Speciation and identification of low molecular weight selenium compounds in the liver of sea turtles†
Received
25th May 2010
, Accepted 26th August 2010
First published on 24th September 2010
Abstract
It is known that marine mammals and seabirds co-accumulate selenium (Se) and mercury (Hg) in their organs in the insoluble form called mercury selenide. In this study, we found that two sea turtles, hawksbill turtle (Eretmochelys imbricata) and green turtle (Chelonia mydas), accumulated Se but not Hg in their livers. Se speciation by HPLC-ICP-MS demonstrated that the livers contained low molecular weight selenometabolites in addition to selenoproteins. Two of the selenometabolites existed in relatively small amounts and were identified as selenosugar (1β-methylseleno-N-acetyl-D-galactosamine) and trimethylselenonium (TMSe) based on their chromatographic behavior. This suggests that selenosugar and TMSe are Se metabolites common to marine and terrestrial animals. The chromatographic behavior of the major hepatic selenometabolite in sea turtles was unique and did not match that of any authentic Se standards. Further analysis using HPLC-ESI-MS-MS revealed it to be selenoneine (2-selenyl-N,N,N-trimethyl-L-histidine), a metabolite that was recently identified in the blood of bluefin tuna. The results suggest that sea turtles possess specific mechanisms for Se metabolism to result in the sole accumulation of Se.
Introduction
Selenium (Se) is an essential micronutrient in animals because it promotes the activities of such selenoenzymes as glutathione peroxidases, iodothyronine 5′-deiodinase, and thioredoxin reductase, by existing as selenocysteine (SeCys) in their active centers.1,2 The metabolic pathway of Se from its ingestion to excretion has been well studied in experimental animals.3 It is known that all Se species ingested via the food chain and drinking water are transformed into selenide, and this is utilized for the biosyntheses of SeCys for incorporation into selenoproteins, or selenosugars and trimethylselenonium (TMSe) for excretion. One inorganic Se, selenite, is simply reduced to selenide by endogenous reductants, such as glutathione (GSH).4 Although the other inorganic Se, selenate, is not reduced to selenide by GSH in vitro, it is also utilized in selenoprotein synthesis and excreted as selenosugar and TMSe in vivo. On the other hand, organic selenocompounds ingested via the food chain, which are mostly selenoamino acids (e.g., SeCys, Se-methylselenocysteine (MeSeCys), and selenomethionine (SeMet)), are transformed into selenide via several pathways. Consequently, even though all Se species are ingested via the food chain and drinking water, Se is utilized to form selenoproteins and excreted as 1β-methylseleno-N-acetyl-D-galactosamine (the major urinary selenosugar) in animals.
Another biological significance of Se in addition to the formation of the active center of selenoenzymes is that Se detoxifies inorganic mercury (Hg) by directly interacting with it in experimental animals.5–7 Besides inorganic Hg, a similar antagonism was reported between Se and methylmercury in animals.8 It is also known that higher trophic marine animals, such as marine mammals and seabirds, co-accumulate Se and Hg in their tissues at a 1
:
1 molar ratio.9,10 However, contrary to the co-accumulation of Se and Hg in marine mammals and seabirds, we previously found that some sea turtles accumulated Se but not Hg in their livers.11 The sole accumulation of Se in the livers suggests that some marine animals other than marine mammals and seabirds have a unique pathway for Se metabolism. However, at present, limited information is available on the Se metabolism in marine animals except Hg detoxification. As Se forms organoselenium compounds as its metabolites, the identification of unknown selenometabolites can pave the way to elucidating the metabolic pathway of Se.
The inductively coupled plasma mass spectrometer (ICP-MS) is a superior instrument for elemental speciation owing to its high sensitivity and specificity when used in combination with separation instruments such as a high-performance liquid chromatograph (HPLC) and a gas chromatograph. HPLC-ICP-MS is the technique of choice for Se speciation, in particular, for the screening of Se compounds in samples. The assignment of Se compounds by HPLC-ICP-MS is performed by matching the retention times of samples with those of authentic standards. However, this technique is inappropriate and insufficient for the assignment of Se compounds whose standards are not available and thus, the sole use of HPLC-ICP-MS does not allow us to unambiguously identify unknown Se compounds.12,13 Electrospray ionization mass spectrometry (ESI-MS) coupled with HPLC is another technique for elemental speciation. A tandem mass spectrometer (ESI-MS-MS) is a powerful tool to identify unknown Se compounds/metabolites in biological samples.14
In the present study, speciation analysis using an HPLC coupled with an ICP-MS and ESI-MS-MS was performed to identify unknown Se species in the livers of hawksbill turtle (Eretmochelys imbricata) and green turtle (Chelonia mydas).
Experimental
Selenocompounds
Sodium selenite, sodium selenate, and L-selenomethionine (SeMet) were purchased from Wako (Osaka, Japan). Methylselenocysteine (MeSeCys) and trimethylselenonium (TMSe) iodide were purchased from Acros Organics (Geel, Belgium) and Tri Chemical Laboratories (Yamanashi, Japan), respectively. 1β-Methylseleno-N-acetyl-D-galactosamine (selenosugar) was synthesized in our laboratory.15
Sample
Liver tissues of hawksbill turtle (E. imbricata) and green turtle (C. mydas) were provided by fishermen in the Yaeyama Islands, Okinawa, Japan. Three each of hawksbill turtles and green turtles were collected from Ishigaki Island, Okinawa, Japan in November 2000, for commercial and scientific purposes after receiving the appropriate permission from the Japanese government. The standard carapace lengths of the hawksbill turtles and the green turtles were 42.1 ± 5.0 cm and 42.1 ± 4.1 cm, respectively. Following the collection, the livers were immediately frozen in liquid nitrogen and stored at −80 °C until analyses in the Environmental Specimen Bank for Global Monitoring (es-BANK), Center for Marine Environmental Studies (CMES), Ehime University, Japan.16 Frozen liver specimens of a terrestrial turtle, the snapping turtle (Chelydra serpentina), which was exterminated because it was considered to be an invasive species in Chiba, Japan, were provided by the Japan Wildlife Research Center. These samples were stored in a freezer at −20 °C until analysis.
Determination of Se and Hg concentrations
Liver samples were dried for 12 h at 80 °C and homogenously powdered. A 0.1 g portion of powdered sample was weighted in a Teflon® tube and 1.5 mL of concentrated nitric acid (ca. 63%, analytical grade) was added. After pre-digestion at room temperature overnight, the samples were digested in a home microwave oven for 10 min at 200 W and the digestion was repeated twice. The digested samples were diluted with deionized water. Se concentrations in each sample were measured with an ICP-MS (Agilent 7500ce, Agilent Technologies, Hachioji, Japan). Important parameters for operation were as follows: plasma RF power, 1500 W; plasma gas flow, 15.0 L min−1; auxiliary gas flow, 1.15 L min−1; nebulizer gas flow, 1.05 L min−1; m/z monitored, 82; dwell time, 100 ms; and point per peak, 1. Mercury concentration was determined by cold vapor (Model HG-3000, Sanso, Tokyo, Japan)-atomic absorption spectrometry (AA-680, Shimadzu, Kyoto, Japan). A mercury hollow cathode lamp operated at 4 mA was used as radiation source for the 253.7 nm mercury line. Detection limits of Se and Hg by the procedure were 0.5 and 2 ng mL−1, respectively.
Approximately 1.0 g of tissue was homogenized with a 10-fold volume of 50 mM Tris–HCl, pH 7.6, under nitrogen atmosphere. The homogenate was separated into cytosol and insoluble fractions by ultracentrifugation at 100
000g for 90 min at 4 °C. A portion of the cytosol fraction was heated in a water bath for 20 min at 95 °C and then centrifuged at 12
000 rpm for 20 min to obtain the heat-treated fraction. Selenosugar and TMSe were stable against the heat treatment (data not shown).
A 200 µL aliquot of the cytosol sample was applied to a multi-mode gel filtration column (GS-520HQ; exclusion size, 300
000 Da; 7.5 i.d. × 300 mm; Showa Denko, Tokyo, Japan) and the column was eluted with 50 mM Tris–HCl, pH 7.4, at a flow rate of 1.0 mL min−1. A 20 µL aliquot of the heat-treated fraction was applied to another multi-mode gel filtration column (GS-320HQ; exclusion size, 40
000 Da; 7.5 i.d. × 300 mm; Showa Denko) and an anion-exchange column (DEAE-2SW; 4.6 i.d. × 250 mm; Tosoh Corporation, Tokyo). The former column was eluted with 50 mM ammonium acetate, pH 6.5, at a flow rate of 0.6 mL min−1 and the latter was eluted with 50 mM ammonium acetate, pH 4.0, at a flow rate of 0.7 mL min−1. The eluate was introduced directly into the nebulizer tube of an ICP-MS and Se, Hg, zinc (Zn), and cadmium (Cd) were detected at m/z 82, 202, 66, and 111, respectively, in the deuterium reaction mode.17
An API3000 triple quadrupole mass spectrometer with an electrospray ionization source (ESI-MS-MS; Applied Biosystems, Tokyo) was used to identify the structure of the unknown Se compound. The ESI-MS-MS was operated in the positive ion mode under the following conditions: ion spray voltage, 5000 V; gas temperature, 450 °C; nebulizer gas, 8 units; curtain gas, 8 units; declustering potential, 20 V; focusing potential, 200 V; and entrance potential, −10 V. A 1.0 µL aliquot of the heat-treated soluble fraction at a concentration of 7 µg Se mL−1 was applied to the narrow bore HPLC equipped with a multi-mode size exclusion column of narrow bore size (Shodex GS320A-2E; exclusion size, 40
000 Da; 2.0 i.d. × 250 mm; Showa Denko). The column was eluted with 10 mM ammonium acetate, pH 6.5, at a flow rate of 40 µL min−1, and the eluate was introduced into the ionization spray of ESI-MS-MS without splitting. The introduction into the ESI-MS-MS was time-separated for Se elution using a four-way valve, i.e., the eluate was introduced from 18.3 to 19.4 min. The dissociation of Se-containing molecular ions at m/z 276 and 278 was induced with collision energies of 15, 25, and 35 eV, and fragment ions were detected with the second mass spectrometer.
Results and discussion
Se and Hg concentrations in the livers of sea turtles
Se concentrations were much higher than Hg concentrations in the livers of green and hawksbill turtles (Table 1). These concentrations were comparable to previous reports.11 Hawksbill turtles showed higher hepatic Se and Hg concentrations than green turtles, particularly in the case of Se (approximately 10-fold). It is known that the adult green turtle is herbivorous, whereas the adult hawksbill turtle feeds preferably on sea sponges. In a previous study, it was shown that Se concentration in the stomach contents of hawksbill turtles was higher than that of green turtles.11 Thus, the difference in hepatic Se concentration may be a result of their food habits. Se concentration in the liver of the snapping turtle, a terrestrial turtle, was considerably lower than those of the two sea turtles (Table 1). This suggests the habitat is also one of the reasons for the hepatic Se accumulation.
Table 1 Concentrations of selenium and mercury (µg g−1 wet weight) and their molar ratios in the livers of sea turtles and terrestrial turtle
Species |
Concentration |
Molar ratio |
Se |
Hg |
Se/Hg |
Sea turtle
|
Hawksbill turtle |
H1 |
43 |
0.29 |
378 |
H2 |
21 |
0.32 |
164 |
H3 |
35 |
0.86 |
104 |
Mean ± sd |
33 ± 11 |
0.49 ± 0.32 |
215 ± 144 |
Green turtle |
G1 |
6.9 |
0.06 |
298 |
G2 |
2.0 |
0.13 |
38 |
G3 |
3.3 |
0.15 |
56 |
Mean ± sd |
4.1 ± 2.5 |
0.11 ± 0.05 |
130 ± 145 |
Terrestrial turtle
|
Snapping turtle (n = 6) |
Mean ± sd |
0.89 ± 0.35 |
0.18 ± 0.09 |
15.4 ± 7.6 |
It is reported that Hg was co-accumulated with Se at the molar ratio of 1 in the livers of marine mammals10,18,19 and seabirds.9,10 Contrary to that report, the molar ratios of Se to Hg in the sea turtles were extremely high, suggesting that Se was solely accumulated in the livers of the sea turtles (Table 1). Our previous study revealed that the major portion of Se that accumulated in the livers of hawksbill and green turtles was recovered in the cytosol fraction,20 whereas it was accumulated in insoluble fraction of livers from marine mammals and seabirds.10 Hence, the chemical species of Se that was accumulated in the livers of sea turtles may be different from that found in the livers of marine mammals and seabirds, i.e., mercury selenide.
Distribution of Se in hepatic cytosol of sea turtles
To investigate the chemical species of Se found in the hepatic cytosol of sea turtles, speciation with HPLC-ICP-MS equipped with a multi-mode gel filtration column (GS-520HQ) was performed. Two Se peaks were observed in the spectra of the hepatic cytosol of hawksbill and green turtles (Fig. 1). The broad and small peak was eluted around the retention time of 8.9 min. This Se peak disappeared on heat treatment, suggesting that it corresponds to selenoproteins and/or Se-containing proteins (data not shown). The sharp and large peak was eluted at the retention time of 13.8 min, and was stable to the heat treatment (data not shown). Considering the chromatographic behavior and the heat stability, this peak may be due to a low molecular weight Se compound. In addition, the Se peak was solely eluted, i.e., it was not co-eluted with an Hg peak (Fig. 1, top panels). Hg was detected in the high molecular weight fraction that contained various proteins and its peak had a retention time of 7–10 min. The peak appearing at the retention time of 9.9 min contained not only Hg but also Cd. This indicates that Hg partially binds to metallothioneins in the livers of sea turtles. A part of Zn was also bound to metallothioneins. Hg was also eluted at the retention time of 11.1 min. Although the Hg species could not be identified yet, it is speculated that Hg may be bound to low molecular weight bioligand(s) on the basis of the chromatographic behavior. Therefore, sea turtles accumulated Se in the form of low molecular weight compounds in the hepatic cytosol and the accumulated Se did not interact with Hg.
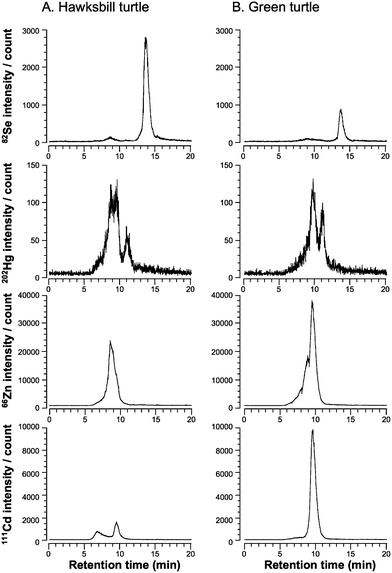 |
| Fig. 1 Elution profiles of Se, Hg, Zn, and Cd in the hepatic cytosol of hawksbill turtle (A) and green turtle (B) on a multi-mode gel filtration column detected by ICP-MS. A 200 µL aliquot of the cytosol fraction was applied to a GS-520HQ column and eluted with 50 mM Tris–HCl, pH 7.4, at a flow rate of 1.0 mL min−1. The eluate was directly introduced to ICP-MS and Se, Hg, Zn, and Cd were detected at m/z 82, 202, 66, and 111, respectively. | |
To analyze novel selenometabolites with higher resolution, a multi-mode gel filtration column (GS-320HQ) that has a smaller molecular weight range than GS-520HQ was used. To assign selenometabolites by the retention time matching with a standard, an anion-exchange column (DEAE-2SW) was also used in addition to the multi-mode gel filtration column. Several Se peaks were detected on the GS-320HQ (Fig. 2A) and DEAE-2SW (Fig. 2B) columns. Two peaks appearing at the retention times of 19.5 and 21.0 min on the GS-320HQ column were assignable to selenosugar and TMSe according to their chromatographic behavior. This is the first detection of selenosugar and TMSe in marine organisms, and suggests that those are metabolites common to terrestrial and marine animals. However, the retention time of the major Se peak (22.0 min) did not match those of any authentic Se standards used in the study, such as selenite, selenate, SeMet, and MeSeCys (data not shown). Further, the chromatographic behavior of the Se peak was quite different from those of the other Se compounds that we analyzed previously, including γ-glutamyl-Se-methylselenocysteine,21Se-methyl-selenomethionine,21Se-adenosyl-selenomethionine,22Se-adenosyl-selenohomocysteine,22 and selenohomolanthionine.21 Consequently, we conclude that the major Se compound that accumulated in the livers of the sea turtles seems to be an unknown Se compound. We tentatively named it “ukSe” and undertook its identification by ESI-MS-MS.
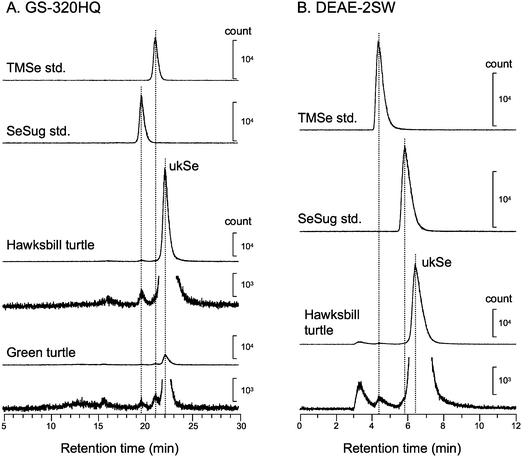 |
| Fig. 2 Elution profiles of low molecular weight Se compound in the hepatic cytosol of sea turtles. Heat-treated cytosol fractions of hawksbill turtle and green turtle were applied to a multi-mode gel filtration column, GS-320HQ column (A), and an anion exchange column, DEAE-2SW column (B). The chromatographic behavior of the Se compound from sea turtles was compared with those of authentic Se standards on both columns. The concentrations of TMSe standard and selenosugar (SeSug) standard were 1.0 µg mL−1; ukSe: unknown Se compound. | |
Identification of unknown Se compound by ESI-MS–MS
Se consists of six isotopes, 74Se (0.89%), 76Se (9.36%), 77Se (7.63%), 78Se (23.8%), 80Se (49.6%), and 82Se (8.73%). Signals showing the isotope patterns of monomeric and dimeric Se were observed at around m/z 278 (Fig. 3A and B) and 553 (Fig. 3A and C), respectively. The isotopic pattern of the monomeric Se-containing molecule did not seem to be the isotopic pattern of Se due to the unexpectedly high intensity at m/z 276 (78Se) (Fig. 3B). To make sure that it contained Se and to identify the unknown molecule, the most and second intense ions at m/z 278 and 276 were extracted and subjected to MS-MS analysis as precursor ions. All ions observed at collision energies of 15 eV, 25 eV, and 35 eV (Fig. 4A) were assignable to the fragments of ukSe, as depicted in Fig. 4C. The mass differences of the fragments between 80Se- and 78Se-containing molecules were always 2, and the intensities of the fragments containing 80Se were around double of those containing 78Se (Fig. 4A and B). These results suggest that ukSe is 2-selenyl-N,N,N-trimethyl-L-histidine, or the so-called selenoneine, which was recently detected in the blood of bluefin tuna.23 MS-MS analysis of the ion at m/z 553 gave the same fragments as that at m/z 278 (data not shown), and the fragments coincided with the results for bluefin tuna. As depicted in Fig. 4C and D, the ion at m/z 278 was assigned to [M + H]+ and the ion at m/z 553 was not a dimer but an oxidized form of the ion at m/z 278 in accordance with their m/z; namely, the ion at m/z 553 is not [2M + H]+ but [(M − H) + (M − H) + H]+ (M = 277). Although it is still unclear why both the ions at m/z 278 and 553 were simultaneously detected at the same retention time, we speculate that selenoneine is in situ oxidized during the electrospray process.
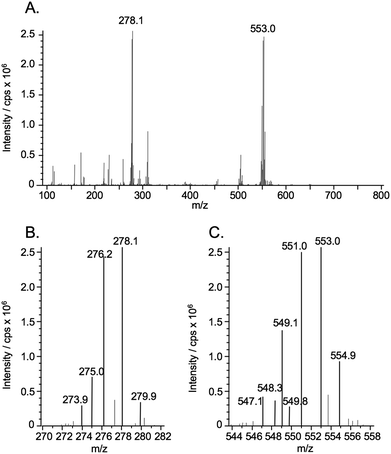 |
| Fig. 3 ESI-MS spectrum of the eluate at retention times of 20.5–21.7 min, containing unknown Se compound (ukSe) in the heat-treated soluble fraction of liver from hawksbill turtle (A). The eluate of the multi-mode size exclusion column of narrow bore size (GS320A-2E) was introduced into the ionization spray of ESI-MS without splitting. The introduction into the ESI-MS was time-separated for Se elution using a four-way valve, i.e., the eluate was introduced from 18.3 to 19.4 min. Magnified parts of the spectrum containing the characteristic isotope pattern of Se are shown in panels (B) and (C). | |
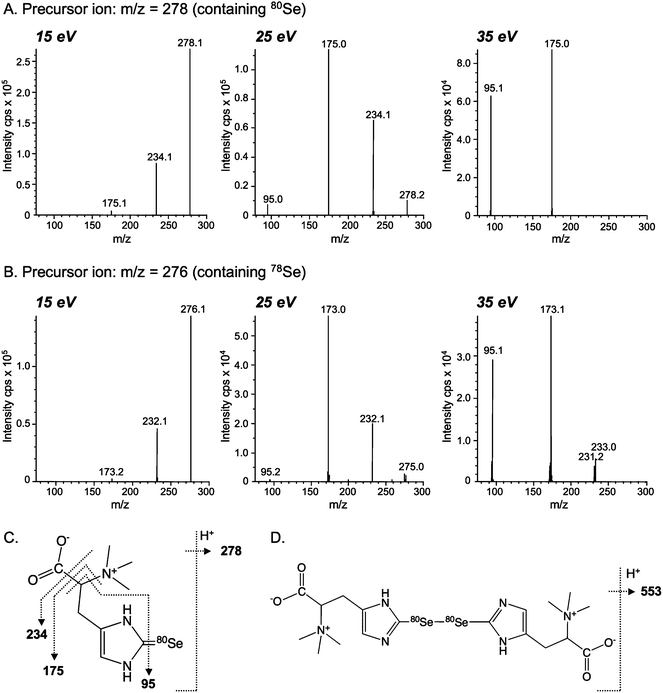 |
| Fig. 4 Collision-induced dissociation mass spectra (ESI-MS-MS) of Se-containing positive molecular ions in ukSe from the liver of hawksbill turtle. The dissociation of the Se-containing molecular ions of ukSe at m/z 278 (A) and 276 (B) were induced with 15, 25, and 35 eV collision energies, and fragment ions were detected with the second mass spectrometer. The assignments of fragments obtained at m/z 278 and 553 are shown in panels (C) and (D), respectively. | |
Conclusion
Two species of sea turtles, hawksbill turtle (E. imbricata) and green turtle (C. mydas), accumulated Se but not Hg in their livers. The major hepatic Se is a novel selenometabolite that was identified as selenoneine (2-selenyl-N,N,N-trimethyl-L-histidine) by HPLC-ESI-MS-MS. The fact that selenoneine was firstly identified in the sea turtles suggests that sea turtles possess specific mechanisms for Se metabolism, which result in the sole accumulation of Se. The biological significance and metabolism of selenoneine are unclear at present and further studies are warranted to reveal its biological and ecological significance in sea turtles.
Acknowledgements
The authors wish to thank Dr N. Kamezaki, Mr T. Shima, Mr M. Wakatsuki, and Mr S. Yamashiro for their help in sample collection. This study was supported by a Grant-in-Aid from the Ministry of Education, Culture, Sports, Science and Technology, Japan (no. 19390033 to Y.O.), and the financial support from Agilent Technologies Foundation, USA. We also wish to thank Showa Denko for providing the narrow bore column and the HPLC system.
References
- J. Lu and A. Holmgren, J. Biol. Chem., 2009, 284, 723–727 CAS.
- M. A. Reeves and P. R. Hoffmann, Cell. Mol. Life Sci., 2009, 66, 2457–2478 CrossRef CAS.
- Y. Ogra and Y. Anan, J. Anal. At. Spectrom., 2009, 24, 1477–1488 RSC.
- Y. Kobayashi, Y. Ogra and K. T. Suzuki, J. Chromatogr. B: Biomed. Sci. Appl., 2001, 760, 73–81 CrossRef CAS.
- A. Naganuma, Y. Ishii and N. Imura, Ecotoxicol. Environ. Saf., 1984, 8, 572–580 CrossRef CAS.
- S. Yoneda and K. T. Suzuki, Toxicol. Appl. Pharmacol., 1997, 143, 274–280 CrossRef CAS.
- L. Su, M. Wang, S. T. Yin, H. L. Wang, L. Chen, L. G. Sun and D. Y. Ruan, Ecotoxicol. Environ. Saf., 2008, 70, 483–489 CrossRef CAS.
- N. V. Ralston, J. L. Blackwell, 3rd and L. J. Raymond, Biol. Trace Elem. Res., 2007, 119, 255–268 CrossRef CAS.
- E. Y. Kim, K. Saeki, S. Tanabe, H. Tanaka and R. Tatsukawa, Environ. Pollut., 1996, 94, 261–265 CrossRef CAS.
- T. Ikemoto, T. Kunito, H. Tanaka, N. Baba, N. Miyazaki and S. Tanabe, Arch. Environ. Contam. Toxicol., 2004, 47, 402–413 CAS.
- Y. Anan, T. Kunito, I. Watanabe, H. Sakai and S. Tanabe, Environ. Toxicol. Chem., 2001, 20, 2802–2814 CAS.
- M. Montes-Bayón, M. J. Molet, E. B. González and A. Sanz-Medel, Talanta, 2006, 68, 1287–1293 CrossRef CAS.
- H. Goenaga-Infante, A. A. Borrego, E. Peachey, R. Hearn, G. O'Connor, T. G. Barrera and J. L. Ariza, J. Agric. Food Chem., 2009, 57, 38–45 CrossRef CAS.
- Z. Pedrero and Y. Madrid, Anal. Chim. Acta, 2009, 634, 135–152 CrossRef CAS.
- Y. Kobayashi, Y. Ogra, K. Ishiwata, H. Takayama, N. Aimi and K. T. Suzuki, Proc. Natl. Acad. Sci. U. S. A., 2002, 99, 15932–15936 CrossRef CAS.
- S. Tanabe, J. Environ. Monit., 2006, 8, 782–790 RSC.
- Y. Ogra, K. Ishiwata and K. T. Suzuki, Anal. Chim. Acta, 2005, 554, 123–129 CrossRef CAS.
- R. Wagemann, E. Trebacz, G. Boila and W. L. Lockhart, Sci. Total Environ., 2000, 261, 21–32 CrossRef CAS.
- K. Das, L. Holsbeek, J. Browning, U. Siebert, A. Birkun, Jr and J. M. Bouquegneau, Environ. Pollut., 2004, 131, 197–204 CrossRef CAS.
- Y. Anan, T. Kunito, H. Sakai and S. Tanabe, Mar. Pollut. Bull., 2002, 45, 224–229 CrossRef CAS.
- T. Kitaguchi, Y. Ogra, Y. Iwashita and K. T. Suzuki, Eur. Food Res. Technol., 2008, 227, 1455–1460 CrossRef CAS.
- Y. Ogra, T. Kitaguchi, K. Ishiwata, N. Suzuki, T. Toida and K. T. Suzuki, Metallomics, 2009, 1, 78–86 RSC.
- Y. Yamashita and M. Yamashita, J. Biol. Chem., 2010, 285, 18134–18138 CrossRef CAS.
Footnote |
† This article is part of a themed issue highlighting outstanding and emerging work in the area of speciation. |
|
This journal is © The Royal Society of Chemistry 2011 |
Click here to see how this site uses Cookies. View our privacy policy here.