DOI:
10.1039/C0MD00234H
(Concise Article)
Med. Chem. Commun., 2011,
2, 542-549
Synthesis and biological activity of hydroxybenzylidenyl pyrrolidine-2,5-dione derivatives as new potent inhibitors of tyrosinase
Received
25th November 2010
, Accepted 25th February 2011
First published on 20th April 2011
Abstract
In this study, we describe the synthesis and tyrosinase inhibitory activity of a new family of hydroxybenzylidenyl pyrrolidine-2,5-dione compounds. Among them, compound 3f (HMP) exhibited the highest inhibition, 83.87%, at a concentration of 20 μM, on the L-DOPA oxidase activity of mushroom tyrosinase. We also predicted the tertiary structure of tyrosinase, simulated its docking with HMP and confirmed that HMP strongly interacts with tyrosinase residues. This result suggested that the binding activity of HMP with tyrosinase could be high. Based on these results, we determined the IC50 value for HMP inhibition of mushroom tyrosinase activity. HMP inhibited mushroom tyrosinase with an IC50 value of 2.23 ± 0.44 μM, which is more potent than the anti-tyrosinase activity of COMPOUND LINKS
Read more about this on ChemSpider
Download mol file of compoundkojic acid (IC50 = 20.99 ± 1.80 μM), a well-known tyrosinase inhibitor. Kinetic analysis of tyrosinase inhibition revealed that HMP is a competitive inhibitor (Ki = 4.24 × 10−7 M at 1.25 μM and Ki = 1.82 × 10−6 M at 20 μM). HMP also inhibited melanin production and tyrosinase activity in B16F10 melanoma cells (B16 cells). These data strongly suggest that HMP can suppress the production of melaninvia the modulation of tyrosinase activity.
Introduction
The innermost layers of the human epidermis produce a dark pigment known as melanin. Upon exposure of the skin to UV radiation, melanogenesis is initiated through an enzyme called tyrosinase.1Tyrosinase (EC 1.14.18.1) is a multifunctional, glycosylated, copper-containing enzyme, and is found exclusively in melanocytes.2 It is therefore a good specific marker for the cells. Tyrosinase is synthesized by melanosomal ribosomes found on the rough endoplasmic reticulum.3 After biosynthesis, tyrosinase is subsequently delivered to melanosomesviacoated vesicles in an inactive form.3,4 The first two steps in the biosynthetic pathway for melanin formation are hydroxylation of COMPOUND LINKS
Read more about this on ChemSpider
Download mol file of compoundL-tyrosine to 3,4-dihyroxyphenylalanine (L-DOPA), and the oxidation of L-DOPA to O-dopaquinone.5–7 This process is a determinant of mammalian skin color and is closely related to local hyperpigmentations such as melasma, ephelide, and lentigo.8,9 It has also been suggested that tyrosinase contributes to the neurodegeneration associated with Parkinson's disease.10 Indeed, the unregulated action of tyrosinase can be a factor in a number of human disease etiologies. Thus, tyrosinase inhibition has been avidly explored as an avenue for therapies to these diseases.
Over the last few decades, a large number of naturally occurring and synthetic compounds that can act as tyrosinase inhibitors have been reported,11–19 but only a few of them are put into a practical use due to their weak activity or safety concerns.
Tyrosinase inhibitors typically either render the copper within the active site inactive by chelation, obviating the substrate–enzyme interaction, or they inhibit oxidationvia an electrochemical process.20,21 Since it has been observed that flavonoids, stilbenes, and tropolones manifest tyrosinase inhibitory activities, we sought to evaluate other phenolic skeletons for their tyrosinase inhibition properties and to elucidate their kinetic mechanisms.
In our previous studies, we reported a method of synthesis of a new family of hydroxy substituted phenyl naphthalenes as analogs of COMPOUND LINKS
Read more about this on ChemSpider
Download mol file of compoundresveratrol,22 which has the structure of hydroxy substituted COMPOUND LINKS
Read more about this on ChemSpider
Download mol file of compoundstilbene and exerts potent inhibitory effects on cyclooxygenase,23,24 rat liver mitochondrial ATPase activity,25 and L-DOPA oxidase activity.23,26 Some of these compounds suppressed the production of melaninvia the modulation of tyrosinase activity.18–22,27–31 We also found several potent tyrosinase inhibitors in natural materials22,32,33 that have an even greater potency than the well-known kojic acid.22,34
Recently, based on kinetic and active site binding studies, Ghani and Ullah suggested the mono-dentate binding of COMPOUND LINKS
Read more about this on ChemSpider
Download mol file of compoundthiadiazole, COMPOUND LINKS
Read more about this on ChemSpider
Download mol file of compoundoxadiazole, and triazole rings to the active site dicopper center of tyrosinase; hydrophobicity appeared to contribute to the potency of the inhibition.35 The most potent was the mono-dentate binding of a thiadiazole containing a 5-(4-hydroxyphenyl) substitution.35 The inhibitory activity of this compound decreased when the 4-hydroxy group on the phenyl ring was removed or the 4-hydroxyphenyl was replaced by a 5-hydroxyphenyl, reflecting the importance of the 4-hydroxy group on the phenyl ring in enhancing the inhibitory activity of mono-dentate binding of COMPOUND LINKS
Read more about this on ChemSpider
Download mol file of compoundthiadiazole.35
In the present study, we describe the synthesis and tyrosinase inhibitory activity of a new family of hydroxybenzylidenyl pyrrolidine-2,5-dione compounds. Compounds 3a, 3f (HMP) and 3l significantly inhibited tyrosinase activity. Among twelve pyrrolidine-2,5-dione compounds synthesized, HMP at a concentration of 20 μM exhibited the highest inhibition, 83.87%, of the L-DOPA oxidase activity of mushroom tyrosinase. We also predicted the tertiary structure of tyrosinase and simulated its docking with HMP. The docking simulation was successful with significant scores using DOCK6 (binding energy: −31.67 kcal mol−1) and suggested that the THR 97 residue was mainly responsible for the interaction with HMP.
Results and discussion
Hydroxybenzylidenyl pyrrolidine-2,5-dione compounds were prepared via a Wittig reaction between a variety of hydroxy substituted benzaldehydes and triphenylphosphoranylidenyl succinimide, which was synthesized from a Michael addition reaction of COMPOUND LINKS
Read more about this on ChemSpider
Download mol file of compoundtriphenylphosphine to COMPOUND LINKS
Read more about this on ChemSpider
Download mol file of compoundmaleimide. The Wittig reactions proceeded smoothly under reflux conditions in COMPOUND LINKS
Read more about this on ChemSpider
Download mol file of compoundmethanol, giving 3a–3l in 54–97.7% yields (Scheme 1 and Table 1). Only the isomers with an (E)-configuration were isolated through filtration of the precipitates formed from the Wittig reaction. The structures of the synthesized compounds were determined by 1H and 13C NMR and mass spectrometric analyses.
Table 1 The substitution pattern and inhibition of tyrosinase activity by the synthesized compounds 3a–3lb
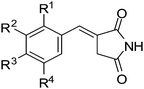
|
Compound |
R1 |
R2 |
R3 |
R4 |
Tyrosinase Inhibition (%)a |
Tyrosinase inhibition was measured using COMPOUND LINKS
Read more about this on ChemSpider
Download mol file of compoundL-tyrosine as the substrate at 20 μM.
Values represent means ± S.E. of three experiments.
|
3a
|
H |
H |
OH
|
H |
75.00 ± 0.17 |
3b
|
OH
|
H |
H |
H |
12.16 ± 0.33 |
3c
|
H |
H |
OMe
|
H |
13.43 ± 0.24 |
3d
|
H |
OH
|
OH
|
H |
14.13 ± 0.09 |
3e
|
H |
OH
|
H |
OH
|
2.70 ± 0.03 |
3f
|
H |
OH
|
OMe
|
H |
83.87 ± 0.27 |
3g
|
H |
OMe
|
OH
|
H |
18.40 ± 0.22 |
3h
|
H |
OEt
|
OH
|
H |
−0.99 ± 0.12 |
3i
|
OMe
|
H |
OMe
|
H |
9.95 ± 0.12 |
3j
|
H |
OMe
|
OMe
|
H |
33.83 ± 0.17 |
3k
|
H |
OMe
|
OH
|
OMe
|
24.86 ± 0.43 |
3l
|
H |
OMe
|
OMe
|
OMe
|
53.51 ± 0.88 |
Kojic acid
|
|
|
|
|
47.45 ± 3.17 |
The inhibitory activities of the synthesized compounds were tested on mushroom tyrosinase as described previously with minor modification.36 Inhibitory activities were compared to that of COMPOUND LINKS
Read more about this on ChemSpider
Download mol file of compoundkojic acid as a positive control. The inhibition of tyrosinase with the newly synthesized compounds is summarized in Table 1. Our results showed that compounds 3a, 3f and 3l exhibited more potent inhibition of mushroom tyrosinase than COMPOUND LINKS
Read more about this on ChemSpider
Download mol file of compoundkojic acid, which caused 47.45% inhibition at a concentration of 20 μM. As shown in Table 1, in the overall structure–activity relationship study, compound 3f bearing two substituents (the hydroxy group at position 3 and the methoxy group at position 4) on the phenyl ring was the most potent inhibitor. It is interesting to note that 3g, in which the positions of hydroxy and methoxy substituents of 3f are transposed, and 3c, in which the hydroxy group at position 3 of 3f is removed, showed remarkably decreased inhibitory activity. Compounds bearing two methoxy substituents on the phenyl ring (e.g., 3i and 3j) showed a low to moderate activity. Also, the insertion of an ethoxy group, which is larger than a methoxy group, exhibited a decrease in inhibitory activity. Compound 3b, with one hydroxyl substituent at position 2 on the phenyl ring, showed little activity. However, compound 3a, containing one hydroxy substituent at position 4, showed high inhibitory activity. Compounds bearing two hydroxyl substituents on the phenyl ring, such as 3d (containing hydroxy groups at position 3 and 4), showed weak inhibitory activity, as did 3e (containing two hydroxy groups at position 3 and 5). Compounds bearing three methoxy substituents (3l), and two methoxy substituents and one hydroxyl substituent (3k), on the phenyl ring showed moderate inhibitory activity. Our results show that the number and position of the methoxy and hydroxy groups are very closely related to the compounds ability to inhibit tyrosinase activity.
Among the newly synthesized compounds, we further investigated the bioactivities of 3f (HMP), because it exhibited the highest inhibition against mushroom tyrosinase. Mushroom tyrosinase has been widely used as a target enzyme for screening and characterizing potential tyrosinase inhibitors.37,38 The inhibitory effects of HMP and COMPOUND LINKS
Read more about this on ChemSpider
Download mol file of compoundkojic acid on mushroom tyrosinase activity are shown in Table 2. Using COMPOUND LINKS
Read more about this on ChemSpider
Download mol file of compoundL-tyrosine as the substrate, the inhibitory effects of HMP and COMPOUND LINKS
Read more about this on ChemSpider
Download mol file of compoundkojic acid on mushroom tyrosinase were determined: both inhibited mushroom tyrosinase activity in a dose-dependent manner. HMP (IC50 = 2.23 ± 0.44 μM) is a more potent inhibitor of mushroom tyrosinase than COMPOUND LINKS
Read more about this on ChemSpider
Download mol file of compoundkojic acid (IC50 = 20.99 ± 1.80 μM), the positive control.
Sample |
Conc.a (μM) |
Tyrosinase inhibition (%) |
IC50b (μM) |
Values represent means ± S.E. of three experiments.
50% inhibitory concentration (IC50).
|
COMPOUND LINKS
Read more about this on ChemSpider
Download mol file of compoundKojic acid
|
1.25 |
7.97 ± 1.06 |
20.99 ± 1.80 |
5 |
27.36 ± 1.99 |
20 |
47.45 ± 3.17 |
HMP (3f) |
1.25 |
44.27 ± 5.19 |
2.23 ± 0.44 |
5 |
60.00 ± 4.89 |
20 |
83.87 ± 0.27 |
To explore the mechanism of inhibition, a study of the kinetic behavior of tyrosinase activity was conducted in the presence of HMP; the data are shown in Fig. 1 and Table 3. The mode of inhibition of the enzyme was determined by Lineweaver–Burk plot analysis, in which the kinetics exhibited a Ki of 4.24 × 10−7 M at 1.25 μM HMP and a Ki of 1.82 × 10−6 M at 20 μM HMP. Mushroom tyrosinase activity in the presence of HMP at 1.25 and 20 μM exhibited Km values of 2.41 and 7.34 mM, respectively, and the same Vmax value of 1.88 × 10−2 mM min−1. As HMP concentrations increased, the Km value of tyrosinase increased gradually, without changing Vmax, thereby indicating that HMP acts as a competitive inhibitor of mushroom tyrosinase. In the presence of a competitive inhibitor, a higher substrate concentration is required to achieve the same velocity that was reached in its absence. So while the Vmax obtained in the absence of an inhibitor can still be reached in the presence of an inhibitor if sufficient substrate is available, one-half Vmax (i.e., Km) requires a higher substrate concentration than before and thus Km becomes larger. Tyrosinase is a copper-containing enzyme widely distributed in nature,39 and most tyrosinase inhibitors that chelate the copper in the active site of the enzyme show competitive inhibition; examples are tropolone40 and COMPOUND LINKS
Read more about this on ChemSpider
Download mol file of compoundkojic acid.41 Thus, HMP might inhibit by binding at the same site as the copper-containing active site of mushroom tyrosinase.
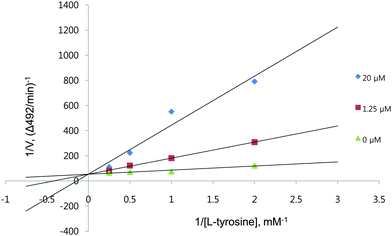 |
| Fig. 1 A Lineweaver–Burk plot of mushroom tyrosinase. Data are presented as mean values of 1/V, inverse of the increase in absorbance at a wavelength of 492 nm per min (ΔA492/min), of three independent tests with different concentrations of COMPOUND LINKS
Read more about this on ChemSpider
Download mol file of compoundL-tyrosine as a substrate. Inhibitors of the enzyme were the new compound at 1.25 μM (squares), 20.0 μM (diamonds) and no HMP (triangles). | |
Table 3 Kinetic analysis of HMP
|
V
o (mM min−1) |
V
max (mM min−1) |
K
m (mM) |
K
i (M) |
Lineweaver–Burk plot of mushroom tyrosinase activity: Data are presented as mean values of 1/V, inverse of the increase of absorbance at a wavelength 492 nm per min (ΔA492/min), of 3 independent tests with different concentrations of COMPOUND LINKS
Read more about this on ChemSpider
Download mol file of compoundL-tyrosine as a substrate. |
No inhibitor |
1.88 × 10−2 |
1.88 × 10−2 |
0.61 |
— |
1.25 μM |
9.75 × 10−6 |
1.88 × 10−2 |
2.41 |
4.24 × 10−7 |
20.0 μM |
5.11 × 10−5 |
1.88 × 10−2 |
7.34 |
1.82 × 10−6 |
To evaluate the cytotoxic effects of HMP, the murine B16F10 melanoma cell line (B16 cells) was treated with this compound. The effect of HMP on cell viability is presented in Fig. 2. At doses of 0.2, 1.0, 2.5, 5.0, and 10.0 μM HMP for 48 h of treatment, cell viability was 99.52%, 98.94%, 98.39%, 98.08%, and 97.80%, respectively, compared with a control group. Thus, HMP was not cytotoxic to B16 cells in the concentration range of 0–10 μM.
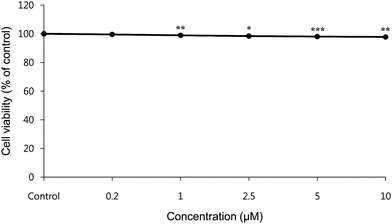 |
| Fig. 2 The Effect of HMP on the viability of B16 Cells. Cells were treated with various doses of HMP (0–10 μM) and the effect of HMP on the viability of B16 cells was examined by MTT assay. Data are expressed as % of control. *p < 0.05, **p < 0.01, ***p < 0.001, compared to the untreated control. | |
To assess the effect of HMP on melanogenesis, we quantified the melanin content of HMP-treated B16 cells. The ability of HMP to decrease melanin content is shown in Fig. 3. Melanin levels in B16 cells after treatment with HMP in the presence of 100 nM α-MSH decreased HMP dose-dependently, showing 236.67% at 0.2 μM, 231.69% at 1.0 μM, 113.11% at 2.5 μM, 103.74% at 5.0 μM, and 99.49% at 10.0 μM of HMP, compared with the group with 100 nM α-MSH only (241.87%) and a control group without α-MSH (100%). Considering the cytotoxic results shown in Fig. 2, this decrease in melanin level was due neither to cytotoxic effects nor to cell growth inhibition, implying that it was due to inhibition of tyrosinase.
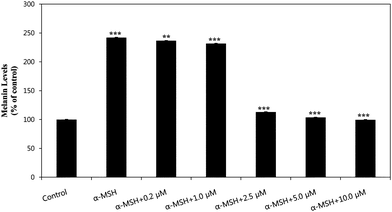 |
| Fig. 3 The ability of HMP to inhibit melanogenesis in the presence of 100 nM α-MSH in B16 Cells. Melanin levels were measured at 405 nm. Values represent the mean ± S.E. of three experiments. Data are expressed as % of control. **p < 0.01 and ***p < 0.001, compared to the group treated with 100 nM α-MSH. | |
To determine the mechanism by which HMP inhibits melanin biosynthesis, we examined the inhibitory effect of HMP on cellular tyrosinase activity using murine-derived tyrosinase, as shown in Fig. 4. After 24 h incubation with HMP, mushroom-derived tyrosinase activities were 223.77% at 0.2 μM, 176.73% at 1.0 μM, 103.46 at 2.5 μM, 100.97% at 5.0 μM, 97.10% at 10.0 μM of HMP, and 244.40% in the α-MSH only group compared to the control group without α-MSH (100%). Judging from the above results, the suppression of melanogenesis by HMP can be attributed to its inhibition of tyrosinase. As a desirable depigmenting agent, HMP should be a potent tyrosinase inhibitor without adverse effects.
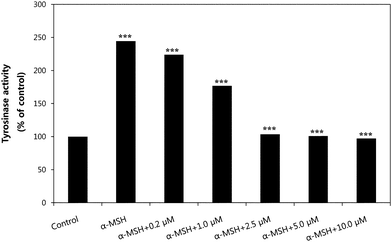 |
| Fig. 4 The effect of HMP on tyrosinase activity. In the presence of 100 nM MSH, B16 cells were treated with various doses of HMP (0.2–10.0 μM) for 24 h. Results are expressed as % of control, and each column represents the mean ± S.E. of three determinations. ***p < 0.001, compared to the group treated with 100 nM α-MSH. | |
Tyrosinase is known as a polyphenol oxidase, and has a type-3 copper center with a coupled dinuclear copper active site in its catalytic core.39,42 Recent studies found that the hydroxy groups in flavonoids inhibited the tyrosinase enzyme by participating in a metal–ligand binding interaction with the dicopper nucleus.43 Accordingly, we predicted the tertiary structure of tyrosinase and simulated its docking with HMP. Using Dock6, we searched for HMP binding residues of tyrosinase. We found that the most important expected binding residues interacting with HMP were VAL 93, PRO 96, COMPOUND LINKS
Read more about this on ChemSpider
Download mol file of compoundTHR 97, TRP 239, LEU 297, PRO 323, ASP 324, GLY 328, and LYS 329, according to Dock6 (yellow box in Fig. 5). The residues existed within 3 angstroms from the ligand. The docking simulation supported the slope–parabolic mixed-type inhibition observed, as this type of inhibition is generally observed when there are multiple binding sites for an inhibitor. The docking simulation was successful with significant scores using DOCK6 (binding energy: −31.67 kcal mol−1) and suggested that the THR 97 residue was mainly responsible for the interaction with HMP.
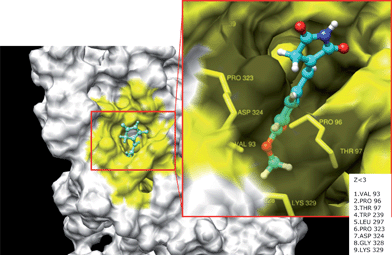 |
| Fig. 5 Computational structure prediction for tyrosinase and docking simulation with HMP. The predicted 3D structure of mushroom tyrosinase. The red box indicates HMP binding sites with tyrosinase residues. The yellow area is interacting with ligand (Z < 3). | |
Materials and methods
General
Melting points are uncorrected. 1H and 13C NMR spectra were recorded on Varian Unity INOVA 400 and Varian Unity AS 500 instruments. Chemical shifts are reported with reference to the respective residual solvent or deuterated peaks (δH 3.30 and δC 49.0 for CD3OD, δH 7.27 and δC 77.0 for COMPOUND LINKS
Read more about this on ChemSpider
Download mol file of compoundCDCl3). Coupling constants are reported in hertz. The abbreviations used are as follows: s (singlet), d (doublet), t (triplet), q (quartet), dd (doublet of doublets), and brs (broad singlet). All the reactions described below were performed under argon or nitrogen atmosphere and monitored by TLC. All anhydrous solvents were distilled over CaH2 or Na/COMPOUND LINKS
Read more about this on ChemSpider
Download mol file of compoundbenzophenone prior to use.
Procedure for the synthesis of triphenylphosphoranylidene succinimide (1)
A solution of COMPOUND LINKS
Read more about this on ChemSpider
Download mol file of compoundmaleimide (6 g, 61.81 mmol) and COMPOUND LINKS
Read more about this on ChemSpider
Download mol file of compoundtriphenylphosphine (16.2 g, 61.76 mmol) in anhydrous COMPOUND LINKS
Read more about this on ChemSpider
Download mol file of compoundacetone (60 mL) was refluxed for 1 h. After cooling, the precipitates formed were filtered through a Buchner funnel and the filter cake was washed with cold COMPOUND LINKS
Read more about this on ChemSpider
Download mol file of compoundacetone (20 mL). Drying under reduced vacuum afforded the title product (20.428 g, 92%) as a white solid. The solid was used for the next step without further purification. Melting point, 257.5–260.3 °C; 1H NMR (400 MHz, COMPOUND LINKS
Read more about this on ChemSpider
Download mol file of compoundDMSO-d6) δ 9.69 (s, 1 H, NH), 7.67–7.49 (m, 15 H, 3 × Ph), 2.85 (s, 2 H, CH2); 13C NMR (100 MHz, COMPOUND LINKS
Read more about this on ChemSpider
Download mol file of compoundDMSO-d6) δ 178.4, 172.4, 133.8 (d, J = 10.7 Hz), 133.3, 129.9 (d, J = 12.1 Hz), 126.5 (d, J = 133. 7 Hz), 38.5, 35.6 (d, J = 130.5 Hz).
A suspension of substituted benzaldehydes (0.72–2.48 mmol) and triphenylphosphoranylidene succinimide (0.72–2.48 mmol, 1.0 eq.) in COMPOUND LINKS
Read more about this on ChemSpider
Download mol file of compoundMeOH (5–10 mL) was refluxed. Before the temperature reached the boiling point of COMPOUND LINKS
Read more about this on ChemSpider
Download mol file of compoundmethanol, the reaction mixture generally became a clear solution. During reflux, the precipitates were formed and filtered through a Buchner funnel after cooling. The filter cake was washed with COMPOUND LINKS
Read more about this on ChemSpider
Download mol file of compoundmethanol and proper solvents, depending on the property of the substituted benzaldehydes used for the reaction, to give the title product (yields: 54–97.7%).
(E)-3-(4-Hydroxybenzylidene)pyrrolidine-2,5-dione (3a)
White solid; reaction time, 3 h; yield, 54%; melting point, >300 °C; 1H NMR (500 MHz, COMPOUND LINKS
Read more about this on ChemSpider
Download mol file of compoundDMSO-d6) δ 11.29 (s, 1 H, NH), 10.04 (s, 1 H, OH), 7.45 (d, 2 H, J = 9.0 Hz, 2′-H, 6′-H), 7.28 (t, 1 H, J = 2.5 Hz, vinyl H), 6.84 (d, 2 H, J = 8.5 Hz, 3′-H, 5′-H), 3.56 (d, 2 H, J = 2.5 Hz, CH2); 13C NMR (100 MHz, COMPOUND LINKS
Read more about this on ChemSpider
Download mol file of compoundDMSO-d6) δ 176.6 (C5), 172.9 (C2), 159.8 (C4′), 132.9 (C2′, C6′), 132.5 (benzyl C), 125.9, 123.5, 116.6 (C3′, C5′), 35.4 (C4); LRMS(ES) m/z 202 (M–H)−.
(E)-3-(2-Hydroxybenzylidene)pyrrolidine-2,5-dione (3b)
Pale yellowish solid; reaction time, 5 h; yield, 69.6%; melting point, 271.3–272.1 °C; 1H NMR (500 MHz, COMPOUND LINKS
Read more about this on ChemSpider
Download mol file of compoundDMSO-d6) δ 11.36 (s, 1 H, NH), 10.18 (s, 1 H, OH), 7.72 (s, 1 H, vinyl H), 7.46 (d, 1 H, J = 7.5 Hz, 6′-H), 7.24 (t, 1 H, J = 7.5 Hz, 4′-H), 6.92 (d, 1 H, J = 8.0 Hz, 3′-H), 6.87 (t, 1 H, J = 7.5 Hz, 5′-H), 3.59 (d, 2 H, J = 2.0 Hz, CH2); 13C NMR (100 MHz, COMPOUND LINKS
Read more about this on ChemSpider
Download mol file of compoundDMSO-d6) δ 176.6 (C5), 172.9 (C2), 157.7 (C2′), 131.9 (benzyl C), 129.7 (C6′), 126.9 (C4′), 125.8 (C3), 121.7 (C1′), 120.0 (C5′), 116.5 (C3′), 35.4 (C4); LRMS(ES) m/z 202 (M–H)−.
(E)-3-(4-Methoxybenzylidene)pyrrolidine-2,5-dione (3c)
White solid; reaction time, 5 h; yield, 85%; melting point, 243.4–245.7 °C; 1H NMR (500 MHz, COMPOUND LINKS
Read more about this on ChemSpider
Download mol file of compoundDMSO-d6) δ 11.33 (s, 1 H, NH), 7.56 (d, 2 H, J = 7.0 Hz, 2′-H, 6′-H), 7.32 (s, 1 H, vinyl H), 7.01 (d, 2 H, J = 6.5 Hz, 3′-H, 5′-H), 3.79 (s, 3 H, OCH3), 3.58 (s, 2 H, CH2); 13C NMR (100 MHz, COMPOUND LINKS
Read more about this on ChemSpider
Download mol file of compoundDMSO-d6) δ 176.4 (C5), 172.7 (C2), 161.1 (C4′), 132.7 (C2′, C6′), 132.1 (benzyl C), 127.5, 124.7, 115.2 (C3′, C5′), 56.0 (OCH3), 35.4 (C4); LRMS(ES) m/z 216 (M–H)−.
(E)-3-(3,4-Dihydroxybenzylidene)pyrrolidine-2,5-dione (3d)
Pale brownish solid; reaction time, 42 h; yield, 58.6%; melting point, 290.8–291.9 °C; 1H NMR (500 MHz, COMPOUND LINKS
Read more about this on ChemSpider
Download mol file of compoundDMSO-d6) δ 11.30 (br s, 1 H, NH), 9.47 (br s, 2 H, 2 × OH), 7.18 (s, 1 H, vinyl H), 6.99 (s, 1 H, 2′-H), 6.92 (d, 1 H, J = 7.5 Hz, 6′-H), 6.80 (d, 1 H, J = 7.5 Hz, 5′-H), 3.59 (d, 2 H, J = 2.0 Hz, CH2); 13C NMR (100 MHz, COMPOUND LINKS
Read more about this on ChemSpider
Download mol file of compoundDMSO-d6) δ 176.6 (C5), 172.9 (C2), 148.4 (C4′), 146.2 (C3′), 132.9 (benzyl C), 126.3 (C3), 124.0 (C6′), 123.2(C1′), 117.6, 116.6, 35.5 (C4); LRMS(ES) m/z 218 (M–H)−.
(E)-3-(3,5-Dihydroxybenzylidene)pyrrolidine-2,5-dione (3e)
White solid; reaction time, 1.5 h; yield, 79.3%; melting point, 275.2–277.8 °C; 1H NMR (500 MHz, COMPOUND LINKS
Read more about this on ChemSpider
Download mol file of compoundDMSO-d6) δ 11.37 (s, 1 H, NH), 9.44 (s, 2 H, 2 × OH), 7.13 (t, 1 H, J = 2.0 Hz, vinyl H), 6.43 (d, 2 H, J = 2.0 Hz, 2′-H), 6.27 (t, 1 H, J = 2.0 Hz, 4′-H), 3.52 (d, 2 H, J = 2.0 Hz, CH2); 13C NMR (100 MHz, COMPOUND LINKS
Read more about this on ChemSpider
Download mol file of compoundDMSO-d6) δ 176.3 (C5), 172.7 (C2), 159.3 (C3′, C5′), 136.3 (C1′), 132.6 (benzyl C), 127.1 (C3), 108.9 (C2′, C6′), 104.9 (C4′), 35.6 (C4); LRMS(ES) m/z 218 (M–H)−.
(E)-3-(3-Hydroxy-4-methoxybenzylidene)pyrrolidine-2,5-dione (3f)
White solid; reaction time, 10 h; yield, 85.3%; melting point, 268.7–270.3 °C; 1H NMR (500 MHz, COMPOUND LINKS
Read more about this on ChemSpider
Download mol file of compoundDMSO-d6) δ 11.31 (s, 1 H, NH), 9.24 (s, 1 H, OH), 7.22 (s, 1 H, vinyl H), 7.04 (d, 1 H, J = 9.0 Hz, 6′-H), 7.03 (s, 1 H, 2′-H), 6.98 (d, 1 H, J = 8.5 Hz, 5′-H), 3.80 (s, 3 H, CH3), 3.53 (s, 2 H, CH2); 13C NMR (100 MHz, COMPOUND LINKS
Read more about this on ChemSpider
Download mol file of compoundDMSO-d6) δ 176.4 (C5), 172.8 (C2), 150.0 (C4′), 147.3 (C3′), 132.5 (benzyl C), 127.7 (C3), 124.5 (C1′), 123.7 (C6′), 117.1 (C2′), 112.9 (C5′), 56.3 (CH3), 35.5 (C4); LRMS(ES) m/z 232 (M–H)−.
(E)-3-(4-Hydroxy-3-methoxybenzylidene)pyrrolidine-2,5-dione (3g)
White solid; reaction time, 14 h; yield, 68.5%; melting point, 243.5–245.7 °C; 1H NMR (400 MHz, COMPOUND LINKS
Read more about this on ChemSpider
Download mol file of compoundDMSO-d6) δ 11.28 (s, 1 H, NH), 9.65 (s, 1 H, OH), 7.25 (s, 1 H, vinyl H), 7.10 (d, 1 H, J = 2.0 Hz, 2′-H), 7.03 (dd, 1 H, J = 2.0, 8.4 Hz, 6′-H), 6.81 (d, 1 H, J = 8.4 Hz, 5′-H), 3.79 (s, 3 H, CH3), 3.59 (d, 2 H, J = 2.0 Hz, CH2); 13C NMR (100 MHz, COMPOUND LINKS
Read more about this on ChemSpider
Download mol file of compoundDMSO-d6) δ 176.6 (C5), 172.9 (C2), 149.3, 148.4, 132.9 (benzyl C), 126.3 (C3), 125.1 (C6′), 123.6 (C1′), 116.5, 114.4, 56.2 (CH3), 35.3 (C4); LRMS(ES) m/z 232 (M–H)−.
(E)-3-(3-Ethoxy-4-hydroxybenzylidene)pyrrolidine-2,5-dione (3h)
White solid; reaction time, 4 h; yield, 58%; melting point, 222.3–224.1 °C; 1H NMR (500 MHz, COMPOUND LINKS
Read more about this on ChemSpider
Download mol file of compoundDMSO-d6) δ 11.29 (s, 1 H, NH), 9.57 (s, 1 H, OH), 7.27 (s, 1 H, vinyl H), 7.11 (s, 1 H, 2′-H), 7.05 (d, 1 H, J = 8.0 Hz, 6′-H), 6.85 (d, 1 H, J = 8.5 Hz, 5′-H), 4.07 (q, 2 H, J = 7.0 Hz, CH3CH2-), 3.60 (s, 2 H, 4-CH2), 1.34 (t, 3 H, J = 7.0 Hz, CH3CH2-); 13C NMR (100 MHz, COMPOUND LINKS
Read more about this on ChemSpider
Download mol file of compoundDMSO-d6) δ 176.5 (C5), 172.8 (C2), 149.6 (C4′), 147.6 (C3′), 132.9 (benzyl C), 126.4 (C3), 125.1 (C6′), 123.6 (C1′), 116.6, 115.9, 64.6 (OCH2), 35.3 (C4), 15.3 (CH2CH3); LRMS(ES) m/z 246 (M–H)−.
(E)-3-(2,4-Dimethoxybenzylidene)pyrrolidine-2,5-dione (3i)
Pale yellowish solid; reaction time, 8 h; yield, 62%; melting point, 245.7–246.6 °C; 1H NMR (500 MHz, COMPOUND LINKS
Read more about this on ChemSpider
Download mol file of compoundDMSO-d6) δ 11.29 (s, 1 H, NH), 7.62 (s, 1 H, vinyl H), 7.46 (d, 1 H, J = 8.5 Hz, 6′-H), 6.63 (d, 1 H, J = 2.0 Hz, 3′-H), 6.60 (dd, 1 H, J = 2.5, 8.5 Hz, 5′-H), 3.85 (s, 3 H, CH3), 3.81 (s, 3 H, CH3), 3.54 (d, 2 H, J = 2.0 Hz, CH2); 13C NMR (100 MHz, COMPOUND LINKS
Read more about this on ChemSpider
Download mol file of compoundDMSO-d6) δ 176.6 (C5), 173.0 (C2), 162.9 (C4′), 160.3 (C2′), 131.0 (benzyl C), 126.2 (C6′), 124.0 (C3), 116.1 (C1′), 106.7 (C5′), 99.0 (C3′), 56.5 (OCH3), 56.2 (OCH3), 35.4 (C4); LRMS(ES) m/z 246 (M–H)−.
(E)-3-(3,4-Dimethoxybenzylidene)pyrrolidine-2,5-dione (3j)
White solid; reaction time, 5 h; yield, 79.6%; melting point, 237.1–238.4 °C; 1H NMR (400 MHz, COMPOUND LINKS
Read more about this on ChemSpider
Download mol file of compoundDMSO-d6) δ 11.31 (s, 1 H, NH), 7.29 (s, 1 H, vinyl H), 7.14 (d, 1 H, J = 8.4 Hz, 6′-H), 7.12 (s, 1 H, 2′-H), 6.99 (d, 1 H, J = 8.4 Hz, 5′-H), 3.78 (s, 3 H, CH3), 3.76 (s, 3 H, CH3), 3.61 (s, 2 H, CH2); 13C NMR (100 MHz, COMPOUND LINKS
Read more about this on ChemSpider
Download mol file of compoundDMSO-d6) δ 176.5 (C5), 172.7 (C2), 151.0, 149.5, 132.5 (benzyl C), 127.6 (C1′), 124.7 (C3, C6′), 113.8, 112.5, 56.3 (OCH3), 56.2 (OCH3), 35.3 (C4); LRMS(ES) m/z 246 (M–H)−.
(E)-3-(4-Hydroxy-3,5-dimethoxybenzylidene)pyrrolidine-2,5-dione (3k)
Pale yellowish solid; reaction time, 2.5 h; yield, 97.7%; melting point, 236.7–237.7 °C; 1H NMR (400 MHz, COMPOUND LINKS
Read more about this on ChemSpider
Download mol file of compoundDMSO-d6) δ 11.29 (s, 1 H, NH), 9.01 (s, 1 H, OH), 7.27 (s, 1 H, vinyl H), 6.83 (s, 2 H, 2′-H, 6′-H), 3.78 (s, 6 H, 3′-OCH3, 5′-OCH3), 3.64 (s, 2 H, CH2); 13C NMR (100 MHz, COMPOUND LINKS
Read more about this on ChemSpider
Download mol file of compoundDMSO-d6) δ 176.6 (C5), 172.8 (C2), 148.7 (C3′, C5′), 138.5 (C4′), 133.2 (benzyl C), 125.1 (C3), 123.9 (C1′), 108.7 (C2′, C6′), 56.7 (3′-OCH3, 5′-OCH3), 35.2 (C4); LRMS(ES) m/z 262 (M–H)−.
(E)-3-(3,4,5-Trimethoxybenzylidene)pyrrolidine-2,5-dione (3l)
White solid; reaction time, 3 h; yield, 66%; melting point, 191.2–193.6 °C; 1H NMR (500 MHz, COMPOUND LINKS
Read more about this on ChemSpider
Download mol file of compoundDMSO-d6) δ 11.39 (s, 1 H, NH), 7.33 (s, 1 H, vinyl H), 6.90 (s, 2 H, 2′-H, 6′-H), 3.82 (s, 6 H, 3′-OCH3, 5′-OCH3), 3.71 (s, 2 H, CH2), 3.69 (s, 3 H, 4′-OCH3); 13C NMR (100 MHz, COMPOUND LINKS
Read more about this on ChemSpider
Download mol file of compoundDMSO-d6) δ 176.5 (C5), 172.6 (C2), 153.6 (C3′, C5′), 139.5 (C4′), 132.5 (benzyl C), 130.3 (C1′), 126.5 (C3), 108.3 (C2′, C6′), 60.8 (4′-OCH3), 56.6 (3′-OCH3, 5′-OCH3), 35.1 (C4); LRMS(ES) m/z 276 (M–H)−.
Materials
Mushroom tyrosinase, L-tyrosine [3-(4-Hydroxyphenyl)]-L-alanine, (S)-2-Amino-3-(4-hydroxyphenyl) COMPOUND LINKS
Read more about this on ChemSpider
Download mol file of compoundpropionic acid), COMPOUND LINKS
Read more about this on ChemSpider
Download mol file of compoundkojic acid [COMPOUND LINKS
Read more about this on ChemSpider
Download mol file of compound5-hydroxy-2-(hydroxymethyl)-4H-pyran-4-one] and α-MSH (Alpha-Melanocyte Stimulating Hormone) were purchased from Sigma Chemical Co (St. Louis, MO, U.S.A). Solvents used for organic syntheses were redistilled. All other chemicals and solvents were of analytical grade and used without further purification.
Cell culture
B16
cells (obtained from the Korean Cell Line Bank) were cultured in DMEM with 10% fetal bovine serum (FBS; Gibco) and penicillin/COMPOUND LINKS
Read more about this on ChemSpider
Download mol file of compoundstreptomycin (100 IU/5 μg ml−1) in a humidified atmosphere containing 5% CO2 in air at 37 °C. B16 cells were cultured in 24-well plates for melanin quantification and enzyme activity assays.
Cell viability
Cell survival was quantified by a colorimetric MTT assay that measures mitochondrial activity in viable cells. This method is based on the conversion of 3-(4,5-dimethylthiazol-2-yl)-2,5-diphenyltetrazolium bromide (MTT, Sigma) to MTT-formazan crystal by mitochondrial enzymes as previously described.44 Briefly, cells seeded at a density of 3 × 104/cell in a Corning 48-well plate (Corning, NY, USA), were allowed to adhere overnight; the culture medium was then replaced with fresh serum free DMEM. MTT was freshly prepared at 5 mg ml−1 in phosphate-buffered saline (PBS). Aliquots of 500 μl of MTT stock solution were added to each well, and the plate was incubated at 37 °C for 4 h in a humidified 5% CO2 incubator. After 4 h, the medium was removed. To each well, 500 μl of COMPOUND LINKS
Read more about this on ChemSpider
Download mol file of compoundEtOH–COMPOUND LINKS
Read more about this on ChemSpider
Download mol file of compoundDMSO (1
:
1 mixture solution) was added to dissolve COMPOUND LINKS
Read more about this on ChemSpider
Download mol file of compoundformazan. After 10 min, the optical density of each well was measured spectrophotometrically with a 560 nm filter. Results from three experiments are shown.
Assay to measure inhibitory effects of compounds on mushroom tyrosinase activity
Mushroom-derived tyrosinase was used as the source of the enzyme for the entire study. Tyrosinase activity was determined as described previously with minor modification.36 Briefly, 20 μl of an aqueous solution of mushroom tyrosinase (1000 units) was added to a 96-well microplate (Nunc, Denmark), in a total assay mixture volume of 200 μl containing 1 mM L-tyrosine solution, and 50 mM phosphate buffer (pH 6.5). The assay mixture was incubated at 25 °C for 30 min. Following incubation, the amount of dopachrome produced in the reaction mixture was determined spectrophotometrically at 492 nm (OD492) using a microplate reader (Hewlett Packard). IC50, inhibitory concentration-50, is the concentration of a drug that inhibits a standard 50% response. IC50 is a value derived from the X-axis and is determined from the alignment of the dose–response curve on the dependent Y-axis. In the present study, dose-dependent inhibition experiments were performed in triplicate to determine the IC50 of a drug. According to the inhibition percentage of three doses in each experiment, log-linear curves and their equations were determined. Then, the individual IC50 was calculated as the concentration at which the Y-axis value equaled 50% inhibition. Results are shown from three experiments.
Kinetic analysis of tyrosinase inhibition
Various concentrations of COMPOUND LINKS
Read more about this on ChemSpider
Download mol file of compoundL-tyrosine (0.25–2 mM) as substrate, 20 μl of aqueous solution of mushroom tyrosinase (1000 units), and 50 mM COMPOUND LINKS
Read more about this on ChemSpider
Download mol file of compoundpotassium phosphate buffer (pH 6.5) with or without test samples (1.25 and 20.0 μM of HMP) were added to a 96-well plate in a total volume assay mixture of 200 μl. Using a microplate reader, the initial rate of dopachrome formation from the reaction mixture was determined by the increase in absorbance at 492 nm per min (ΔOD492/min). The Michaelis constant (Km) and the maximal velocity (Vmax) of the tyrosinase activity were determined by Lineweaver–Burk plots at various concentrations of COMPOUND LINKS
Read more about this on ChemSpider
Download mol file of compoundL-tyrosine as a substrate.36 The reaction kinetics required a modification of the Michaelis–Menten equation due to competitive inhibition by HMP together with substrate inhibition by COMPOUND LINKS
Read more about this on ChemSpider
Download mol file of compoundL-tyrosine. The result from three experiments is shown.
Assay of murine tyrosinase activity
Murine tyrosinase activity was estimated by measuring the rate of oxidation of L-DOPA.45Cells were plated in 24-well dishes at a density of 5 × 104cells ml−1. B16 cells were incubated in the presence or absence of 100 nM α-MSH and then treated for 24 h at various concentrations of HMP (0–10 μM). Cells were washed and lysed in 100 μl of 50 mM COMPOUND LINKS
Read more about this on ChemSpider
Download mol file of compoundsodium phosphate buffer (pH 6.5) containing 1% Triton X-100 (sigma) and 0.1 mM PMSF (COMPOUND LINKS
Read more about this on ChemSpider
Download mol file of compoundphenylmethylsulfonyl fluoride) and then frozen at −80 °C for 30 min. After thawing and mixing, cellular extracts were clarified by centrifugation at 12
000 rpm for 30 min at 4 °C. Eight μl of the supernatant and 20 μl of L-DOPA (2 mg ml−1) were put in a 96-well plate, and the absorbance at 492 nm was read every 10 min for 1 h at 37 °C using an ELISA plate reader. The final activity was expressed as ΔOD/min for each condition. Results from three experiments are shown.
Determination of melanogenesis in B16 cells
Determination of melanin content was done using a modification of the method of Bilodeau et al.46 The amount of melanin was used as an index of melanogenesis. B16 cells (5 × 104) were plated on 24-well dishes and incubated in the presence or absence of 100 nM α-MSH. Cells were then incubated for 24 h with various concentrations of HMP (0–10 μM). After washing twice with PBS, samples were dissolved in 100 μl of 1 N COMPOUND LINKS
Read more about this on ChemSpider
Download mol file of compoundNaOH. The samples were incubated at 60 °C for 1 h and mixed to solubilize the melanin. The absorbance at 405 nm was compared with a standard curve of synthetic melanin. Results from three experiments are shown.
Homology modeling of tyrosinase
A three-dimensional model of Lentinula edodes (mushroom) tyrosinase containing 618 amino acids was built using SWISS-MODEL47 based on homology modeling.48 The SWISS-MODEL program automatically provides an all-atom model using alignments between the query sequence and known homologous structures. For homology modeling, the known homologous structures of tyrosinase from the Protein Data Bank (PDB) (http://www.pdb.org) were used as the structural templates.
In silico docking of tyrosinase and novel inhibitor candidates
Among the many tools available for in silicoprotein-ligand docking, DOCK6 is the one most commonly used, because of the automated docking capability. The program performs ligand docking using a set of predefined 3D grids of the target protein using a systemic search technique.49
We describe the structures of novel inhibitor candidates in Fig. 5. To prepare for the docking procedure, the following steps were taken: 1) conversion of 2D structures to 3D structures, 2) calculation of charges, and 3) addition of hydrogen atoms using the ChemOffice program (http://www.cambridgesoft.com).
Statistical analysis
The inhibition of tyrosinase activity is expressed as a percentage of inhibition based on: 100 − [(A × 100)/B], where A = OD492 with a test sample and B = OD492 without a test sample. Data collected are presented as means ± standard errors (n = 3). The statistical significance of differences between groups was determined by one-factor analysis of variance (ANOVA) followed by Fischer's protected least significant difference post hoc test. Values of *p < 0.05 were considered statistically significant.
Conclusions
In this study, we describe the synthesis and tyrosinase inhibitory activity of a new family of hydroxybenzylidenyl pyrrolidine-2,5-dione compounds. We investigated in depth the bioactivities of 3f (HMP) because it exhibited the most potent inhibitory activity against mushroom tyrosinase among the compounds we synthesized. High affinity of the 3-position hydroxy and 4-position methoxy groups of HMP is most likely due to hydrogen-bonding interactions with one of the active site residues (PRO 96). Changing the number and position of the methoxy and hydroxyl groups of HMP decreased the inhibitory activity. Our results indicate that the number and position of methoxy and hydroxy groups on the phenyl ring are closely associated with tyrosinase inhibition activity. We found from docking simulation that HMP strongly binds to mushroom tyrosinase. In a kinetic study, HMP was identified as a competitive inhibitor of mushroom tyrosinase, with the same Vmax value regardless of the concentration of HMP. Therefore, the enzyme inhibitory activity of HMP might result from binding at the same site as the copper active site of mushroom tyrosinase. In the B16 cell culture system, tyrosinase activity and melanin levels were also significantly reduced by the addition of HMP to the incubation medium containing cells pretreated with α-MSH. HMP exhibited more potent anti-tyrosinase activity than did COMPOUND LINKS
Read more about this on ChemSpider
Download mol file of compoundkojic acid, a well-known tyrosinase inhibitor. All these findings suggest that HMP might be utilized for the development of new candidates for the treatment of dermatological disorders related to pigmentation.
Acknowledgements
This work was supported by the National Research Foundation of Korea (NRF) grant funded by the Korea government (MEST) (No. 2009-0083538) and Basic Science Research Program through the National Research Foundation of Korea (NRF) funded by the Ministry of Education, Science and Technology (No. 2010-0012038). We thank the Aging Tissue Bank for providing research materials for the study.
Notes and references
- L. Vomos-Vigyazo, Crit. Rev. Food Sci. Nutr., 1981, 15, 49–127 CrossRef.
- K. G. Strothkemp, R. L. Jolley and H. S. Mason, Biochem. Biophys. Res. Commun., 1976, 70, 519–524 CrossRef.
- R. Halaban, E. Cheng, S. Svedine, R. Aron and D. N. Hebert, J. Biol. Chem., 2001, 276, 11933–11938 CrossRef CAS.
- R. Halaban, R. S. Patton, E. Cheng, S. Svedine, E. S. Trombetta, M. L. Wahl, S. Ariyan and D. N. Hebert, J. Biol. Chem., 2002, 277, 14821–14828 CrossRef CAS.
- H. S. Raper, Physiol. Rev., 1928, 8, 245–282 CAS.
- H. S. Mason, J. Biol. Chem., 1948, 172, 83–99 CAS.
- A. B. Lerner, T. B. Fitzpatrick, E. Calkins and W. H. Summerson, J. Biol. Chem., 1949, 178, 185–190 CAS.
- K. Iozumi, G. E. Hoganson, R. Pennella, M. A. Everett and B. B. Fuller, J. Invest. Dermatol., 1993, 100, 806–811 CrossRef CAS.
-
T. B. Fitzpatrick, A. Z. Eisen, K. Woff, I. M. Freedberg and K. F. Austen, Dermatology in General Medicine, McGraw-Hill, New York, 1979 Search PubMed.
- Y. Xu, A. H. Stokes, W. M. Freeman, S. C. Kumer, B. A. Vogt and K. E. Vrana, Mol. Brain Res., 1997, 45, 159–162 CrossRef CAS.
- S. J. Cho, J. S. Roh, W. S. Sun, S. H. Kim and K. D. Park, Bioorg. Med. Chem. Lett., 2006, 16, 2682–2684 CrossRef CAS.
- M. Funayama, H. Arakawa, R. Yamamoto and T. Nishino, Biosci., Biotechnol., Biochem., 1995, 59, 143–144 CrossRef CAS.
- K. Sugimoto, T. Nishmura, K. Nomura, K. Sugimoto and T. Kuriki, Chem. Pharm. Bull., 2003, 51, 798–801 CrossRef CAS.
- W. Yi, R. H. Cao, H. Wen, Q. Yan and B. H. Zhou, Bioorg. Med. Chem. Lett., 2008, 18, 6490–6493 CrossRef CAS.
- J. B. Liu, R. H. Cao, W. Yi, C. M. Ma, Y. Q. Wan, B. H. Zhou, L. Ma and H. C. Song, Eur. J. Med. Chem., 2009, 44, 1773–1778 CrossRef CAS.
- J. B. Liu, W. Yi, Y. Q. Wan, L. Ma and H. C. Song, Bioorg. Med. Chem., 2008, 16, 1096–1102 CrossRef CAS.
- S. Sabrina Okombi, D. Rival, S. Bonnet, A. M. Mariotte and A. Perrier Boumendjel, Bioorg. Med. Chem. Lett., 2006, 16, 2252–2255 CrossRef.
- S. Okombi, D. Rival, S. Bonnet, A. M. Mariotte, E. Perrier and A. Boumendjel, J. Med. Chem., 2006, 49, 329–333 CrossRef CAS.
- A. Andrawis and V. Kahn, Biochem. J., 1986, 235, 91–96 CAS.
- T. S. Chang, Int. J. Mol. Sci., 2009, 10, 2440–2475 Search PubMed.
- S. Parvez, M. Kang, H. S. Chung and H. Bae, Phytother. Res., 2007, 21, 805–816 CrossRef CAS.
- Y. M. Ha, S. W. Chung, S. Song, H. Lee, H. Suh and H. Y. Chung, Biol. Pharm. Bull., 2007, 30, 1711–1715 CrossRef CAS.
- H. Takiwaki, S. Shirai, H. Kohno, H. Soh and S. Arase, J. Invest. Dermatol., 1994, 103, 642–646 CrossRef CAS.
- N. H. Shin, S. Y. Ryu, H. S. Lee, K. R. Min and Y. S. Kim, Planta Med., 1998, 64, 283–284 CrossRef CAS.
- S. Nimmanpisut, P. Chudapongse and K. Ratanabanangkoon, Biochem. Pharmacol., 1976, 25, 1245–1248 CrossRef CAS.
- S. H. Song, H. J. Lee, Y. G. Jin, Y. M. Ha, S. J. Bae, H. Y. Chung and H. S. Suh, Bioorg. Med. Chem. Lett., 2007, 17, 461–464 CrossRef CAS.
- N. H. Shin, S. Y. Ryu, E. J. Choi, S. H. Kang, I. M. Chang, K. R. Min and Y. S. Kin, Biochem. Biophys. Res. Commun., 1998, 243, 801–803 CrossRef CAS.
- S. Y. Choi, S. H. Kim, H. C. Kim, K. H. Suk, J. S. Hwang, M. G. Lee, A. J. Kin and S. Y. Kim, Chem. Pharm. Bull., 2002, 50, 450–452 CrossRef CAS.
- S. K. Ha, K. Mamoro, K. Lee, S. Y. Choi, J. H. Park, I. Hideharu and S. Y. Kim, Biol. Pharm. Bull., 2005, 28, 838–840 CrossRef CAS.
- K. Ohguchi, T. Tanaka, I. Iliya, T. Ito, M. Iinuma, K. Matsumoto, Y. Akao and Y. Nozawa, Biosci., Biotechnol., Biochem., 2003, 67, 663–665 CrossRef CAS.
- K. Ohguchi, T. Tanaka, I. Iliya, T. Ito, M. Iinuma, K. Matsumoto, Y. Akao and Y. Nozawa, Biosci., Biotechnol., Biochem., 2003, 67, 1587–1589 CrossRef CAS.
- Y. J. Kim, J. K. No, J. H. Lee and H. Y. Chung, Biol. Pharm. Bull., 2005, 28, 323–327 CrossRef CAS.
- J. K. No, M. S. Kim, Y. J. Kim, S. J. Bae, J. S. Choi and H. Y. Chung, Am. J. Chin. Med., 2004, 32, 97–103 Search PubMed.
- J. T. Lim, Dermatol. Sur., 1999, 25, 292–284 Search PubMed.
- U. Ghani and N. Ullah, Bioorg. Med. Chem., 2010, 18, 4042–4048 CrossRef CAS.
- J. K. No, D. Y. Soung, Y. J. Kim, K. H. Shim, Y. S. Jun, S. H. Rhee, T. Yokozawa and H. Y. Chung, Life Sci., 1999, 65, 241–246.
- S. Passi and M. Nazzaro-Porro, Br. J. Dermatol., 1981, 104, 659–665 CrossRef CAS.
- J. M. Menter, A. A. Etemadi, W. Chapman, T. D. Hollins and I. Willis, Melanoma Res., 1993, 6, 443–449 CrossRef.
- V. J. Hearing and K. Tsukamoto, FASEB J., 1991, 5, 2902–2909 CAS.
- V. Kahn and A. Andrawis, Phytochemistry, 1985, 24, 905–908 CrossRef CAS.
- G. Battaini, E. Monzani, L. Casella, L. Santagostini and R. Pagliarin, JBIC, J. Biol. Inorg. Chem., 2000, 5, 262–268 CrossRef CAS.
- Y. Matoba, T. Kumagai, A. Yamamoto, H. Yoshitsu and M. Sugiyama, J. Biol. Chem., 2006, 281, 8981–8990 CrossRef CAS.
- D. Kim, J. Park, J. Kim, C. Han, J. Yoon, N. Kim, J. Seo and C. Lee, J. Agric. Food Chem., 2006, 54, 935–941 CrossRef CAS.
- H. Tada, O. Shiho, K. Kuroshima, M. Koyama and K. Tsukamoto, J. Immunol. Methods, 1986, 93, 157–165 CrossRef CAS.
- D. S. Kim, S. Y. Kim, J. H. Chung, K. H. Kim, H. C. Eun and K. C. Park, Cell. Signalling, 2002, 14, 779–785 CrossRef CAS.
- M. L. Bilodeau, J. D. Greulich, R. L. Hullinger, C. Bertolotto, R. Ballotti and O. M. Andrisani, Pigm. Cell Res., 2001, 14, 328–336 Search PubMed.
- K. Arnold, L. Bordoli, J. Kopp and T. Schwede, Bioinformatics, 2006, 22, 195–201 CAS.
- R. Rodriguez, G. Chinea, N. Lopez, T. Pons and G. Vriend, Bioinformatics, 1998, 14, 523–528 CrossRef CAS.
- D. T. Moustakas, P. T. Lang, S. Pegg, E. Pettersen, I. D. Kuntz, N. Brooijmans and R. C. Rizzo, J. Comput.-Aided Mol. Des., 2006, 20, 601–619 CrossRef CAS.
|
This journal is © The Royal Society of Chemistry 2011 |