DOI:
10.1039/C1FO10087D
(Paper)
Food Funct., 2011,
2, 475-482
Cranberry phytochemicals inhibit glycation of human hemoglobin and serum albumin by scavenging reactive carbonyls†
Received
29th May 2011
, Accepted 11th July 2011
First published on 8th August 2011
Abstract
Protein glycation caused by sugars and reactive carbonyls is a contributing factor to diabetic complications, aging, and other chronic diseases. The objective of this study was to investigate the inhibitory effects of cranberry phytochemicals on protein glycation. Cranberries, purified to yield sugar-free phytochemical powder, were fractionated into ethyl acetate and water fractions. Water fraction was further separated into water fraction I, II, and III on a Sephadex LH-20 column. Cranberry phytochemical powder and its fractions significantly inhibited the formation of glycated hemoglobin. The concentrations of cranberry phytochemicals required to inhibit 50% of albumin glycation (EC50) in albumin-glucose assay were lower than that of aminoguanidine except for water fraction I. Cranberry phytochemicals inhibited glycation of human serum albumin mediated by methylglyoxal, but the EC50 were higher than that of aminoguanidine. Carbonyl scavenging assay showed that water fraction II scavenged 89.3% of methylglyoxal at 6 h of reaction. Fractions enriched with procyanidins showed higher antiglycation activities, suggesting procyanidins were the major active components. The hypothesis whether cranberry procyanidins scavenged reactive carbonyls by forming adducts was tested. Epicatechin was used as a model compound to react with methylglyoxal and glyoxal at pH 7.4. Five adducts were detected and their structures were tentatively identified using HPLC-ESI-MS/MS.
1. Introduction
Protein glycation, a reaction between carbonyl groups of reducing sugars and amino groups of protein, has been demonstrated to be a major pathogenic factor in diabetes,1 advanced aging,2 Alzheimer's disease,2 and renal failure.3 Advanced glycation endproducts (AGEs) are a group of complex and heterogeneous compounds generated during protein glycation.4 In one mechanism, reducing sugars such as glucose react with the primary amino groups to form a Schiff base, which undergo Amadori rearrangement to generate stable Amadori products.5 Amadori products go through a complex series of reactions including further rearrangement, oxidation, dehydration, and polymerization to yield the advanced glycation endproducts.5 In an alternative mechanism, reactive di-carbonyls such methylglyoxal, glyoxal, or 3-deoxyglucosone react with lysine or arginine residue on cellular or tissular proteins to form AGEs.6,7 Reactive di-carbonyls are produced from sugar glycoxidation,8degradation of Schiff base, and oxidation of Amadori products.7 Accumulation of reactive di-carbonylsin vivo leads to structural and functional modifications of proteins and lipids, and causes oxidative stress and vascular damages.3,9
Cranberry (Vaccinium macrocarpon), a native plant of North America,10 is a rich source of phytochemicals including anthocyanins10,11 and procyanidins.12 Phytochemicals in cranberries have been found to possess antiproliferative,13 anticarcinogenic,14 antitumorigenic,10 antimutagenic,15 and antioxidant activities.16,17 Previous studies showed that polyphenols extracted from cinnamon bark and mung bean inhibited protein glycation and the formation of AGEs.18,19Phloretin in apples and genistein from soy were methylglyoxal scavengers.20,21 No study has been conducted to investigate the capacity of cranberry phytochemicals to scavenge reactive carbonyls and inhibit protein glycation. On the basis of the high phenolic content and antioxidant capacities of cranberries,16,17 we hypothesized that cranberry phytochemicals may inhibit protein glycation and the formation of AGEs by scavenging reactive dicarbonyls. The present study was to test these hypotheses.
2. Materials and methods
2.1 Chemicals and materials
Freeze-dried cranberries were provided by Ocean Spray Cranberries, Inc (Lakeville-Middleboro, MA). Human serum albumin was a product from Lee Biosolutions, Inc. (St. Louis, MO). Sodium azide, phosphate buffer saline (pH 7.4), D-glucose, and HPLC grade solvents used were purchased from Fisher Scientific Co. (Pittsburg, PA). Aminoguanidine, o-phenylenediamine, and glyoxal (40% aqueous solution) were from Acros Organics (Morris Plains, NJ). Methylglyoxal (40% aqueous solution) was obtained from MP Biomedical, LLC (Solon, OH). (-)-Epicatechin, and Sephadex LH-20 resin were purchased from Sigma-Aldrich (St. Louis, MO). Amberlite FPX 66 resin was a product of Rohm and Haas Co. (Philadelphia, PA).
2.2 Cranberry phytochemical extraction, fractionation, and characterization
The procedure is depicted in Fig. 1. One kilogram of freeze-dried cranberries was extracted with 4 L of acidified (0.5% acetic acid) methanol at room temperature for 48 h. The extraction was repeated once. Extracts obtained after vacuum filtration were combined and concentrated under partial vacuum using a rotary evaporator. Seven hundred and forty grams (740 g) of crude extract was obtained in a form of slurry. Part of this extract (60 g) was suspended in 30 mL of water and loaded onto a column (2.8 × 55 cm) packed with Amberlite FPX 66 resins. Column was eluted with 3 L of 1% acetic acid water to remove sugars followed by 1 L of methanol to yield cranberry phytochemical powder (5.55 g). Part of this powder (5.05 g) was suspended in 300 mL of water, and partitioned with 300 mL ethyl acetate for three times in a separation funnel. The ethyl acetate phases were combined and evaporated to yield 0.86 g of extract. The aqueous phase was dried in a rotary evaporator at 40 °C to yield the water fraction (4.06 g). An extra water fraction was prepared by repeating the previous fractionation steps. One part of water fraction was dissolved in a 30% methanol solution and loaded onto a Sephadex LH-20 column (5.8 × 28 cm). The column was eluted with 30% methanol (3.5 L), 70% methanol (2.5 L), and 70% acetone (1 L) to yield three fractions that weighed 1.08 g, 1.40 g, and 0.73 g, respectively.
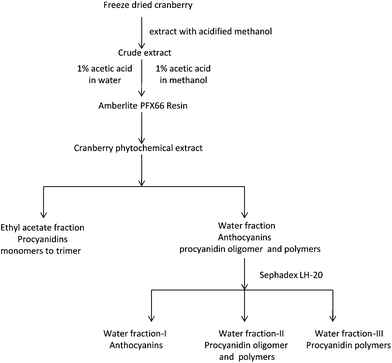 |
| Fig. 1
Extraction and fractionation procedure of cranberry phytochemicals. Major phytochemicals in each fraction are noted. | |
The total phenolic content was determined by Folin-Ciocalteu assay.22 A pH differential assay was used to determine the total anthocyanin content.23 The results were expressed as cyanidin 3-glucoside equivalents. Total procyanidins in berry and grape extracts were determined by 4-dimethylaminocinnamaldehyde (DMAC) colorimetric method using epicatechin as a standard.24
2.3 Analysis of sugars in cranberry phytochemical powder
Sugar analysis was conducted using a Hitachi HPLC system with a refractive index detector and a Shodex SP0810 column (300 mm × 8 mm, Shodex, Colorado Springs, CO) with a SP-G guard column (2 mm × 4 mm). An isocratic flow rate of water was run at 1.0 mL min−1. Cranberry phytochemical powder was dissolved in water to a concentration of 15 mg mL−1. Sample injection volume was 5 μL. Sucrose, glucose, fructose, lactose, and maltodextrin were used as standards.
This assay evaluates the early stage of protein glycation by measuring the formation of glycated human hemoglobin (HbA1c). Published methods were used with modifications.25–27Glucose and cranberry phytochemicals were dissolved in phosphate buffer (pH 7.4) to a concentration of 625 mM and 10 mg mL−1, respectively. Human blood was from a healthy volunteer. The fresh blood (400 μL) was mixed with glucose (80 μL) and cranberry phytochemical powder or fractions (20 μL). The final concentrations of glucose and cranberry phytochemical fractions were 100 mM and 0.4 mg mL−1, respectively. Blood was incubated with phosphate buffer without or with 100 mM glucose as blank or positive controls. After incubation at 37 °C for 4 days, the percentage of glycated hemoglobin was determined using an immunoassay-based automatic analyzer (DCA 2000 + Analyzer, Bayer Co. Elkhart, IN). Experiments were conducted in duplicates. Aminoguanidine, a drug candidate for treating diabetic complications, was used at a final concentration of 10 mM to compare with cranberry phytochemicals.
2.5 Human serum albumin (HSA)-methylglyoxal assay
A modified method based on Bovine serum albumin-methylglyoxal was used.18 This assay evaluates the middle stage of protein glycation. HSA and methylglyoxal were dissolved in phosphate buffer (50 mM, pH 7.4) to a concentration of 30 mg mL−1 and 60 mM, respectively. Cranberry phytochemical powder and its fractions were dissolved in the same phosphate buffer. One mL of the HSA solution was mixed with 1 mL of methylglyoxal solution, and 1 mL cranberry fraction and the mixture was incubated at 37 °C. Sodium azide (0.2 g L−1) was used as an aseptic agent. Phosphate buffer was used as blank. Aminoguanidine was used as a positive control. After six days of incubation, fluorescence of samples was measured using an excitation of 340 nm and an emission of 420 nm. The % inhibition of AGEs formation = [1 − (fluorescence of the test group/fluorescence of the control group)] × 100%.
2.6
HSA-glucose assay
This method was modified based on BSA-glucose assay.18 This assay evaluates protein glycation mediated by glucose. Human serum albumin and glucose were dissolved in phosphate buffer (50 mM, pH 7.4) to a concentration of 50 mg mL−1 and 0.8 M, respectively. Cranberry phytochemical powder or fractions were dissolved in the same phosphate buffer. One mL of the HSA solution was mixed with 1 mL of glucose solution and 1 mL of cranberry fraction. The mixtures were incubated at 37 °C. Sodium azide (0.2 g L−1) was used as an aseptic agent. Phosphate buffer was used as blank. Aminoguanidine was used as a positive control. After six days of incubation, fluorescence of samples was measured using an excitation of 330 nm and an emission of 410 nm, respectively. The % inhibition of AGEs formation = [1 − (fluorescence of the test group/fluorescence of the control group)] × 100%.
Methylglyoxal trapping assay followed a published method with modifications.18Methylglyoxal (10 mM), o-phenylenediamine (derivatization agent, 50 mM) were freshly prepared in phosphate buffer (50 mM, pH 7.4). Cranberry fractions were dissolved in the same buffer to a concentration of 2.5 mg mL−1. Methylglyoxal solution (0.125 mL) was mixed with 0.125 mL of phosphate buffer (blank) or test samples. The mixtures were incubated at 37 °C for 0.5, 1, 2, 4, 6, and 12 h respectively. After incubation, 0.25 mL of o-phenylenediamine was added to each test solution. The mixtures were kept for 30 min at room temperature for the completion of derivatization reaction before they were injected for HPLC analysis (Agilent Technologies, Palo Alto, CA). Compound separation was carried out on a Zorbax SB-C18 column (4.6 × 250 mm, 5 μm, Agilent Technologies, Palo Alto, CA). Mobile phases were composed of 0.1% formic acid in water (phase A) and methanol (phase B). The flow rate was set as 1 mL min−1 and the injection volume was 15 μl. The linear gradient for elution was: 0–3 min, 5–50% B; 3–16 min, 50–50% B; 16–17 min, 50–90% B; 17–19 min, 90–90% B; 19–19.5 min, 90–5% B; followed by 1 min of re-equilibration. Methylglyoxal reacted with o-phenylenediamine to form 1-methylquinoxaline which eluted at 12.9 min using detection wavelength of 315 nm.
2.8
Epicatechin-methylglyoxal/glyoxal reaction and adduct identification
Epicatechin, methylglyoxal, and glyoxal were dissolved in phosphate buffer (50 mM, pH 7.4). O-phenylenediamine (50 mM) was prepared in same phosphate buffer as a derivatization agent for glyoxal and methylglyoxal. Epicatechin (10 mM, 2 mL) was mixed with 2 mL of methylglyoxal or glyoxal (10 mM) and incubated at 37 °C. Six hours after incubation, a 125 μL aliquot was removed from incubation media and was mixed with 125 μL of o-phenylenediamine to terminate the phytochemical-carbonyl reaction, followed by HPLC-ESI-MS/MS analysis. An Agilent 1200 HPLC system (Agilent Technologies, Palo Alto, CA) equipped with a fluorescence detector and HCT mass spectrometer (Bruker Daltonics, Billerica, MA) were used for adduct identification. Compound separation was carried out on a Zorbax SB-C18 column (4.6 × 250 mm, 5 μm, Agilent Technologies, Palo Alto, CA). Mobile phases were composed of 0.1% formic acid in water (phase A) and methanol (phase B). The linear gradient was: 0–5 min, 10–22.5% B; 5–15 min, 22.5–27.5% B; 15–18 min, 27.5–70% B; 18–21 min, 70–90% B; 21–23 min, 90–10% B, followed by 2 min of re-equilibration. The detection wavelength on fluorescence detector was 230 nm for excitation and 321 nm for emission. Electrospray ionization at both positive and negative modes was performed using nebulizer 50 psi, drying gas 10 L min−1 and drying temperature 300 °C. Two precursor ions with highest intensities were isolated and fragmented to obtain the product ion spectra of adducts.
2.9 Statistical analysis
Half inhibition concentrations (EC50) were determined using Probit analysis function of SPSS software (Version 13, SPSS Inc., Chicago, IL.) One way ANOVA with Tukey-Kramer HSD test were done using JMP software (Version 8.0, SAS Institute Inc., Cary, NC) and a difference of p ≤ 0.05 was considered as significant. All data were expressed as the mean ± standard deviation.
3. Results and discussion
A cranberry phytochemical powder was obtained using Amberlite FP × 66 resins. The amount of procyanidins and anthocyanins contained in this powder are indicated in Table 1. No free sugar was detected using HPLC with a refractive index detector. This is because polystyrene copolymer resins absorb polyphenols from the aqueous phase but not sugars.28Fractionation of cranberry phytochemical powder was designed to separate phytochemicals according to their differences in solubility and polarities. Water fraction of the cranberry phytochemical power had significantly higher amount of procyanidins and anthocyanins than ethyl acetate fraction. Water fraction I showed the least amount of total phenolic compounds and procyanidins, but contained higher amounts of anthocyanins. The contents of total phenolic compounds and procyanidins were the highest in water fraction II and water fraction III, while the amounts of anthocyanins were significantly lower than other fractions except for ethyl acetate fraction. The total procyanidin and total phenolic content had a correlation coefficient of r = 0.97, suggesting procyanidins were the major phenolic compounds in these fractions. The total anthocyanin content correlated negatively with the total phenolic content and procyanidin content with coefficient r = −0.66 and r = −0.68, respectively (see ESI†). Procyanidin profiles of cranberry extracts and fractions were assessed using HPLC-ESI-MS/MS (see ESI†).29Ethyl acetate and water fraction I contained procyanidin monomer, dimers, and trimers. Procyanidins oligomers and polymers were found in water fraction and water fraction II. Water fraction III contained exclusively polymeric procyanidins.
Table 1 Total phenolic, total procyanidin, and total anthocyanin contents of cranberry phytochemical powder and its fractionsa,b
Cranberry fractions |
Total phenolic content (mg gallic acid equivalents/g) |
Total procyanidin content (mg epicatechin equivalents/g) |
Total anthocyanin content (mg cyanidin 3-glucoside equivalents/g) |
Data are mean ± standard deviation of triplicate tests. Concentrations in mmol equivalents/g are shown in parenthesis.
Values with different superscript numbers differed significantly at p ≤ 0.05.
|
Cranberry phytochemical powder |
366.7 ± 18.62 (2.16) |
38.5 ± 0.554 (0.13) |
19.8 ± 0.33 (0.04) |
Ethyl acetate fraction |
338.3 ± 21.62 (1.99) |
31.4 ± 1.15 (0.11) |
9.9 ± 0.45 (0.02) |
Water fraction |
407.0 ± 26.62 (2.39) |
43.4 ± 2.73 (0.15) |
28.6 ± 0.42 (0.06) |
Water fraction I |
143.7 ± 9.23 (0.84) |
1.9 ± 0.16 (0.006) |
36.0 ± 0.21 (0.07) |
Water fraction II |
753.8 ± 61.01 (4.43) |
67.8 ± 1.11 (0.23) |
16.3 ± 0.14 (0.03) |
Water fraction III |
697.6 ± 17.51 (4.10) |
62.1 ± 1.92 (0.21) |
5.6 ± 0.46 (0.01) |
The inhibitory effect of cranberry phytochemical powder and its fractions on the formation of HbA1C is depicted in Fig. 2. The blood was from a healthy volunteer and had an HbA1C of 3.75% after incubating with phosphate buffer for four days. The HbA1C level was 7.05% after blood was incubated with 100 mM of glucose. Results showed that all cranberry fractions significantly inhibited HbA1C formation, compared to the glucose control. Cranberry phytochemical powder and its water fraction and water fraction III decreased HbA1C levels to 5.75, 5.55, and 5.45%, respectively. Aminoguanidine did not inhibit HbA1C formation in this assay. This is because HbA1C is a product of early stage of protein glycation and the reaction does not involve reactive carbonyls. Aminoguanidine is a carbonyl scavenger that does not inhibit the early stage of protein glycation.30
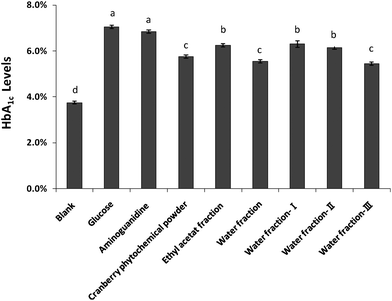 |
| Fig. 2 Effect of cranberry phytochemicals on the formation of glycated hemoglobin (HbA1C). Results are means ± standard deviation of duplicate assay. Bars with different letters differed significantly (p < 0.05). | |
Glycated human hemoglobin (HbA1c) is formed by the attachment of glucose to the N-terminal amino group of the β-chains of hemoglobin, forming a Schiff base which can slowly undergo an Amadori rearrangement to generate stable Amadori products.31 Level of glycated human hemoglobin (HbA1c) is routinely measured in diabetic patients to monitor risk for complications. The American Diabetes Association recommends that the HbA1c be below 7.0%.32In vitro, HbA1c can be synthesized by incubating glucose with hemoglobin in human blood.5 This model provides insight into early stage of nonenzymatic protein glycation.33,34 Flavan-3-ols were shown to inhibit the formation of HbA1c.33 This may in part explain the inhibitory effects of cranberry phytochemicals because procyanidins in cranberries consisted of flavan-3-ols. Water fraction and water fraction III appeared as effective as cranberry phytochemical powder in inhibiting the formation of HbA1c. Ethyl acetate fraction, water fraction I and II showed lower activity than the cranberry phytochemical powder. Such differences in anti-glycation activity may be due to different phytochemical composition in various fractions.
3.3
HSA-methylglyoxal and HSA-glucose assays
In HSA-methylglyoxal assay, cranberry phytochemical powder and its fractions significantly inhibited protein glycation mediated by methylglyoxal and the inhibitory effects increased with concentrations (Fig. 3A). The concentrations of cranberry phytochemicals required to inhibit 50% of albumin glycation were calculated. The water fraction II and ethyl acetate fraction had lower EC50, hence were more effective than other cranberry fractions (Table 2). However, all fractions were less effective than aminoguanidine to inhibit HSA glycation mediated by methylglyoxal due to a higher EC50. Similarly, in the HSA-glucose assay, all cranberry fractions inhibited the glucose-mediated protein glycation in a concentration-dependent manner (Fig. 3B). Water fraction II exhibited the highest inhibitory activity on the formation of AGEs, with the lowest EC50 values of 0.021 mg mL−1. All cranberry fractions, except water fraction I, were more than or as effective as aminoguanidine with the EC50 values of 0.064 mg mL−1 (Table 2), a drug candidate to treat diabetic complications.8
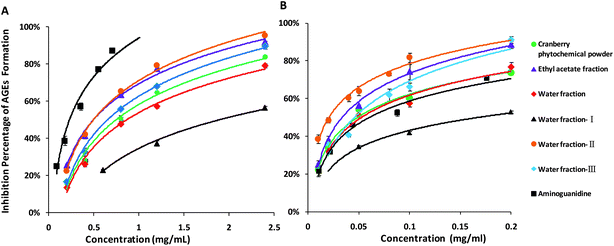 |
| Fig. 3 Glycation inhibitory effects of cranberry phytochemicals in human serum albumin (HSA)-methylglyoxal assay (A) and HSA-glucose assay (B). Results are means ± standard deviation of triplicate assay. | |
Table 2
EC50 of cranberry extracts to inhibit protein glycation in two assaysa,b
Cranberry fractions |
EC50 (mg mL−1) |
HSA-MGO assay |
HSA-Glucose assay |
Data are mean ± standard deviation of triplicate tests.
Values with different superscript numbers differed significantly at p ≤ 0.05.
|
Cranberry phytochemical powder |
0.75 ± 0.023 |
0.047 ± 0.0043 |
Ethyl acetate fraction |
0.48 ± 0.015 |
0.035 ± 0.0034 |
Water fraction |
0.87 ± 0.022 |
0.052 ± 0.0042,3 |
Water fraction-I |
1.89 ± 0.031 |
0.174 ± 0.0081 |
Water fraction-II |
0.48 ± 0.085 |
0.021 ± 0.0025 |
Water fraction-III |
0.65 ± 0.024 |
0.045 ± 0.0043,4 |
Aminoguanidine
|
0.23 ± 0.016 |
0.064 ± 0.0032 |
Reactive carbonyls such as methylglyoxal, glyoxal, and 3-deoxyglucosone are formed from the degradation and oxidation of Amadori products7 in the middle stage of protein glycation. Alternatively, these key intermediates can be generated by the glucose glycoxidation and lipid peroxidation.8HSA-methylglyoxal model evaluates antiglycation activities of cranberry phytochemicals in the middle stage. HSA-glucose model assesses the effects of cranberry phytochemicals on all stages of nonenzymatic glycation. The EC50 of cranberry extracts and fractions in HSA-methylglyoxal correlated with those in HSA-glucose assays with r = 0.99. They correlated negatively with total procyanidin content with r = −0.81 and r = −0.84, respectively (see ESI†). These results suggested procyanidins were the major antiglycation compounds in both assays. Procyanidin dimers and trimers had been shown to be absorbed into blood circulation.35 Larger procyanidins depolymerized into (+)-catechin and (-)-epicatechin in the gastrointestinal tract and the resultant compounds were absorbed.36 These absorbed procyanidins were expected to have antiglycation activities in vivo. Phenolic acids were shown to be the major urinary metabolites in rats fed cranberry powder.37 The antiglycation activities of phenolic acid such as gallic acid were also reported in a previous study.38 Our results on cranberry phytochemicals were consistent with a previous research in which the catechin, epicatechin, and procyanidins B2 were demonstrated to inhibit protein glycation mediated by methylglyoxal and glucose.18 The ethyl acetate fraction showed potent anti-glycation activities that were similar to water fraction II in HSA-methylglyoxal system. Such activities were attributed to procyanidin momers, dimers, and trimers. Besides procyanidins, mass spectral data suggested the presence of quercetin pentosides and hexosices in ethyl acetate fraction. Quercetin was known to have much lower anti-glycation activities than flavan-3-ols.33,38Water fraction I, which contained higher amounts of anthocyanins, had lower glycation inhibitory effects than other fractions. This observation was consistent with a previous study where anthocyanins extracted from purple sweet potato inhibited fructose-mediated protein glycation.39Anthocyanins were known to react slowly with pyruvic acid and acetaldehyde to form pyranoanthocyanins in wine.40 The reaction between anthocyanins and di-carbonyls were not reported. Its kinetics and reaction products remain unknown.
The methylglyoxal scavenging curves of cranberry phytochemicals are demonstrated in Fig. 4. Methylglyoxal content decreased significantly after incubation with all cranberry fractions for 1 h. About 9.1% and 5.1% of methylglyoxal remained, respectively, after incubating with water fraction II and water fraction III for 12 h. Aminoguanidine scavenged 84% of methylglyoxal after 0.5 h of incubation. Water fraction II and III appeared to be the most effective methylglyoxal scavenger, followed by ethyl acetate fraction, water fraction, and cranberry phytochemical powder (Fig. 4). The results were consistent with those in HSA-methylglyoxal. They were also in agreement with earlier research where oligomeric and polymeric procyanidins in cinnamon bark extract trapped methylglyoxal by forming the dicarbonyl-procyanidins adducts.18 Procyanidins were the major phenolic compound in cranberries. Thiolysis and mass spectrum data showed that cranberry procyanidins consisted of (−)-epicatechin that were linked through inter-flavan bonds.29 Results from this research suggested procyanidins in cranberries could be the active components that inhibited protein glycation and scavenged reactive carbonyls.
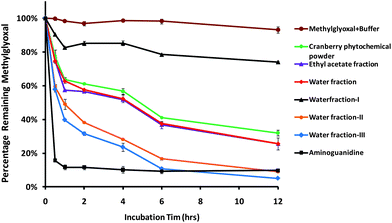 |
| Fig. 4 The capacities of cranberry phytochemicals to scavenge methylglyoxal. Aminoguanidine was used as a positive control. Results are means ± standard deviation of duplicate assay. | |
3.5
Epicatechin-carbonyl adduct identification
Incubating (−)-epicatechin in pH 7.4 phosphate buffer led to the generation of (−)-catechin due to epimerization of (−)-epicatechin29 (Fig. 5I). (−)-Epicatechin content decreased after reacting with methylglyoxal or glyoxal, and five epicatechin-carbonyl adducts were detected (Fig. 5II, III). Product ion spectra of these adducts are shown in Fig. 6. Structures of adducts and major fragments are shown in Fig. 7.
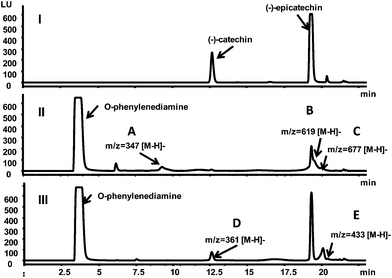 |
| Fig. 5 Fluorescent chromatograms (excitation 231 nm, emission 320 nm) of epicatechin after incubated with phosphate buffer (I), glyoxal (II), and methylglyoxal (III) for six hours. Peaks of identified adducts were labeled as A, B, C, D, and E. | |
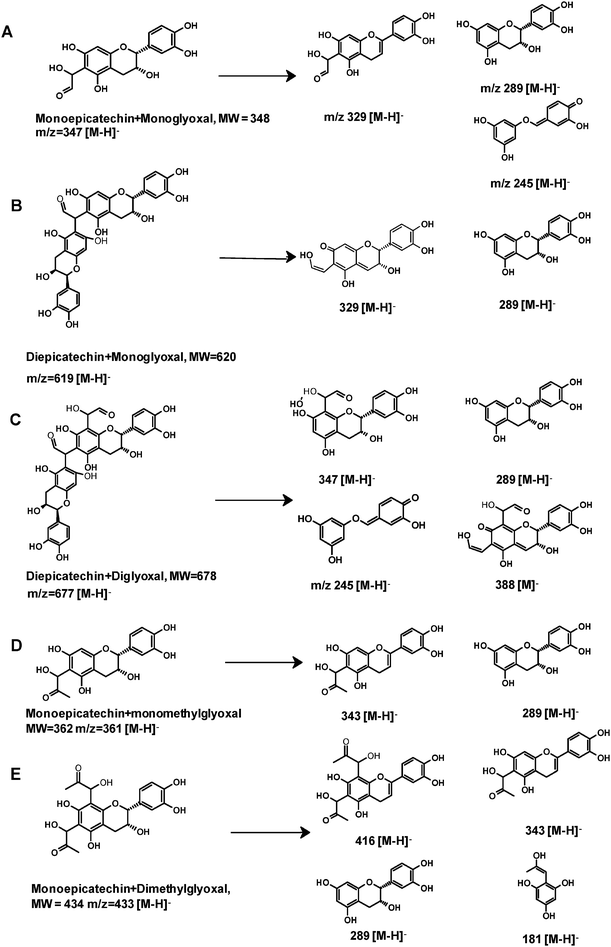 |
| Fig. 7 The proposed structures of epicatechin-glyoxal adducts and epicatechin-methylglyoxal adducts and their fragments. Letters A to E correspond to those in Fig. 5 and 6. | |
The first adduct gave m/z 347 [M − H]− and was tentatively identified as a monoepicatechin-monoglyoxal adduct (Fig. 6A). The fragment at m/z 329 was due to water elimination from m/z 347 [M − H]−. Fragment of m/z 289 was resulted from epicatechin. Epicatechin lost C3–C4 to form m/z 245.41 It has been reported that epicatechin could react with glyoxal or methylglyoxal at the C6 or C8 position.42 Because the exact position of glyoxal addition could not be determined on the basis of mass spectra, a C6 isomer is shown in Fig. 7A as an example. Because procyanidins in cranberries consisted of (−)-epicatechin that were linked mainly via C4–C8 inter-flavan bonds,23 the C6 of epicatechin in cranberry procyanidins were deduced to be major site for adduct formation.
The second adduct had m/z 619 [M − H]− and was consistent with an adduct that composed of two epicatechin molecules and a glyoxal molecule in between. It was tentatively identified as a diepicatechin-monoglyoxal adduct (Fig. 6B). The proposed structure of an isomer is shown in Fig. 7B. This adduct lost an epicatechin to yield a fragment m/z 329 [M-epicatechin-H]−. The fragment m/z 289 [M − H]− was epicatechin. The third adduct yielded m/z 677 [M − H]− and was tentatively identified as a diepicatechin-diglyoxal adduct (Fig. 6C). Proposed structure of an isomer is shown in Fig. 7C. It produced fragment at m/z 388 after losing an epicatechin moiety. Fragment at m/z 347 was generated after losing one glyoxal moiety and one epicatechin moiety. The fragment at m/z 289 [M − H]− was epicatechin. Cross-linking of two epicatechin molecules by aldehydes had been reported.43 We speculated that the reaction began with protonation of glyoxal, which was attacked by one epicatechin molecule through nucleophilic addition to form a monoepicatechin-monoglyoxal adduct. The monomer glyoxal-epicatechin intermediate continued to lose one water molecule, leading to a new carbocation.43,44 The new carbocation then attacked another epicatechin unit to yield an ethyl-linked diepicatechin-monoglyoxal adduct.
Similarly, in the reaction mixture of epicatechin and methylglyoxal, we detected and tentatively identified two adducts. An adduct gave m/z 361 [M − H]− and was tentatively identified as a monoepicatechin-monomethylglyoxal adduct (Fig. 6D). A proposed structure of an isomer is shown in Fig. 7D. Fragments of m/z 289 and 245 were resulted from epicatechin. The fragment at m/z 329 was yielded after losing a water moiety. The second adduct yielded m/z 433 [M − H]− and was tentatively identified as a monoepicatechin-dimethylglyoxal adduct (Fig. 6E). It yielded an epicatechin fragment at m/z 289 and m/z 416 due to water elimination. The fragment at m/z 343 was yielded after losing a methylglyoxal moiety. The fragment m/z 181 was deduced to be a methylglyoxal linked with a phloroglucinol molecule after water elimination. Proposed structures for adducts and fragments are shown in Fig. 7E.
4. Conclusions
In conclusion, our study showed that cranberry phytochemicals inhibited the glycation of human hemoglobin and glycation of human serum albumin mediated by methylglyoxal and glucose, respectively. The inhibitory effect of cranberry phytochemicals was attributed in part to their abilities to scavenge reactive carbonyls. Procyanidins appeared to be the major active compounds of cranberries in this in vitro study. Its ability to react with reactive carbonyl was the major mechanism.
Acknowledgements
This research is supported in part by Ocean Spray Cranberries inc.
References
- N. Ahmed, Diabetes Res. Clin. Pract., 2005, 67, 3–21 CrossRef CAS.
- G. Münch, J. Thome, P. Foley, R. Schinzel and P. Riederer, Brain Res. Rev., 1997, 23, 134–143 CrossRef.
- D. Raj, D. Choudhury, T. Welbourne and M. Levi, Am. J. Kidney Dis., 2000, 35, 365–380 CrossRef CAS.
- R. Singh, A. Barden, T. Mori and L. Beilin, Diabetologia, 2001, 44, 129–146 CrossRef CAS.
- J. Burn, Br. Med. J., 1957, 1, 149 CrossRef.
- K. J. Wells-Knecht, D. V. Zyzak, J. E. Litchfield, S. R. Thorpe and J. W. Baynes, Biochemistry, 1995, 34, 3702–3709 CrossRef CAS.
- P. Thornalley, A. Langborg and H. Minhas, Biochem. J., 1999, 344, 109 CrossRef CAS.
- A. Negre Salvayre, C. Coatrieux, C. Ingueneau and R. Salvayre, Br. J. Pharmacol., 2008, 153, 6–20 CrossRef CAS.
- J. Onorato, S. Thorpe and J. Baynes, Ann. N. Y. Acad. Sci., 1998, 854, 277–290 CrossRef CAS.
- X. Yan, B. T. Murphy, G. B. Hammond, J. A. Vinson and C. C. Neto, J. Agric. Food Chem., 2002, 50, 5844–5849 CrossRef CAS.
- T. Fuleki and F. Francis, J. Food Sci., 1968, 33, 471–478 CrossRef CAS.
- R. Prior, S. Lazarus, G. Cao, H. Muccitelli and J. Hammerstone, J. Agric. Food Chem., 2001, 49, 1270–1276 CrossRef CAS.
- N. P. Seeram, L. S. Adams, M. L. Hardy and D. Heber, J. Agric. Food Chem., 2004, 52, 2512–2517 CrossRef CAS.
- J. Bomser, D. Madhavi, K. Singletary and M. Smith, Planta Med., 1996, 62, 212–216 CrossRef CAS.
- D. Vattem, H. Jang, R. Levin and K. Shetty, J. Food Biochem., 2006, 30, 98–116 CrossRef CAS.
- I. Heinonen, P. Lehtonen and A. Hopia, J. Agric. Food Chem., 1998, 46, 25–31 CrossRef CAS.
- S. Wang and A. Stretch, J. Agric. Food Chem., 2001, 49, 969–974 CrossRef CAS.
- X. Peng, K.-W. Cheng, J. Ma, B. Chen, C.-T. Ho, C. Lo, F. Chen and M. Wang, J. Agric. Food Chem., 2008, 56, 1907–1911 CrossRef CAS.
- X. Peng, Z. Zheng, K.-W. Cheng, F. Shan, G.-X. Ren, F. Chen and M. Wang, Food Chem., 2008, 106, 475–481 CrossRef CAS.
- L. Lv, X. Shao, H. Chen, C.-T. Ho and S. Sang, Chem. Res. Toxicol., 2011, 24, 579–586 CrossRef CAS.
- X. Shao, N. Bai, K. He, C. T. Ho, C. S. Yang and S. Sang, Chem. Res. Toxicol., 2008, 21, 2042–2050 CrossRef CAS.
- V. Singleton and J. Rossi Jr, Am. J. Enol. Vitic., 1965, 16, 144 CAS.
-
M. M. Giusti and R. E. Wrolstad, Characterization and Measurement of Anthocyanins by UV-Visible Spectroscopy, John Wiley & Sons, Inc., 2001 Search PubMed.
- R. Prior, E. Fan, H. Ji, A. Howell, C. Nio, M. Payne and J. Reed, J. Sci. Food Agric., 2010, 90, 1473–1478 CrossRef CAS.
- R. M. Lindsay, W. Smith, W. K. Lee, M. H. Dominiczak and J. D. Baird, Clin. Chim. Acta, 1997, 263, 239–247 CrossRef CAS.
- S. Rahbar and J. L. Nadler, Clin. Chim. Acta, 1999, 287, 123–130 CrossRef CAS.
- R. Flückiger and K. H. Winterhalter, FEBS Lett., 1976, 71, 356–360 CrossRef.
-
L. Dravenstadt, P. Lent, M. Gomez, H. Mantius, L. Rose, S. Nojeim, C. Mcnamara and J. Johnson, WO Patent WO/2010/121,203, 2010.
- L. Gu, M. Kelm, J. Hammerstone, G. Beecher, D. Cunningham, S. Vannozzi and R. Prior, J. Agric. Food Chem., 2002, 50, 4852–4860 CrossRef CAS.
- X. Peng, J. Ma, F. Chen and M. Wang, Food Funct., 2011, 2, 289 CAS.
- H. Bunn, D. Haney, K. Gabbay and P. Gallop, Biochem. Biophys. Res. Commun., 1975, 67, 103–109 CrossRef CAS.
-
Diabetes Care, 2009, 32, pp. S3–S5 Search PubMed.
- C.-H. Wu and G.-C. Yen, J. Agric. Food Chem., 2005, 53, 3167–3173 CrossRef CAS.
- S. Rahbar and J. Figarola, Arch. Biochem. Biophys., 2003, 419, 63–79 CrossRef CAS.
- C. Tsang, C. Auger, W. Mullen, A. Bornet, J. M. Rouanet, A. Crozier and P. L. Teissedre, Br. J. Nutr., 2005, 94, 170–181 CrossRef CAS.
- L. Gu, S. E. House, L. Rooney and R. L. Prior, J. Agric. Food Chem., 2007, 55, 5326–5334 CrossRef CAS.
- R. L. Prior, T. R. Rogers, R. C. Khanal, S. E. Wilkes, X. Wu and L. R. Howard, J. Agric. Food Chem., 2010, 58, 3940–3949 CrossRef CAS.
-
K. V. Tarwadi and V. V. Agte, Biological Trace Element Research, pp. 1–9 Search PubMed.
- K. Park, J. Kim, J. Lee, H. Lee and K. Cho, J. Med. Food, 2010, 13, 91–98 CrossRef CAS.
- A. Morata, F. Calderón, M. C. González, M. C. Gómez-Cordovés and J. A. Suárez, Food Chem., 2007, 100, 1144–1152 CrossRef CAS.
- S. Pérez-Magariño, I. Revilla, M. L. González-SanJosé and S. Beltrán, J. Chromatogr., A, 1999, 847, 75–81 CrossRef.
- V. M. Totlani and D. G. Peterson, J. Agric. Food Chem., 2006, 54, 7311–7318 CrossRef CAS.
- N. Es-Safi, V. Cheynier and M. Moutounet, J. Agric. Food Chem., 2002, 50, 5571–5585 CrossRef CAS.
- C. Timberlake and P. Bridle, Am. J. Enol. Vitic., 1976, 27, 97 CAS.
Footnote |
† Electronic supplementary information (ESI) available: See DOI: 10.1039/c1fo10087d |
|
This journal is © The Royal Society of Chemistry 2011 |
Click here to see how this site uses Cookies. View our privacy policy here.