of multivalent designs all play a role yet to be fully defined.
The group of naturally occurring AMPs related to indolicidin lacks apparent secondary structural constraints and contains a high frequency of amino acids including Trp, COMPOUND LINKS
Read more about this on ChemSpider
Download mol file of compoundArg, Lys, Tyr, Phe, His and Pro.22,34,35 Display of seven different dipeptides on the four-branched COMPOUND LINKS
Read more about this on ChemSpider
Download mol file of compoundlysine dendritic core reveals that RW offers the best choice in terms of antimicrobial activity and selectivity. This result is consistent with previous reports by Svendsen and coworkers that very short peptides-dimers and trimers based on R and W represent a minimum cationic antimicrobial pharmacophore.29 The results of this study also corroborate later findings by Hancock and coworkers.51–53 In several recent studies, they generated libraries of small cationic antimicrobial peptides either free in solution or tethered to surfaces; high-throughput screening revealed that the sequences of potent antimicrobial peptides are rich in Trp and COMPOUND LINKS
Read more about this on ChemSpider
Download mol file of compoundArg.51–53 Another study has also reported that the antimicrobial activity of peptides containing COMPOUND LINKS
Read more about this on ChemSpider
Download mol file of compoundArg is higher than that of peptides containing Lys54 although Lys tends to be less hemolytic.
Why should combinations of R and W be the most effective antimicrobial agent? Studies have shown that COMPOUND LINKS
Read more about this on ChemSpider
Download mol file of compoundArg plays a key role in membrane insertion since electrostatic interactions of the COMPOUND LINKS
Read more about this on ChemSpider
Download mol file of compoundArg side chains with the COMPOUND LINKS
Read more about this on ChemSpider
Download mol file of compoundphosphate head group serves to stabilize the peptide-membrane interaction.55 The guanidinium group of COMPOUND LINKS
Read more about this on ChemSpider
Download mol file of compoundArg has a more dispersed positive charge and higher pKa than the single amine of Lys, which may facilitate electrostatic attraction between peptides and the negatively charged bacterial surface. Trp also has a clear preference for the interfacial regions of lipid bilayers.56–59 It is not strongly hydrophobic; its extensive π-electron system is asymmetric in both shape and charge distribution. Finally the π-electron system of Trp can participate in cation-π interactions that are energetically favorable in aqueous solution allowing the residues to stabilize their interactions with the membrane.58–62
Comparing different dendrimer peptides [(RW)n]4/8D indicates there are threshold effects from the valence number and monomer charge
The activity order for dendrimers that we observe—(RWRWRW)4D < (RWRW)4D < (RW)4D and (RWRW)8D < (RWRW)4D—is notable because the observed orders differ from those seen in linear RW peptides, for which longer chains lower the IC50.27 One traditional hypothesis has been that cationic antimicrobial peptides interact electrostatically with negatively charged bacterial surfaces, such as lipopolysaccharide or lipoteichoic acid. Since bacterial killing by AMPs is cooperative we hypothesized that higher valence should increase potency. However this does not appear to be true. Instead activity might be lost because of potential immobilization of cationic peptides on the surfaces of bacteria, impeding passage to the membrane itself. Perturbation of the integrity of bacterial membrane via interactions of cationic peptides with negatively charged membrane surfaces may contribute only partially to a combination of different killing mechanisms as noted by Hancock and coworkers.63
Very slow development of resistance to (RW)4D and indolicidin suggests that surface mutations might be costly in terms of bacterial fitness
Given appropriate exposure, bacteria can evolve resistance to almost any known antibiotic or treatment.64,65 Facile gene transfer allows resistance genes to spread rapidly.4 Bacteria evade antibiotics by inactivating the drug, modifying the target, or by pumping out the drug.4,6–8 As Groisman et al. demonstrated for polymyxin resistance, COMPOUND LINKS
Read more about this on ChemSpider
Download mol file of compoundAMP resistance tends to reflect changes in surface charge rather than efflux.66 Our resistance data suggest that alterations in surface charge may be costly for fitness. However the genes that confer polymyxin resistance in Salmonella are triggered also by changes in pH and/or COMPOUND LINKS
Read more about this on ChemSpider
Download mol file of compoundMg2+ concentration.66 Thus we anticipate that resistance to agents such as (RW)4D can and will develop depending on the environment. Natural AMPs have evolved in living organisms for billions of years,14 confronted by pathogens with access to an enormous pool of resistance genes.12 One tactic to suppress resistance is to kill rapidly, even at the expense of collateral damage to host cells. This is characteristic of AMPs involved in mammalian innate immunity: high local concentrations secreted by pursuing macrophages ensure rapid inactivation of pathogens. A second strategy would be to make the evading process so costly that the survival of resistant genotypes is compromised. Recent studies have indicated that after 700 passages in the presence of increasing concentrations of AMPs, bacteria indeed develop resistance64 in contrast to previous reports by COMPOUND LINKS
Read more about this on ChemSpider
Download mol file of compoundGe et al.67 Among the mechanisms are reduction of the negative charge of LPS via transfer of amino sugars and phosphatidyl ethanolamine. The fitness cost of such modifications does not appear to impair virulence of Salmonella strains. Our conclusion that resistance to cationic (RW)4D or indolicin is much slower to develop than to conventional antibiotics does not preclude development of such resistance. Bacteria will surely evolve resistance to (RW)4D and indolicin once sufficiently stringent challenges are applied.
In summary, we have evaluated several series of multivalent AMPs as well as the natural small COMPOUND LINKS
Read more about this on ChemSpider
Download mol file of compoundAMP, indolicidin. (RW)4D demonstrates the highest level of effectiveness among the constructs of this study, being more potent and less cytotoxic than indolicidin. The results also show that under extended exposure to sublethal doses of (RW)4D and indolicidin over the 400 generations period, MDR strains elicit much lower levels of resistance than traditional antibiotics or antimicrobials such as COMPOUND LINKS
Read more about this on ChemSpider
Download mol file of compoundciprofloxacin, COMPOUND LINKS
Read more about this on ChemSpider
Download mol file of compoundvancomycin, COMPOUND LINKS
Read more about this on ChemSpider
Download mol file of compoundchlorhexidine and gentamicin.
Abbreviations list
Acknowledgements
This work was supported by a grant from ONR (N00014-03-1-0129). We acknowledge the NCRR/NIH for a Research Facilities Improvement Grant (C06 RR-16572) at NYU.
References
- B. B. Kaufmann and D. T. Hung, Mol. Cell, 2010, 37, 297–298 CrossRef CAS.
- M. A. Kohanski, M. A. DePristo and J. J. Collins, Mol. Cell, 2010, 37, 311–320 CrossRef CAS.
- K. Nagano and H. Nikaido, Proc. Natl. Acad. Sci. U. S. A., 2009, 106, 5854–5858 CrossRef CAS.
- H. Nikaido, Annu. Rev. Biochem., 2009, 78, 119–146 CrossRef CAS.
- M. A. Schumacher, K. M. Piro, W. Xu, S. Hansen, K. Lewis and R. G. Brennan, Science, 2009, 323, 396–401 CrossRef CAS.
- J. S. Blanchard, Chem. Biol., 2003, 10, 104–106 CrossRef CAS.
- S. B. Levy and B. Marshall, Nat. Med., 2004, 10, S122–S129 CrossRef CAS.
- G. D. Wright, Chem. Biol., 2000, 7, R127–R132 CrossRef CAS.
- H. F. Chambers, N. Engl. J. Med., 2005, 352, 1485–1487 CrossRef CAS.
- A. E. Clatworthy, E. Pierson and D. T. Hung, Nat. Chem. Biol., 2007, 3, 541–548 CrossRef CAS.
- A. Fajardo, J. F. Linares and J. L. Martinez, Clin. Microbiol. Infect., 2009, 15, 14–16 Search PubMed.
- M. O. A. Sommer, G. Dantas and G. M. Church, Science, 2009, 325, 1128–1131 CrossRef CAS.
- D. A. Harris, M. E. Powers and F. E. Romesberg, Bioorg. Med. Chem. Lett., 2009, 19, 3787–3790 CrossRef CAS.
- Y. J. Gordon, E. G. Romanowski and A. M. McDermott, Current Eye Research, 2005, 30, 505–515 Search PubMed.
- R. E. W. Hancock and H. G. Sahl, Nat. Biotechnol., 2006, 24, 1551–1557 CrossRef CAS.
- A. R. Koczulla and R. Bals, Drugs, 2003, 63, 389–406 CrossRef CAS.
- L. J. Zhang and T. J. Falla, Expert Opin. Pharmacother., 2006, 7, 653–663 Search PubMed.
- J. M. Conlon, J. Kolodziejek and N. Nowotny, Biochim. Biophys. Acta, Proteins Proteomics, 2004, 1696, 1–14 CrossRef CAS.
- A. J. Otero-Gonzalez, B. S. Magalhaes, M. Garcia-Villarino, C. Lopez-Abarrategui, D. A. Sousa, S. C. Dias and O. L. Franco, FASEB J., 2010, 24, 1320–1334 CrossRef CAS.
- O. Toke, Biopolymers, 2005, 80, 717–735 CrossRef CAS.
- W. van 't Hof, E. C. I. Veerman, E. J. Helmerhorst and A. V. N. Amerongen, Biol. Chem., 2001, 382, 597–619 CAS.
- M. Zasloff, Antimicrobial peptides of multicellular organisms, Nature, 2002, 415, 389–395 CrossRef CAS.
- K. L. Brown and R. E. W. Hancock, Curr. Opin. Immunol., 2006, 18, 24–30 CrossRef CAS.
- M. W. Hornef, M. J. Wick, M. Rhen and S. Normark, Nat. Immunol., 2002, 3, 1033–1040 CrossRef CAS.
- J. J. Oppenheim, A. Biragyn, L. W. Kwak and D. Yang, Ann. Rheum. Dis., 2003, 62, 17–21.
- M. E. Selsted and A. J. Ouellette, Nat. Immunol., 2005, 6, 551–557 CrossRef CAS.
- Z. G. Liu, A. Brady, A. Young, B. Rasimick, K. Chen, C. H. Zhou and N. R. Kallenbach, Antimicrob. Agents Chemother., 2007, 51, 597–603 CrossRef CAS.
- M. E. Selsted, M. J. Novotny, W. L. Morris, Y. Q. Tang, W. Smith and J. S. I. Cullor, J Biol Chem, 1992, 267, 4292–4295 CAS.
- M. B. Strom, B. E. Haug, M. L. Skar, W. Stensen, T. Stiberg and J. S. Svendsen, J. Med. Chem., 2003, 46, 1567–1570 CrossRef CAS.
- Z. G. Liu, H. Deshazer, A. J. Rice, K. Chen, C. H. Zhou and N. R. Kallenbach, J. Med. Chem., 2006, 49, 3436–3439 CrossRef CAS.
- Z. G. Liu, A. W. Young, P. Hu, A. J. Rice, C. H. Zhou, Y. K. Zhan and N. R. Kallenbach, ChemBioChem, 2007, 8, 2063–2065 CrossRef CAS.
- W. Bellamy, M. Takase, H. Wakabayashi, K. Kawase and M. Tomita, Antibacterial Spectrum of Lactoferricin-B, a Potent Bactericidal Peptide Derived from the N-Terminal Region of Bovine Lactoferrin, J Appl Bacteriol, 1992, 73, 472–479 CAS.
- S. E. Blondelle, E. Takahashi, K. T. Dinh and R. A. Houghten, J Appl Bacteriol, 1995, 78, 39–46 CAS.
- H. G. Boman, J. Intern. Med., 2003, 254, 197–215 CrossRef CAS.
- K. V. R. Reddy, R. D. Yedery and C. Aranha, Int. J. Antimicrob. Agents, 2004, 24, 536–547 CrossRef CAS.
- D. J. Schibli, P. M. Hwang and H. J. Vogel, Biochemistry, 1999, 38, 16749–16755 CrossRef CAS.
- L. W. Tinoco, A. da Silva, A. Leite, A. P. Valente and F. C. L. Almeida, J. Biol. Chem., 2002, 277, 36351–36356 CrossRef CAS.
- F. Y. Chen, M. T. Lee and H. W. Huang, Biophys. J., 2003, 84, 3751–3758 CrossRef CAS.
- M. N. Melo, R. Ferre and M. A. R. B. Castanho, Nat. Rev. Microbiol., 2009, 7, 245–250 Search PubMed.
- Y. Shai, Biopolymers, 2002, 66, 236–248 CrossRef CAS.
- K. A. Brogden, Nat. Rev. Microbiol., 2005, 3, 238–250 Search PubMed.
- M. A. Kohanski, D. J. Dwyer and J. J. Collins, Nat. Rev. Microbiol., 2010, 8, 423–435 Search PubMed.
- M. A. Kohanski, D. J. Dwyer, B. Hayete, C. A. Lawrence and J. J. Collins, Cell, 2007, 130, 797–810 CrossRef CAS.
- A. Liu, L. Tran, E. Becket, K. Lee, L. Chinn, E. Park, K. Tran and J. H. Miller, Antimicrob. Agents Chemother., 2010, 54, 1393–1403 CrossRef CAS.
- M. R. Yeaman and N. Y. Yount, Pharmacol. Rev., 2003, 55, 27–55 CrossRef CAS.
- L. L. Kiessling, L. E. Strong and J. E. Gestwicki, Annu. Rep. Med. Chem., 2000, 35, 321–330 Search PubMed.
- P. I. Kitov and D. R. Bundle, J. Am. Chem. Soc., 2003, 125, 16271–16284 CrossRef CAS.
- A. Pini, C. Falciani, E. Mantengoli, S. Bindi, J. Brunetti, S. Iozzi, G. M. Rossolini and L. Bracci, FASEB J., 2010, 24, 1015–1022 CrossRef CAS.
- J. P. Tam, Y. A. Lu and J. L. Yang, Eur. J. Biochem., 2002, 269, 923–932 CrossRef CAS.
- C. J. Arnusch, H. Branderhorst, B. de Kruijff, R. M. Liskamp, E. Breukink and R. J. Pieters, Biochemistry, 2007, 46, 13437–13442 CrossRef CAS.
- K. Hilpert, M. Elliott, H. Jenssen, J. Kindrachuk, C. D. Fjell, J. Korner, D. F. H. Winkler, L. L. Weaver, P. Henklein, A. S. Ulrich, S. H. Y. Chiang, S. W. Farmer, N. Pante, R. Volkmer and R. E. W. Hancock, Chem. Biol., 2009, 16, 58–69 CrossRef CAS.
- K. Hilpert, M. R. Elliott, R. Volkmer-Engert, P. Henklein, O. Donini, Q. Zhou, D. F. H. Winkler and R. E. W. Hancock, Chem. Biol., 2006, 13, 1101–1107 CrossRef CAS.
- K. Hilpert, R. Volkmer-Engert, T. Walter and R. E. W. Hancock, Nat. Biotechnol., 2005, 23, 1008–1012 CrossRef CAS.
- H. J. Vogel, D. J. Schibli, W. G. Jing, E. M. Lohmeier-Vogel, R. F. Epand and R. M. Epand, Biochem. Cell Biol., 2002, 80, 49–63 Search PubMed.
- F. Jean-Francois, S. Castano, B. Desbat, B. Odaert, M. Roux, M. H. Metz-Boutigue and E. J. Dufourc, Biochemistry, 2008, 47, 6394–6402 CrossRef CAS.
- J. A. Killian, I. Salemink, M. R. R. dePlanque, G. Lindblom, R. E. Koeppe and D. V. Greathouse, Biochemistry, 1996, 35, 1037–1045 CrossRef CAS.
- S. Persson, J. A. Killian and G. Lindblom, Biophys. J., 1998, 75, 1365–1371 CAS.
- W. M. Yau, W. C. Wimley, K. Gawrisch and S. H. White, Biochemistry, 1998, 37, 14713–14718 CrossRef CAS.
- M. Zorko, B. Japelj, I. Hafner-Bratkovic and R. Jerala, Biochim. Biophys. Acta, Biomembr., 2009, 1788, 314–323 CrossRef CAS.
- D. I. Chan, E. J. Prenner and H. J. Vogel, Biochim. Biophys. Acta, Biomembr., 2006, 1758, 1184–1202 CrossRef CAS.
- J. C. Ma and D. A. Dougherty, Chem. Rev., 1997, 97, 1303–1324 CrossRef CAS.
- Z. S. Shi, C. A. Olson and N. R. Kallenbach, J. Am. Chem. Soc., 2002, 124, 3284–3291 CrossRef CAS.
- M. H. Wu, E. Maier, R. Benz and R. E. W. Hancock, Biochemistry, 1999, 38, 7235–7242 CrossRef CAS.
- G. G. Perron, M. Zasloff and G. Bell, Proc. R. Soc. London, Ser. B, 2006, 273, 251–256 CrossRef CAS.
- O. Samuelsen, H. H. Haukland, H. Jenssen, M. Kramer, K. Sandvik, H. Ulvatne and L. H. Vorland, FEBS Lett., 2005, 579, 3421–3426 CrossRef CAS.
- E. A. Groisman, J. Kayser and F. C. Soncini, J Bacteriol, 1997, 179, 7040–7045 CAS.
- Y. G. Ge, D. L. MacDonald, K. J. Holroyd, C. Thornsberry, H. Wexler and M. Zasloff, Antimicrob Agents Ch, 1999, 43, 782–788 Search PubMed.
Footnote |
† Electronic supplementary information (ESI) available. See DOI: 10.1039/c0md00247j |
|
This journal is © The Royal Society of Chemistry 2011 |