DOI:
10.1039/C0FO00070A
(Paper)
Food Funct., 2011,
2, 130-136
Received
14th July 2010
, Accepted 21st November 2010
First published on 13th December 2010
Abstract
This study aims to determine the function of Plectranthus barbatus (Lamiaceae) herbal tea as inhibitor of the brain acetylcholinesterase (AChE) activity. To accomplish this objective the herbal tea as well as its main component, rosmarinic acid were administered to laboratory animals (rats) and the effect on the brain AChE activity was evaluated. The study of the herbal tea metabolites in the plasma and also in the brain was undertaken. The herbal water extract was administered intragastrically and also intraperitoneally. When the plant extract was intragastrically administered, vestigial amounts of metabolites from P. barbatus extract compounds were present in rat plasma, but none were found in brain, although inhibition of brain acetylcholinesterase activity was detected. However, when P. barbatus extract was administered intraperitoneally, all its compounds were found in plasma, and rosmarinic acid was found in brain. The highest concentrations of compounds/metabolites were found 30 min after administration. An inhibition of 29.0 ± 2.3% and 24.9 ± 3.7% in brain acetylcholinesterase activity was observed 30 and 60 min after intraperitoneal administration, respectively. These values were higher than those expected, taking into account the quantity of rosmarinic acid detected in the brain, which suggests that other active extract compounds or metabolites may be present in non-detectable amounts. These results prove that the administration of P. barbatus aqueous extract can reach the brain and act as AChE inhibitor.
Introduction
Herbal teas may be considered as functional drinks,1 as indeed almost all of the chemical components of these water extracts possess some biological function in the human body. Some of the ethnobotanical uses of these herbs can be explained through the biochemical activities found and described in the literature either for the complete extracts or for the isolated compounds. Leaves of Plectranthus barbatus (Lamiaceae) that are used to prepare herbal teas to treat a wide range of diseases in South America, Africa and world Eastern regions,2 were studied previously concerning the antiacetylcholinesterase as well as the antioxidant activity.3,4 The inhibition of the referred enzyme is the most effective pharmacotherapy for the symptomatic treatment for the Alzheimer disease (AD)5 and the antioxidant activity has been connected with the capacity to scavenge the free radicals that are formed during the inflammation processes.6 The activities found in the P. barbatus herbal tea could be attributed to the main constituent of this tea, rosmarinic acid, together with other compounds, although present in much lesser quantity, abietane diterpenoids and flavonoid glucuronides.3,4 The function of the herbal teas depends on the metabolism that the compounds present in the extract may be subject to during the gastro-intestinal digestion process. The compounds may be transformed into metabolites with different biological activity compared to the one initially determined. In the case of P. barbatus, some of the active compounds found were transformed when the extract was submitted to in vitro conditions simulating the gastrointestinal tract, what caused a small decrease in the biological activity.4 The fact that the herbal tea could pass the digestive tract and keep some of its function lead to an in vivo experiment in order to see if the compounds present in the water extract could reach the brain and still be active there. Recently, for instance, an ethanol extract of Tabernaemontana divaricata proved to be effective in inhibiting neuronal acetylcholinesterase when administered to rats.7
The quantification of the metabolites of the herbal tea components in the blood stream, and the study of the remaining biological activity in the target organ, especially on what concerns the acetylcholinesterase activity, are topics that are seldom referred to in scientific papers. Therefore, the aim of this study was to investigate if the active compounds present in the herbal tea of P. barbatus, or their derivatives, when administered to rats were found in the blood stream and in the brain, and if the neuronal acetylcholinesterase activity was affected by the herbal tea administration.
Experimental
Plant material and extract preparation
Leaves of Plectranthus barbatus Andr. were collected during September of 2008 from plants cultivated in the Botanical Garden of The University of Lisbon. A voucher specimen was deposited in the Herbarium of this Botanical Garden (LISU:214625).
The aqueous plant extract was prepared as a decoction, 10 g of ground fresh leaves boiled for 10 min at 100 °C, in 100 ml of distilled water and filtered through grade 1 Whatman paper. The extract was lyophilised and the yield of extraction was approximately 140 mg of extract per g of plant.
Chemicals
5,5′-Dithiobis[2-nitrobenzoic acid] (DTNB), acetylthiocholine iodide (AChI), dimethylsulfoxide (DMSO), β-glucuronidase type IX-A from E.coli 1
134
600 U g−1, 3
660
000 U g−1 protein, sulfatase from Helix pomatia 14400 U g−1, HEPES buffer, cathecol o-methyltransferase (COMT) from porcine liver, S-adenosylmethionine (SAM), L-cystein non-animal source, rosmarinic acid (RA), quercetin, luteolin and apigenin were obtained from Sigma, St. Louis, USA. Methanol and acetonitrile, both HPLC grade, and trifluoroacetic acid were obtained from Merck, Darmstadt, Germany.
Animals
All experiments were carried out in accordance with the guidelines of the European Communities Council Directive of 24th November 1986 (86/609/ECC). Adult male Sprague–Dawley rats (3–4 months old) were obtained from Instituto de Investigação Científica Bento da Rocha Cabral (Lisbon, Portugal). Two rats per cage were maintained in a room at 22 °C under 12 h dark/light cycling and ad libitum access to water and regular chow.
Preparation of cell-free extracts.
A cell-free liver extract was prepared, essentially as described by Justino and co-workers,8 to obtain the hepatic enzymes that metabolize flavonoids. In brief, rat livers were collected and homogenized in ice-cold 10 mM phosphate buffer, pH 7.4, containing 10 mM 2-mercaptoethanol (1 g wet tissue/2.5 mL buffer). The homogenate was then centrifuged for 90 min at 12
000 g at 4 °C. The protein content of the supernatant was immediately determined by the Lowry method9 and the remainder supernatant was lyophilized and stored at −20 °C until required.
Glucuronidation assay.
The standard assay mixture contained 0.5 mg mL−1P. barbatus extract, or 40 μM rosmarinic acid, or 50 μM flavonoid standards, 2 mM UDPGA, and cell-free extract (5 mg mL−1) in 10 mM potassium phosphate buffer, pH 7.4, in a final volume of 1 mL.8 The reaction was initiated by addition of the cytosolic fraction extracted and the mixture was incubated for 30 min at 37 °C without shaking. The reaction was stopped and the metabolites were extracted as described in the HPLC analysis section below.
Synthesis and identification of methyl rosmarinic acid.
The methylation of rosmarinic acid was done by the porcine liver enzyme catechol-o-methyl-transferase (COMT), using the cofactor S-adenosylmethionine (SAM), with an adaptation of the method described by Baba and co-workers.10 Briefly, 20 U of COMT were dissolved in 200 μl of deoxygenated 10 mM K-phosphate buffer, 20 mM L-cystein, 2 mM MgCl2, pH 7.4 and pre-incubated at 37 °C for 10 min under inert atmosphere. Five hundred microlitres of 7.1 mM SAM and 100 μl of rosmarinic acid, both dissolved in the reaction buffer, were added. Two hundred microlitres of 7.1 mM were added every two hours, and the reaction was stopped at 6 h by the process described in the section for HPLC analysis. The compounds were isolated by HPLC, collected and analyzed by electrospray ionization mass spectrometry (ESI-MS). All experiments were performed using a LCQ Duo ion trap mass spectrometer from Thermo Scientific (San Jose, CA, USA) equipped with an ESI source. Samples were introduced, via a syringe pump (flow rate of 5 μL min−1), into the stainless steel capillary of the ESI source. The applied spray voltage in the source was 4.5 kV, the capillary voltage was 10 V and the capillary temperature was 220 °C. All the mass spectrometer parameters were adjusted in order to optimize the signal-to-noise ratios for the ions of interest. Nitrogen was used as nebulising and auxiliary gas in the source. All mass spectrometry data were acquired in the negative ion mode, the full scan spectra were recorded in the range m/z 100–1000 and three micro-scans were averaged. CID and MS/MS experiments were performed with helium as collision gas.
In vivo studies protocol
Intragastric and intraperitoneal administration.
P. barbatus herbal tea was administered to rats through intragastric procedure (600 mg kg−1, equivalent to 150 μmol RA kg−1) or intraperitoneal injection (1000 mg kg−1, equivalent 250 μmol RA kg−1). For each experiment, twelve adult male Sprague–Dawley rats weighing approximately 400 g and 16 h fasted were randomly divided into two groups. Rosmarinic acid standard was administered dissolved in ethanol saline solution (20% ethanol, 0.9% NaCl solution) in a concentration of 550 μmol kg−1. The high dose administered is justified by the need of obtaining high levels of the different metabolites for analytical purposes. To control animals only the ethanol saline solution was administered. Blood was withdrawn from three rats, thirty minutes and 1 h after administration, by cardiac punction into K3EDTA tubes and kept on ice. The brains were than collected in ice cold K-phosphate buffer 10 mM pH 7.4 10 mM mercaptoethanol and also kept on ice.
Plasma and brain sample preparation.
Plasma was separated from red blood cells by centrifugation at 5000 g for 5 min at 4 °C and samples were stored at −80 °C until further studies.
Each rat brain was weighed and homogenized in 5 ml 10 mM K-phosphate buffer pH 7.4 10 mM mercaptoethanol. The homogenate was then centrifuged for 90 min at 12
000 g, at 4 °C and the supernatant (brain extract) stored at −80 °C until further studies.
Determination of glucuronated and sulfated metabolites.
An adaptation of the method described by Justino and co-workers8 was used. To determine the amounts of glucuronated, sulfated and glucuronated-sulfated compounds sets comprised one assay with β-glucuronidase, one assay with sulfatase, one assay with both enzymes and a control with no addition of enzymes were prepared. To 500 μl of sample were added 1000 U of β-glucuronidase, and/or 25 U sulfatase and incubated at 37 °C for 1 h. The samples were then processed as described in the HPLC analysis section.
HPLC analysis
Methanol was added to 500 μl of plasma or brain extract to 1 ml solution and the mixture was vortexed, left to precipitate for one hour at 4 °C, and centrifuged for 10 min at 10
000 g, at 4 °C. The supernatant was recovered, left to precipitate another hour and centrifuged in the same conditions prior to HPLC analysis. Controls were done using rosmarinic acid and caffeic acid as internal standards added to plasma and brain extracts to determine the losses related to this purification process. This protocol showed negligible loss of the standard compounds (less than 10%) and the yield was taken into account for the concentration calculations.
The HPLC analysis was carried out in Liquid Chromatograph Finnigan™ Surveyor® Plus Modular LC System, Thermo-Finningan, Germany equipped with a LiChroCART® 250-4 LiChrospher® 100 RP-18 (5 μm) column, from Merck, Darmstadt, Germany, and Xcalibur software. The extracts were analysed by HPLC injecting 25 μl with an auto injector, and using a linear gradient composed of solution A (0.05% trifluoroacetic acid), solution B (acetonitrile) and solution C (methanol) as following: 0 min, 70% A, 5% B, 20% C; 20 min 10% A, 10% B, 80% C; 25 min, 10% A, 10% B, 80% C. The detection was carried out between 200 and 600 nm with a diode array detector.
Acetylcholinesterase enzymatic activity was measured using an adaptation of the method described by Chattipakorn and co-workers.7 Four hundred microlitres of 50 mM HEPES buffer pH 8 and 50 μl brain extract were mixed in spectrophotometer cuvette and left to incubate for 15 min at 25 °C. Subsequently, 75 μl of a solution of AChI (0.023 mg ml−1) and 475 μl of 3 mM DTNB in Hepes 50 mM pH 8 were added. The absorbance at 405 nm was read during the first five minutes of the reaction and the initial velocity was calculated as μAU min−1 mg−1 and converted into nmole min−1 mg−1 of tissue mass. A control reaction was carried out using the brain extract from the control rats, and it was considered 100% activity for calculations of enzymatic inhibition.
Statistical analysis
All results are presented as mean ± standard deviation and the software used was Microsoft ® Excel 2007. Additionally analysis of variance was performed with p = 0.05.
Results and discussion
P. barbatus herbal tea proved to have antiacetylcholinesterase activity in previous studies, with an IC50 of 1.02 ± 0.02 mg of dry leaves ml−1 and this activity was kept constant after in vitro gastric digestion and lost approximately 50% after the in vitro pancreatic studies.3,4 Rosmarinic acid, luteoline 7-O-glucuronide, apigenine 7-O-glucuronide, two abietane diterpenoid, acacetin 7-O-glucuronide and (16S)-coleon E, were the compounds identified in the water extract,4 being rosmarinic acid the main component. Although all the compounds demonstrated inhibition activity relatively to AChE, an IC50 of 0.44 mg ml−1 for the main component was determined.3 Inhibition studies demonstrated that the process was reversible (unpublished studies). Due to these previous results, the study was continued by analysing the action of P. barbatus herbal tea in vivo. In the present work the metabolism of the herbal tea after intragastric and intraperitoneal administration to laboratory animals was analysed.
Intragastric administration of P. barbatus extract
Plasma.
After intragastric administration of P. barbatus extract, the plasma was analysed by HPLC and rosmarinic acid was the only compound detected. In order to confirm if this was indeed the only compound present in the plasma or if some metabolites could be present but in a low amount that was beneath the detection limit of the system, β-glucuronidase and sulfatase were added and allowed to react under the conditions described in the Experimental section. The plasma was analysed once again by HPLC, and this time the rosmarinic acid showed an increase in its area and the aglycons from the flavonoid derivatives were also detected. The results from this study confirmed that not only the presence of rosmarinic acid glucoronide and sulfo-derivatives, but also the presence of the flavonoid glucoronide derivatives in the plasma. The concentration of these rosmarinic acid metabolites found in the plasma are shown in Table 1. It can be seen that the derivatives of rosmarinic acid in circulation in the blood stream decrease after 30 min. This study indicated that, in fact, the flavonoid derivatives found initially in the herbal tea could pass through the gastro-intestinal barrier and appear in the plasma (Table 1). A vestigial peak with retention time corresponding to acacetin was also found. The quantity of total rosmarinic acid found in the plasma relatively to the amount of rosmarinic acid administered in the extract can be calculated, assuming that a male Sprague-Dawley rat contains 4.12 ml plasma per 100 g body weight,11 as 0.009% and 0.005% for 30 and 60 min, respectively.
Table 1 Concentration of rosmarinic acid, its metabolites and flavonoid glucuronide derivatives in the plasma and in the brain, 30 and 60 min after the intragastric and intraperitoneal administration of P. barbatus extract
|
Compound |
Concentration in plasma |
Concentration in the brain |
Intragastric administration/nM |
Intraperitoneal administration/μM |
Intraperitoneal administration/μM |
30 min |
60 min |
30 min |
60 min |
30 min |
60 min |
P. barbatus extract |
Rosmarinic acid (RA) |
<0.5 |
10.1 |
1440 ± 144 |
745 ± 282 |
24.1 ± 1.1 |
20.4 ± 0.4 |
RA glucuronides |
112.1 |
87.8 |
— |
— |
— |
— |
RA sulfates |
210.4 |
77.3 |
— |
— |
— |
— |
RA methyl |
— |
— |
17.8 ± 2.3 |
11.1 ± 3.6 |
— |
— |
Luteolin glucuronide |
1.9 |
— |
40.5 ± 5.9 |
29.9 ± 8.0 |
— |
— |
Apigenin glucuronide |
6.5 |
— |
20.0 ± 3.4 |
3.7 ± 0.3 |
— |
— |
Rosmarinic acid standard |
RA |
4.9 × 103 |
1.9 × 103 |
1042.3 ± 192.1 |
— |
25.4 ± 0.9 |
— |
RA methyl |
— |
— |
16.7 ± 5.6 |
— |
— |
— |
Rosmarinic acid was also intragastrically administered, in a higher quantity than that found in the herbal extract, and analysed under the same conditions. Rosmarinic acid was present in the plasma 30 min and 60 min after the intragastric administration. The quantities found (Table 1) corresponded to 0.036% and 0.015% of the amount of rosmarinic acid administered to rats, 30 and 60 min after administration, respectively. The results one hour after administration showed a decrease in the rosmarinic acid concentration in circulation. The test with β-glucuronidase was also carried out, but an increase in the rosmarinic acid area in the HPLC chromatogram was not detected. This means that there were no glucoronated metabolites of this phenolic acid formed in the plasma. It seems that rosmarinic acid, as pure compound or inside the herbal tea, behaves differently.
Brain AChE.
When rat brains were analysed by HPLC no compounds or metabolites of P. barbatus extract or rosmarinic acid were found. However, when the brain acetylcholinesterase activity was measured, a decrease of 10.0 ± 1.8% and 5.5 ± 1.7% of the enzymatic activity was observed, 30 and 60 min after the extract administration, respectively (Table 2), from the control value of activity of 0.149 ± 0.002 nmol min−1 mg−1. In the case of rosmarinic acid injection a 13.5% decrease in AChE activity was detected after 30 min.
Table 2 Brain acetylcholinesterase inhibition (%) 30 and 60 min after administration (intragastric and intraperitoneal) of rosmarinic acid and P. barbatus extract. Results significantly different from the control are marked with * (P < 0.05) and ** (P < 0.1). Values that are not significantly different (P < 0.05) are marked from a to d
|
|
Brain acetylcholinesterase inhibition (%) |
Intragastric administration |
Intraperitoneal administration |
P. barbatus
|
30 min |
10.0 ± 1.8*a |
29.0 ± 2.3*c |
|
60 min |
5.5 ± 1.7**b |
24.9 ± 3.7*d |
Rosmarinic acid |
30 min |
13.5 ± 1.7*a |
10.7 ± 5.0**ab |
|
60 min |
12.8 ± 2.4*a |
— |
When standard rosmarinic acid was administered intragastrically the concentration of rosmarinic acid found in blood showed values in the same magnitude of those found in other studies.10,12 However, in the present work, when rosmarinic acid was present within the extract, a lower quantity of this acid was found in plasma, suggesting that the other extract components may interfere in the gut permeation of rosmarinic acid. Previous pharmacokinetic studies also reported that the highest concentration of rosmarinic acid was reached in less than 30 min after intragastric administration.10,12 The present study also observed a decrease in the rosmarinic acid concentration after 30 min. The methylated, sulfated and hydrolysed forms of rosmarinic acid into simpler hydroxycinnamic acids forms were observed.10,12,13 In the present work, the methylated form of rosmarinic acid was not detected. This suggests that when rosmarinic acid is administered alone, it can pass the intestinal barrier without undergoing metabolization by the intestinal cells, but it can be glucoronated and sulfated as confirmed by the action of β-glucuronidase and sulfatase.
Taking into account that the amount of rosmarinic acid in the extract was approximately one third of the rosmarinic acid standard, it is seen that the decrease in the enzyme activity is not linearly correlated with the rosmarinic acid concentration. This is due to the fact that the extract contains other compounds besides rosmarinic acid. These compounds also inhibit acetylcholinesterase4 and the present study indicates that they can also reach the brain and act together with rosmarinic acid as enzyme inhibitors. This fact is corroborated by the analysis of the rosmarinic acid concentration in the plasma (less than 0.5 nM when the herbal tea is administered and 4.9 μM when the standard is given to the laboratory animals) and the enzyme inhibitory activity, which is similar in both situations. These results can only be explained with the inhibitory activity of all the compounds, including the rosmarinic acid derivatives, towards AChE.
Intraperitoneal administration of P. barbatus extract
Plasma.
After intraperitoneal administration of P. barbatus aqueous extract, rat plasma was analysed by HPLC, Fig. 1a. With the exception of the diterpenoid 6, all the extract compounds were present in rat plasma in higher amount at 30 min than 60 min after injection (Fig. 1a). The abietane diterpenoid 4 has a structure similar to the abietane diterpenoid 6, and is present in the extract as a minor compound. Previous studies reported that it may be formed from abietane diterpenoid 6 in the small intestine conditions,4 this suggests that abietane diterpenoid 6 might have been completely transformed into abietane diterpenoid 4 under the biological blood-circulation conditions.
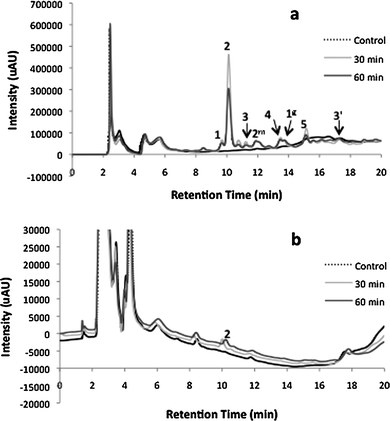 |
| Fig. 1 HPLC analysis, 30 and 60 min after intraperitoneal administration of P. barbatus extract, of (a) plasma and (b) brain. 1: luteolin 7-O-glucuronide (retention time 9.6 min); 2: rosmarinic acid (RT: 10.4); 3: apigenin 7-O-glucuronide (RT: 11.2); 4: abietane diterpenoid (RT: 13.8); 5: acacetin 7-O-glucuronide (RT: 15.1); 2m: monomethylated rosmarinic acid; 1g: luteolin glucuronide derivative; 3′: apigenin. | |
Rosmarinic acid, compound 2, is the major compound found in the chromatogram, Fig. 1a. The amount of rosmarinic acid present in the plasma was calculated to be 1439.8 ± 144.0 μM and 744.5 ± 282.3 μM for 30 and 60 min after the extract administration, respectively, Table 1. The amount of rosmarinic acid found in the plasma corresponded to 23.7 ± 2.4% and 12.3 ± 4.3% of the administered amount. In the chromatogram, a peak of methylated rosmarinic acid, 2m, could be detected. According to a standard previously developed by using a commercial COMT from porcine liver and SAM as cofactor in a methodology described in the Experimental section, it was possible to prove the formation of a methylated derivative from rosmarinic acid. The amount of methyl rosmarinic acid was 17.8 ± 2.3 μM and 11.1 ± 3.6 μM for the same administration time-points, Table 1. The search for glucuronated/sulfated metabolites was carried out by reacting β-glucuronidase and sulfatase with the plasma. The only increase in area was observed for flavonoid aglycons, corresponding to the decrease in the corresponding of area of the respective glucuronide peaks. The fact that no significant amounts of glucuronated/sulfated rosmarinic acid metabolites were found after intraperitoneal administration could be explained by the predominance of methylation occurring in the liver over other metabolization reactions, such as sulfation and glucuronidation. This situation was also reported by O'Leary and co-workers.14
In the chromatogram shown in Fig. 1a, a different glucoronide of luteolin than the one initially present in the water extract (compound numbered 1g) could be detected. Compound 1g was identified after preparing, in vitro, the glucoronidation derivatives according to Experimental section. In this chromatogram the aglycon apigenin (compound numbered 3′) could also be seen. No luteolin or acacetin aglycons were detected in the plasma samples. These results suggest that the deglucuronation of plasma apigenin glucuronide may be the main reaction occurring to this compound. Studies concerning the oral administration of luteolin suggested that the presence of the unconjugated luteolin in plasma might be due to the permeation of the aglycon without undergoing conjugation by the intestinal cells.15 However, in the present study apigenin was already administrated in its glucuronated form, and so the high amount of aglycon found in plasma must be due to deglucuronation of the apigenine glucuronide. The deglucuronidation of flavonoid glucuronides by the liver was previously reported by O'Leary and co-workers,14 who remarked that the conversion of the quercetin-7- and quercetin-3-glucuronide to the mono-sulfate conjugate showed an intermediate step of deglucuronidation by β-glucuronidase activity, allowing transient contact of the free aglycon with the cellular environment. The detection only of the apigenin aglycon in plasma may be due to a higher deglucuronidation of the apigenine glucuronide, comparatively to the other flavonoids and/or to a lower re-glucuronidation of apigenin by the liver. The in vitro glucuronidation assay showed a lower rate of glucuronidation for apigenin than for luteolin, which may explain the existence of apigenin aglycon relatively to luteolin. This hypothesis is also supported by the appearance of a luteolin glucuronide, that could only be produced by deglucuronidation of the extract's luteoline 7-O-glucuronide, followed by glucuronidation in a different position (Fig. 1a). Little is known about these de-conjugation and re-conjugation reactions in flavonoids, especially when they are administered in their glucuronated forms. The present study differs from the majority of the in vivo flavonoid administration reports in the fact that the several flavonoids were simultaneously administered as glucuronide derivatives, as they are commonly found in herbal teas, instead of their aglycons.
All extract compounds showed a decrease from 30 to 60 min after extract administration, with rates of elimination ranging from 25% to 85% (Table 3), the lowest value for the luteolin glucuronide with retention time 13.8 min, numbered 1g (24.4 ± 6.9%), and the highest value for the apigenin glucuronide, numbered 3 (84.5% ± 4.6%). Only the aglycon from apigenin (retention time of 17.3 min, numbered 3′) (Fig. 1a) was seen. No luteolin or acacetin aglycons were detected in the plasma.
Table 3 Retention time of compounds from the P. barbatus extract found in plasma and their decrease from 30 to 60 min after the extract intraperitoneal administration
|
Compound |
Retention time/min |
Decrease from 30 to 60 min (%) |
Luteolin 7-O-glucuronide |
(1) |
9.7 |
44.91 ± 13.48 |
Rosmarinic acid |
(2) |
10.5 |
51.66 ± 15.60 |
Apigenin 7-O-glucuronide |
(3) |
11.3 |
84.57 ± 4.59 |
Monomethylated rosmarinic acid |
(2m) |
12.0 |
41.7 ± 14.5 |
Abietane diterpenoid |
(4) |
13.5 |
36.33 ± 15.61 |
Luteolin glucuronide |
(1g) |
13.8 |
24.36 ± 6.88 |
Acacetin 7-O-glucuronide |
(5) |
15.2 |
56.75 ± 0.10 |
Apigenin |
(3′) |
17.3 |
33.4 ± 12.8 |
Brain AChE.
After intraperitoneal administration of P. barbatus extract, brains from rats were also analysed after 30 and 60 min by HPLC, Fig. 1b. The chromatograms showed only the presence of unconjugated rosmarinic acid, the other extract compounds or their metabolites were not detected (Fig. 1b). No increase of the rosmarinic acid peak area or the aglycons from the flavonoid derivatives were observed after the reactions with β-glucuronidase or sulfatase. The amount of rosmarinic acid in rat brains was quantified and a relationship with the amount of rosmarinic acid in the plasma was calculated (Table 1). The amount of rosmarinic acid found in the brain was 0.40 ± 0.02% and 0.34 ± 0.01% of the administered amount, for 30 and 60 min after administration, respectively. Although the plasma rosmarinic acid concentration showed a decrease from 30 to 60 min (around 52%, Table 3) the brain rosmarinic acid concentration decreased around 15% (14.90 ± 7.11%, Table 1). This difference is reflected in the ratio of brain/plasma rosmarinic acid concentration, which is higher 60 min after the extract administration than at 30 min (0.027 at 60 min and 0.016 at 30 min). The brain/plasma concentration ratio reflects the permeability of the compounds from the plasma to the brain, which may be restricted primarily by the blood brain barrier, and ranges from 0 (no permeability) to 1 (total permeability).16 These ratios indicate that rosmarinic acid has the ability to pass to the brain, but the permeability is low. Rosmarinic acid diffuses slowly to the brain, but it is retained there longer than in the plasma, where a higher decrease was observed from 30 to 60 min. Brains of rats to which standard rosmarinic acid was administered, (results not shown) showed the same concentration of rosmarinic acid as when rosmarinic acid was given within the extract, leading to the conclusion that the other extract compounds do not interfere with the permeability of rosmarinic acid in the blood-brain barrier, in opposition to the gut barrier where a interference of the other extract compounds was observed in the permeability of rosmarinic acid.
Thirty minutes after the extract administration the rat brain showed an inhibition in acetylcholinesterase activity of 29.0 ± 2.3%, and after 60 min an inhibition of 24.9 ± 3.7% (Table 2), in comparison with the control rats, where the activity was 0.145 ± 0.012 nmol min−1 mg−1. Brains of rats where standard rosmarinic acid was administered showed the same concentration of rosmarinic acid as that detected when the extract was administered, but demonstrated to have less acetylcholinesterase inhibition (10.7 ± 5.0% after 30 min). This confirms the inhibition of AChE by the other extract components, although they were not detected by HPLC.
The percentages of acetylcholinesterase inhibition achieved were of the same magnitude of the ones reported to galanthamine in the rat model, however the amounts of interaperitoneal injection of galanthamine were lower: 3 mg kg−1 for 10% inhibition17 and 10 mg kg−1 for 28% inhibition.7 Higher inhibitions can be obtained with lower amounts of donepezil, 39% with 3 mg kg−1,17 however this inhibitor may present a higher incidence of adverse effects in high amounts. The dosage/activity in humans for galanthamine is 16–24 mg day−1 to 30–40% brain acetylcholinesterase inhibition,18 and for donepezil is 10 mg day−1 to 19–27% inhibition.19 As there is an interest in decreasing the adverse peripheral effects of acetylcholinesterase inhibition, mostly related to gastrointestinal and hepatic disturbances, herbal teas may offer an alternative for mild treatments of Alzheimer's disease. P. barbatus water extracts are traditionally prepared as decoctions (as in the present report) and taken by people and those secondary effects were not reported.2 In fact, the use of this plant extract to treat gastrointestinal and hepatic conditions is widely reported.2 Rosmarinic acid when administered in low doses seems to act as an axiolytic-like compound, only when administered in high doses it seem to act on the peripheral nervous system.20
Several recent reports showed that flavonoids, such as quercetin and its metabolites, have the ability to reach the brain.21 Coleta and co-workers22 demonstrated that intragastrically-administered luteolin, or its metabolites, should reach the brain, causing anxiolytic-like effects. Gujinski and co-workers23 reported that ethanolic extracts from Melissa officinalis, a Lamiaceae species, and its main component rosmarinic acid, when administered to rats produced dose-related antinociception in several models of chemical pain, through mechanisms that involved cholinergic systems. Other studies also show that rosmarinic acid, when administered through intraperitoneal injection (2–8 mg kg−1), induced neurobehavioral changes in rats.20 Although rosmarinic acid in the brain was not quantified in those studies, its presence in the brain was suggested by the activities it exerted in vivo.
The bioavailability of the currently used acetylcholinesterase inhibitors is highly variable, depending mostly to the structure of the compounds, their metabolization and elimination, and their ability to permeate the intestinal and blood-brain barriers.24 Their time of action also depends on the mode of acetylcholinesterase inhibition. Donepezil, for instance, has a peak plasma level 4 h after administration and a half-life of over 70 h, while tacrine shows low bioavailability when taken orally and has a half-life in plasma of 1.4 h.24 However, tacrine reaches a brain concentration that is 10-fold that of plasma, its dosage and brain bioavailability are not proportional, and so higher doses and multiple dosing are used to increase bioavailability and the half-life.24
In the present case rosmarinic acid and the other components of the extract also do not seem to follow a direct relation between dose and response. First, because the intestinal absorption and metabolization of rosmarinic acid is influenced by the presence of the other extract compounds. Also, although the concentration of rosmarinic acid was always much lower in brain than in the plasma, the relationship between plasma and brain rosmarinic acid concentrations does not seem to be linear, as a decrease of more than 50% in plasma rosmarinic acid concentration corresponded just to a slight decrease in the brain (Table1). However, the amount of detected rosmarinic acid and the activity seem to be related, as the decrease in the brain acethylcholinesterase inhibition was similar to the decrease in the content in rosmarinic acid. As the phenolic compounds from the plant extract seem to act in the brain in very low concentrations, and their amount in brain has less fluctuations than the amount in the plasma, taking a large volume of herbal tea during a long amount of time, and several times per day, as it is usually taken, may be the correct way of increasing its effects.
Apart from the beneficial effect of increasing the acetylcholine-mediated neuronal tansmission, the treatment of Alzheimer's disease with acetylcholinesterase inhibitors also seems to protect free radical toxicity, β-amyloid injury and to attenuate cytokine release from microglial cells.25 Apart from this “cholinergic anti-inflamatory pathway”, rosmarinic acid is also a well-known antioxidant and free radical scavenger.3
As far as we know, this is the first report confirming the neurologic activity of rosmarinic acid by the quantification its amount in the brain.
Conclusion
In this work it was demonstrated that the rosmarinic acid, present in herbal teas, may, in fact, cross the intestinal barrier, as well as the blood brain barrier, and be detected in the brain. There, it inhibits the enzyme AChE. The metabolization and bioavailability of the herbal tea components is different from the administration of pure compounds.
Acknowledgements
The authors thank Fundação para a Ciência e Tecnologia, FCT, for financial support (REDE/1501/REM/2005). Pedro L. V. Falé and Paulo J. A. Madeira thank FCT for the PhD Grant SFRH/BD/37547/2007 and SFRH/BD/27614/2006, respectively.
References
-
S. S. Percival, R. E. Turner. Applications of Herbs to Functional Foods. In: Handbook of Nutraceuticals and Functional Foods, 2nd Ed. (Edited by R. E. C. Wildman), CRC Press, 2007, pp 269–284 Search PubMed.
- C. W. Lukhoba, M. S. J. Simmond and A. J. Paton, Plectranthus: A review of ethnobotanical uses, J. Ethnopharmacol., 2006, 103, 1–24 CrossRef.
- P. L. Falé, C. Borges, P. J. A. Madeira, L. Ascensão, M. E. M. Araújo, M. H. Florêncio and M. L. M. Serralheiro, Rosmarinic acid, scutellarein 4′-methyl ether 7-O-glucuronide and (16S)-coleon E are the main compounds responsible for the antiacetylcholinesterase and antioxidant activity in herbal tea of Plectranthus barbatus (“falso boldo”), Food Chem., 2009, 114, 798–805 CrossRef CAS.
- S. Porfirio, P. L. V. Falé, P. J. A. Madeira, H. Florêncio, L. Ascensão and M. L. M. Serralheiro, Antiacetylcholinesterase and antioxidant activities of Plectranthus barbatus tea, after in vitro gastrointestinal metabolism, Food Chem., 2010, 122, 798–805.
- M. Heinrich and H. L. Teoh, Galanthamine from snowdrop – the development of a modern drug against Alzheimer's disease from local Caucasian knowledge, J. Ethnopharmacol., 2004, 92, 147–162 CrossRef CAS.
- A. Gomes, E. Fernandes, J. F. C. Lima, L. Mira and M. L. Corvo, Molecular mechanisms of anti-inflammatory activity mediated flavonoids, Curr. Med. Chem., 2008, 15, 1586–1605 CrossRef CAS.
- S. Chattipakorn, A. Pongpanparadorn, W. Pratchayasakul, A. Pongchaidacha, K. Ingkaninan and N. Chattipakorn, Tabernaemontana divaricata extract inhibits neuronal acetylcholinesterase activity in rats, J. Ethnopharmacol., 2007, 110, 61–68 CrossRef.
- G. C. Justino, M. R. Santos, S. Canário, C. Borges, M. H. Florêncio and L. Mira, Plasma quercetin metabolites: structure–antioxidant activity relationships, Arch. Biochem. Biophys., 2004, 432, 109–121 CAS.
- O. H. Lowry, N. J. Rosebrough, A. L. Farr and R. J. Randall, Protein measurement with the Folin phenol reagent, J. Biol. Chem., 1951, 193, 265–275 CAS.
- S. Baba, N. Osakabe, M. Natsume and J. Terao, Orally administered rosmarinic acid is present as the conjugated and/or methylated forms in plasma, and is degraded and metabolized to conjugated forms of caffeic acid, ferulic acid and m-coumaric acid, Life Sci., 2004, 75, 165–178 CrossRef CAS.
- R. J. Probst, J. M. Lim, D. N. Bird, G. L. Pole, A. K. Sato and J. R. Claybaugh, Gender differences in the blood volume of conscious Sprague-Dawley rats, J. Am. Assoc. Lab. Anim. Sci., 2006, 45, 49–52 Search PubMed.
- Y. Konishi, Y. Hitomi, M. Yoshida and E. Yoshioka, Pharmacokinetic Study of Caffeic and Rosmarinic Acids in Rats after Oral Administration, J. Agric. Food Chem., 2005, 53, 4740–4746 CrossRef CAS.
- T. Nakazawa and K. Ohsawa, Metabolism of rosmarinic acid in rats, J. Nat. Prod., 1998, 61, 993–996 CrossRef CAS.
- K. A. O'Leary, A. J. Day, P. W. Needs, F. A. Mellon, N. M. O'Brien and G. Williamson, Metabolism of quercetin-7- and quercetin-3-glucuronides by an in vitro hepatic model: the role of human β-glucuronidase, sulfotransferase, catechol-O-methyltransferase and multi-resistant protein 2 (MRP2) in flavonoid metabolism, Biochem. Pharmacol., 2003, 65, 479–491 CrossRef CAS.
- K. Shimoi, H. Okada, M. Furugori, T. Goda, S. Takase, M. Suzuki, Y. Hara, H. Yamamoto and N. Kinae, Intestinal absorption of luteolin and luteolin 7-O-beta-glucoside in rats and humans, FEBS Lett., 1998, 438, 220–224 CrossRef CAS.
- G. Z. Zheng, P. Bhatia, T. Kolasa, M. Patel, O. F. El Kouhen, R. Chang, M. E. Uchic, L. Miller, S. Baker, S. G. Lehto, P. Honore, J. M. Wetter, K. C. Marsh, R. B. Moreland, J. D. Brioni and A. O. Stewart, Correlation between brain/plasma ratios and efficacy in neuropathic pain models of selective metabotropic glutamate receptor 1 antagonists, Bioorg. Med. Chem. Lett., 2006, 16, 4936–4940 CrossRef CAS.
- H. Geerts, P.-O. Guillaumat, C. Grantham, W. Bode, K. Anciaux and S. Sachak, Brain levels and acetylcholinesterase inhibition with galantamine and donepezil in rats, mice, and rabbits, Brain Res., 2005, 1033, 186–193 CrossRef CAS.
- A. Kadir, T. Darreh-Shori, O. Almkvist, A. Wall, M. Grut, B. Strandberg, A. Ringheim, B. Eriksson, G. Blomquist, B. Långström and A. Nordberg, PET imaging of the in vivo brain acetylcholinesterase activity and nicotine binding in galantamine-treated patients with AD, Neurobiol. Aging, 2008, 29, 1204–1217 CrossRef CAS.
- N. I. Bohnen, D. I. Kaufer, R. Hendrickson, L. S. Ivanco, B. J. Lopresti, R. A. Koeppe, C. C. Meltzer, G. Constantine, J. G. Davis, C. A. Mathis, S. T. DeKosky and R. Y. Moore, Degree of inhibition of cortical acetylcholinesterase activity and cognitive effects by donepezil treatment in Alzheimer's disease, J. Neurol., Neurosurg. Psychiatry, 2005, 76, 315–319 CrossRef CAS.
- P. Pereira, D. Tysca, P. Oliveira, L. F. S. Brum, J. N. Picada and P. Ardenghi, Neurobehavioral and genotoxic aspects of rosmarinic acid, Pharmacol. Res., 2005, 52, 199–203 CrossRef CAS.
- P. Huebbe, A. E. Wagner, C. Boesch-Saadatmandi, F. Sellmer, S. Wolffram and G. Rimbach, Effect of dietary quercetin on brain quercetin levels and the expression of antioxidant and Alzheimer's disease relevant genes in mice, Pharmacol. Res., 2010, 61, 242–246 CrossRef CAS.
- M. Coleta, M. G. Camposa, M. D. Cotrim, M. C. T. Lima and A. P. Cunha, Assessment of luteolin (3_,4_,5,7-tetrahydroxyflavone) neuropharmacological activity, Behav. Brain Res., 2008, 189, 75–82 CrossRef CAS.
- G. Guginski, A. P. Luiz, M. D. Silva, M. Massaro, D. F. Martins, J. Chaves, R. D. Mattos, V. M. Ferreira, J. B. Calixto and A. R. Santos, Mechanisms involved in the antinociception caused by ethanolic extract obtained from the leaves of Melissa officinalis (lemon balm) in mice, Pharmacol., Biochem. Behav., 2009, 93, 10–16 CrossRef CAS.
- B. M. McGleenon, K. B. Dynan and A. P. Passmore, Acetylcholinesterase inhibitors in Alzheimer's disease, Br. J. Clin. Pharmacol., 2001, 48, 471–480 CrossRef.
- N. Tabet, Acetylcholinesterase inhibitors for Alzheimer's disease: anti-inflammatories in acetylcholine clothing!, Age Ageing, 2006, 35, 336–338 CrossRef CAS.
|
This journal is © The Royal Society of Chemistry 2011 |
Click here to see how this site uses Cookies. View our privacy policy here.