DOI:
10.1039/C0FO00016G
(Review Article)
Food Funct., 2011,
2, 18-27
Bioactive peptides derived from milk proteins and their health beneficial potentials: an update
Received
19th May 2010
, Accepted 21st September 2010
First published on 7th December 2010
Abstract
It has been well recognized that dietary proteins provide a rich source of biologically active peptides. Today, milk proteins are considered the most important source of bioactive peptides and an increasing number of bioactive peptides have been identified in milk protein hydrolysates and fermented dairy products. Bioactive peptides derived from milk proteins offer a promising approach for the promotion of health by means of a tailored diet and provide interesting opportunities to the dairy industry for expansion of its field of operation. The potential health benefits of milk protein-derived peptides have been a subject of growing commercial interest in the context of health-promoting functional foods. Hence, these peptides are being incorporated in the form of ingredients in functional and novel foods, dietary supplements and even pharmaceuticals with the purpose of delivering specific health benefits.
1. Introduction
The major role of milk proteins is to supply amino acids and nitrogen to young mammals and constitute an important part of dietary proteins for the adult. Intact milk proteins also have specific functions such as micelle formation. Furthermore, milk proteins have physiological importance, they facilitate uptake of several important nutrients such as trace elements and vitamins and contain a group of proteins which perform a protective function, therefore indicating their importance as a multi-functional substances. Milk borne bioactive peptides have various beneficial health activities, and few of them are listed in Fig. 1. During the last two decades, it has become clearer that milk proteins are a source of biologically active peptides.1,2 These peptides are inactive within the sequence of parent protein, and can be released during gastrointestinal digestion or food processing. Once bioactive peptides are liberated, they may act as regulatory compounds with hormone-like activity. Since 1979, several research groups have studied numerous peptides exhibiting various activities such as opiate, anti-thrombotic or anti-hypertension activity, immuno-modulation or mineral utilization properties.3,4 Milk proteins are the most important source of bioactive peptides, though other animal as well as plant proteins also contain potential bioactive sequences.3,5,6 The first biologically active peptide found in milk was opioid peptide followed by immuno-modulating peptide.7
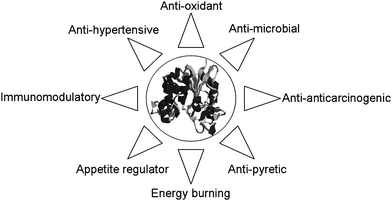 |
| Fig. 1 The health beneficial bioactivites of milk borne bioactive peptides. | |
The investigation techniques have included the following: the establishment of an assay system of biological activities, hydrolysis of proteins by digestive enzymes, isolation of peptides, determination of structures and synthesis of peptides. Investigation strategies have also comprised synthesis of peptides within milk proteins, based on sequence similarities of peptides having known biological activity. Most of the studies have so far been in vitro. The physiological functions of these peptides remain to be established in vivo.
In the present paper some aspects concerning biologically functional milk derived peptides, biochemical and physiological properties as well as possible dietary application of peptides will be discussed.
2. Peptides with opioid activity
Opioid peptides are defined as peptides like enkephalins that have both affinity for opiate receptor and opiate-like effects which are inhibited by naloxone. The typical opioid peptides all originate from three precursor proteins- proopiomelanocortin (endorphins), proenkephalin (enkephalin) and prodynorphin (dynorphins).8 All of these typical opioid peptides have the same N-terminal sequence, Tyr-Gly-Gly-Phe. Opioid peptides exert their activity by binding to specific receptors of the target cell. Individual receptors are responsible for specific physiological effects, e.g., the μ-receptor for emotional behavior and suppression of intestinal motility, the σ-receptor for emotional behavior, and the κ-receptor for sedation and food intake. The opioid peptides derived from a variety of precursor proteins are called “atypical” opioid peptides, since they carry various amino acid sequences at their N-terminal regions, only the N-terminal tyrosine is conserved. The N-terminal sequence of the atypical opioid peptides is Tyr-X-Phe or Tyr-X1-X2-Phe. The tyrosine residue at the N-terminal and the presence of another aromatic amino acid at third or fourth position is an important structural motif that fits into the binding site of the opioid receptors.
The first studied food derived opioid peptides were the β-casomorphins.9 Opioid properties have been demonstrated for β-casein f60–70 as well as for fragments thereof. Morphiceptin, which is an amide derivative of β-casomorphin-4, is a highly specific opioid agonist for both μ receptors in guinea pig ileum and morphine binding sites in rat brain. Other β-casomorphins has also been characterized as μ-type ligands.10 β-casomorphins were also found in analogous positions in sheep, water buffalo and human casein.11 Other milk opioid agonist peptides are α-casein derived exorphins, corresponding to bovine αs1-casein f90–96.12 These α-casein exorphins are -selective receptor ligands and can be separated from pepsin hydrolysate of α-casein.13,14 All bovine κ-casein fragments having opioid activities - referred to as casoxins (κ-casein f33–39, f25–34) as well as lactoferrin fragments termed lactoferroxin – behave as opioid antagonists. The antagonistic activity of casoxin (κ-casein f33–38) was lower than that of naloxone, and it can be regarded as a μ- and κ-selective receptor with low affinity.15
Opioid peptides can be obtained by in vitro enzymatic hydrolysis of bovine caseins.16 Precursors of β-casomorphins have also been identified in Parmesan-cheese.17 β-casomorphins have been detected in the duodenal chyme of minipigs18 and in the human small intestine19 as a consequence of in vivo digestion.
Whey proteins contain opioid-like sequences in their primary structure, namely α-lactalbumin (both bovine and human) f50–53 and β-lactoglobulin (bovine) f102–105. These peptides were termed as α- and β-lactorphins.15,20 Bovine blood serum albumin f399–404 - serorphins - also exhibit opioid activity.21 α-, and β-lactorphin can be released from parent protein using in vitro proteolysis by various proteolytic enzymes.22,23 α-lactorphin exerts a weak but consistent opioid property (Table 1) in the smooth muscle and receptor binding, while β-lactorphin, in spite of the similar receptor binding affinity, exerts an apparently non-opioid stimulatory effect on the guinea pig ileum. α-, and β-lactorphins were μ-type receptor ligands.24
Table 1 Examples of biologically functional peptides derived from bovine whey proteins
There are different possible intestinal and peripheral target sites of the active substance. As opioid receptor ligands, these peptides can be expected to behave like other opioids i.e. to act as agonist or antagonist, to bind to receptors and elicit effects at all cells or tissues where opioids are known to do this, mediated via signal transduction pathways as already known for opioids. Indirect evidence have suggested the presence of β-casomorphins in the intestinal of humans after milk ingestion,19 whereas milk derived opioid peptides do not seem to permeate into the cardiovascular compartment, in more than negligible amounts, in adult mammals. Enzymatic degradation of peptides in the intestinal wall and in the blood appears to prevent gastrointestinal malfunction.25 It seems likely that casomorphins participate in the control of gastrointestinal function in adults.26 Casomorphins have been found to prolong gastrointestinal transit time and exert antidiarrhoeal action.27 The physiological significance of opioid peptides may be different in pregnant, puerperal women and in neonates. β-casomorphin immunoreactive materials, obviously representing β-casein cleavage products larger than β-casomorphins, i.e. potential β-casomorphin precursors, have been found in plasma and in the cardiovascular compartment in women during pregnancy and lactation, and new-born animals.28 These findings indicate the pharmacological activity of β-casomorphins not for physiological significance.
3. Peptides with antihypertensive properties
Angiotensin-converting enzyme (ACE) is a multifunctional enzyme, located in different tissues throughout the body. It plays an important physiological role in the regulation of local levels of several endogenous bioactive peptides.29 Blood pressure is regulated by renin-angiotensin system and bradykinin system (Fig. 2).
ACE converts Angiotensin I to a potent vaso constrictor, angiotensin II, through the removal of two aminoacids from terminal of the precursor, consequently raising the blood pressure. Inhibition of ACE activity reduces angiotensin II production and lowers blood pressure in hypertensive patients.30 The Brady Kinin system ACE prevents the degradation of vasodialator, thus helping in controlling blood pressure.
The first point of action of ACE inhibitory peptide would be in brush border membrane bound ACE. It is feasible that smaller ACE inhibitory peptides would move across the gut epithelium and into the circulation to reach other sites within the body. C-terminal tri peptide sequence of substrate strongly influences binding of these peptides to ACE.31,32 Hydrophobic residues tryptophan, tyrosine, phenyl alanine and proline were found to be most effective.33 Two potent ACE inhibitory tri-peptides val-pro-pro and Ile-pro-pro from bovine casein was isolated from Calpis (Japanese sour fermented milk) with a starter L. helveticus and Saccharomyces cerevisiae.34 The tripeptides liberated from Evolus fermented milk was also found to reduce the blood pressure.35 del Mar Contreras et al.36 also observed the novel casein-derived peptides with antihypertensive activity. Working on similar lines, Jauhiainen et al.37 observed that milk products containing bioactive tripeptides have an antihypertensive effect in double transgenic rats (dTGR) harbouring human renin and human angiotensinogen genes. Lehtinen et al.38 revealed the significance of milk casein-derived tripeptides Ile-Pro-Pro, Val-Pro-Pro, and Leu-Pro-Pro.
Inhibition of ACE, which is located in different tissues (e.g. plasma, lung, kidney, heart, skeletal muscle, pancreas brain), may influence different regulatory systems.39 When ACE-inhibitory peptides (Val-Pro-Pro and Ile-Pro-Pro) were given to spontaneously hypertensive rats, the blood pressure was reduced dose dependently. The peptide mixture or the fermented milk containing the peptides did not change the blood pressure40,41 detected two ACE-inhibitory tripeptides, present in fermented milk product, in aorta after oral administration of fermented milk in spontaneously hypertensive rats. Moreover, the ACE activity in fractions from aorta was lower in rats given fermented milk than in control group. However, de Leeuw et al.42 reported the dose-dependent lowering of blood pressure by dairy peptides in mildly hypertensive patient. The results indicate that these tripeptides are absorbed directly without being decomposed by digestive enzymes, reach the abdominal aorta, inhibit the ACE and show antihypertensive activity.43 Saito44 and Sanchez45 observed the antihypertensive peptides derived from bovine casein and whey proteins.
4. Peptides with antithrombotic properties
Cardiovascular disease represents the principal cause of mortality in industrial countries, and thrombosis is mainly implicated in this physiopathology of endothelial cells. Anti-thrombotic components maintain a natural equilibrium; a break down of this equilibrium leads to the formation of thrombosis through the creation of a surface where the platelets can accumulate. Subsequently, the platelets synthesize prostaglandin H2 which is transformed into thromboxan A2. Thromboxan A2 is a potent stimulator of platelet aggregation. Prothrombin is transformed in thrombin and an adenosine-di-phosphate (ADP) and calcium is released by the granules. Presence of the platelets on the surface stimulates aggregation and fibrinogen receptors, where platelets bind to fibrinogen and other adhesive proteins like fibronectine and the Von Willebrandt factor.46
Mechanisms involved in the clotting of milk (interaction of κ-casein with chymosin), bear a remarkable similarity to the processes involved in blood clotting (interaction of fibrinogen and thrombin).47 In fact, three amino acid residues viz. Ile108, Lys112, Asp115 of the unodecapeptide of κ-casein are in homologous position as compared with the R-chain sequence of human fibrinogen. A peptide derived from C-terminal and of κ-casein i.e. casoplatelin may compete with the r-chain of human fibrinogen for platelet receptors, thus inhibiting the clot formation.48 These also inhibit the aggregation of ADP activated platelets.
The glycomacropeptide obtained during digestion (f106–169) appears to limit clot formation by inhibiting Von Whillebrand factor dependent platelet aggregation. Based on these findings, GMP has been suggested for intravenous and oral administration in humans as a prophylaxis to prevent thrombosis.49
Casein and its derived peptides have most potent mineral binding properties among milk proteins and milk-originated bioactive peptides. Casein derived peptides generated either during in vitro hydrolysis, enzyme catalysis or in vivo by hydrolysis during GI tract digestion binds minerals i.e. calcium, copper, iron, zinc etc., via specific or non-specific binding sites. The most studied bioactive peptides are those derived from casein in particular caseinophosphopeptides (CPPs). Several phosphopeptides containing the cluster sequence -Ser(P)-Ser(P)-Ser(P)-Glu-Glu- have been identified from whole bovine casein. These sequences provide the peptides with the unique capacity to keep Ca, P and other mineral in a solution at intestinal pH. Several phosphopeptides have been identified from enzymatic digest of milk proteins, for example: αs1-casein f43–58, f59–79, f43–79, αs2-casein f1–24 and f46–70 and β-casein f1–28, f2–28, f1–25, f33–48.50 The highly anionic character of these peptides renders them resistant to further proteolytic attack, allows them to form soluble complexes with calcium and prevents the formation of insoluble calcium phosphate.51,52 The proportion of phosphopeptides interacting with colloidal calcium phosphate correlates with their relative content of phosphoserine residues.50 Various phosphopeptide fractions revealed significant differences in their calcium-binding activities, which may be due to variant amino acid composition around the phosphorylated region.53
The formation of caseinphosphopeptide has been observed during in vitro digestion of bovine caseins and specific caseinphosphopeptide residues have also been identified in the intestinal content of minipigs after ingestion of a diet containing casein.54 Caseinphosphopeptide can be formed also during cheese ripening due to plasmin and microbial protease activity during ripening.55,56
The binding of minerals serve two purposes: (a) bound minerals may more readily absorb across the intestinal epithelium,29 entrapment of certain minerals such as iron in a peptide may limit intra luminal availability and therefore depress intestinal microbial growth57; and (b) CPP can have an anti carcinogenic effect by promoting both recalcification of tooth enamel and by inhibiting the adhesion of plaque forming bacteria. The addition of CPP'S to tooth paste formulas has been suggested to have anti-cariogenic effects and to prevent enamel demineralization.58
Milk and dairy products are excellent source of calcium and its believed that CPPs produced during GI tract digestion of casein enhances the bioavailability of calcium in the diet. This proposed increase in bioavailability of calcium by CPPs has been attributed to their ability to increase solubility of calcium in alkaline environment of small intestine where maximal absorption of dietary calcium thought to occur.
6. Peptides with immunomodulating activities
Immunomodulating peptides have been detected in human as well as in cow milk proteins.59 The two peptides, namely β-casein f54–59 and α-lactalbumin f51–53, digest human milk protein and enhance the phagocytic activity of macrophages both in mice and humans, and enhance resistance against certain bacteria in mice.60,61 Among the immunomodulating peptides isolated from bovine caseins, the β-casein f191–193, αs1-casein C-terminal hexapeptide (f194–199) stimulated macrophages. β-casein f63–68 stimulated in vitro phagocytosis, but this peptide as well as β-casein f191–193 failed to exert protection of mice in vivo.62 The physiological mode of action is not yet known, but they may stimulate the proliferation and maturation of immune system cells. Synthetic peptides derived from milk proteins have been shown to enhance proliferation of human peripheral blood lymphocytes. These peptides were Tyr-Gly and Tyr-Gly-Gly and they correspond to fragments of bovine κ-casein and α-lactalbumin. β−casomorphin-7 and β-casokinin-10 showed suppression and stimulation of lymphocyte proliferation depending on the peptide concentration.63 Laffieneur et al.64 have shown that β-casein fermented by lactic acid bacteria have immunomodulatory activity which might be related to interaction with monocyte-macrophage and T-helper cells, especially Th1-like cells. Sütas and coworkers65 showed that caseins hydrolyzed with Lactobacillus GG and digestive enzymes generate compounds with suppressive effects on lymphocyte proliferation. Several known immunostimulating peptides were identified from these hydrolysates.66
Another group of peptides which may be implicated in the stimulation of immunosystem are the ACE-inhibitors. Inhibition of ACE favors bradykinin formation and thus acts as immunomodulators. Bradykinin, known as a mediator of the acute inflammatory process, is able to stimulate macrophages to enhance lymphocyte migration and increase secretion of lymphokinines.67 In this context, it should be emphasized that peptides αs1-casein f194–199, β-casein f60–66 and f193–202 have shown to have both immunostimulatory and ACE-inhibitory activities (Table 2).
The structure–activity relationship and the mechanism by which milk-derived peptides exert their immunomodulatory effects is not yet defined. It has been suggested that arginine in the N- or C-terminal region of peptide is important structural entity recognized by specific membrane bound receptors.67 A common structural feature among some immunomodulatory peptides is the presence of arginine in the C-terminal.
7. Peptides with antimicrobial properties
These are positively charged, cationic, hydrophobic peptides derived from milk proteins.68,69 These peptides inhibit the growth of most of the spoilage and few pathogenic microorganisms. However, gram-negative organisms are more sensitive than gram positive organisms.
7.1. From caseins
Caseins are random coil type proteins with nutritional and calcium modulation function.70 The finding of an antibacterial αS2 casein fragment in acid treated milk assigned a new biological function to the casein.71
Lahov et al.72 isolated basic antibacterial glycopeptides that were released from cow's milk by heating. They showed that heating and chymosin digestion of milk produced similar, basic high molecular weight polypeptides called casecidins, which inhibited the growth of pathogenic bacteria as well as lactobacilli. These experiments used α-casein, which subsequently are shown to consist of αS1 – casein and κ-casein. Hill et al.73 treated αS1- and κ-casein with chymosin at pH 6.4, which produced a basic peptide containing residues 1–23 of the original casein protein. Jäkälä et al. reported the casein-derived bioactive tripeptides Ile-Pro-Pro and Val-Pro-Pro with anti hypertension activity and improved endothelial function in salt-loaded Goto-Kakizaki rats. Jäkälä et al.74 also reported tripeptides Ile-Pro-Pro and Val-Pro-Pro playing significant role in protecting endothelial function in vitro in hypertensive rats. They observed the cardiovascular activity of milk casein-derived tripeptides and plant sterols in spontaneously hypertensive rats.75
Interest in the antibacterial activity of milk began in 1968 when a group from Weizmann Institute of Science in Israel filed a series of patents dealing with the isolation of novel polypeptides derivatives of casein.76
7.1.1. Isracidin.
Isracidin was obtained by the hydrolysis of the αS1 – casein by pepsin or chymosin. It is a polypeptide of mol wt. 2770 Da, and consist of N-terminal segment (1–23) of αS1 casein B.76 Isracidin differs from other casein – derived glycopeptides or peptides, not only in the absence of amino-sugar residues, but also in its amino acid composition.77 Isracidin was found to inhibit the in vitro growth of lactobacilli, which led to study of its broader antibacterial action. In vitro, bactericidal affects were found against a variety of Gram-positive bacteria, but only at the high concentrations of 0.1–1 mg ml−1.
Sequence of purified Isracidin.
NH2 – Arg – Pro – Lys – His – Pro – Ilu – Lys – His – Gln – Gly – Leu- Pro – Glu – Gln – Val – Leu – Asn – Glu – Asn – Leu – Leu – Arg – Phe – CCOH
Pure Isracidin is a white, fluffily substance with a yield of 1.44 g or 4.8% of the total casein processed. Isracidin to be effective had to be given prior to bacterial challenge. It exerted protective effect against strains of Staphylococcus aureus, Streptococcus pyogenes and Listeria monocytogenes.
7.1.2. Casocidin.
Zucht et al.71 isolated antibacterial peptide from bovine milk inhibiting the growth of Escherichia coli and Staphylococcus carnosus. The sequence of this peptide is
CTEEEKNRINFLKKISQRYQKFALPQYLKTVYQHQK from αS2 casein (165–203). Basic residues are types in boldface. It is 32 amino acid peptide with a molecular weight of 4870 Da. Due to the regular alternation of hydrophobic and hydrophilic residues, this gives rise to the hypothesis that the mechanism of action resembles that of amphiphatic defensins permeabilizing bacterial membranes. Casein αS2 is not present in human milk, hence casocidin-I or related peptides of bovine milk influence the human intestinal flora, particularly of the sucklings.78 However, these were found to be more effective against gram positive bacteria than gram negative bacteria.
Recio and Visser79 isolated two distinct domains from a peptic hydrolysate from bovine αS2 casein. The two fragments include of (183–207) and of (164–179). The previously reported casocidin I71 was identified as fragment of (165–203) of the immature precursor casein, which corresponds to fragment (150–188) of presently used sequence of nature protein. Both fragments showed activity against Gram positive and Gram-negative bacteria but (183–207) was consistently more active than (164–179). S. thermophilus was highly sensitive than strains E. coli. Undigested αS2-casein did not show antibacterial activity when tested against E. coli or M. flavus.
A distinctive structural feature of both identified domains is the relatively high proportion and asymmetric clustering of basic amino acid residues. The fragment f(164–179) containing four basic amino acid residues out of 16, has more net positive charge than f(183–207) with 5 or 6 basic amino acid out of 25. But b(164–179) exhibited weaker antibacterial activity a similar hemolysis percentage compared to b(183–207).
7.2.1. α-Lactoalbumin.
Lactoalbumin shares a striking homology with lysozyme with respect to amino acid sequence, protein and gene structures. Despite these structural similarities La and lysozyme differ strongly in their biological function. Lysozyme possesses bactericidal properties due to its enzymatic activity of hydrolyzing the peptidoglycan layer of gram positive bacteria where as the primary function of α-LA is to modulate the substrate specificity to the lactose synthase complex from N-acetyl glucosamine to glucose, permitting the formation of lactose in the lactating udder. The bactericidal active polypeptide fragments of α-LA are located in the non homologous part of the sequence of Lysozyme. Pellegrini80 reported α-LA digested with trypsin yielded at least eight polypeptides.
Fraction |
Sequence |
pI |
LDT1 |
EQLTK (1–5) |
6.1 |
LDT2 |
GYGGVSLPWEWV CTTFALCSEK (17–31)S–S(109–144) |
4.5 |
LDC |
CKDDQNPHISCDKF (61–68)S–S(75–80) |
5.3 |
α-LA digested with pepsin does not yield any bactericidal peptides. All these peptides were found to be most active against gram positive bacteria and weakly active against gram negative bacteria. B. subtilis was found to be most susceptible organism, and LDT2 was found to be most bactericidal of all peptides against B. subtilis and S. epidermis in terms of bactericidal activity, followed by LDC and LDT1 i.e. LDT2 > LDC > LDT1.
The antimicrobial peptides of α-LA were negatively charged with their pI indicated in the table. This may explain why they are weak against gram negative bacteria whose outer membranes contain negatively charged lipopolysaccharide as a major component.
7.2.2. β-Lactoglobulin.
This is the major whey protein in the milk of ruminants. It is absent in the milk of human and rodents.81 It is an anionic protein with PI of 4.8 and molecular weight 18
280 Da. Its tertiary structure shares strong homology with the human serum retinol binding protein. The main protein involved in the transport of retinol in serum. In fact β-lactoglobulin forms a water soluble complex with retinol.
Pellegrini80 reported that the digestion of lactoglobulin with trypsin yielded negatively charged peptides.
Fraction |
Sequence |
pI |
LGDT1 |
IPAVFK (78–83) |
4.2 |
LGDT2 |
VAGTWY (15–20) |
5.5 |
LGDT3 |
VLVLDTYK (92–100) |
4.2 |
LGDT4 |
AASDISLLDAQISAPLR (25–40) |
4.2 |
The anionic bactericidal peptides derived from the proteolytic digestion of Lactoglobulin were active peptides against gram positive bacteria only.82 However, the cationic peptide LGDT1, which was also hydrophobic, was only effective against gram positive bacteria. This result indicates that apart from charge and hydrophobicity, other factors play an important role in bactericidal activity. The replacement of Asp in LGDT3, a amphipathic peptide with Arg, and the addition of Lys at the C terminal of LDGT381 changed this peptide to cationic with PI 9.7 displayed a wider bactericidal activity than native LGDT3 inhibiting E. coli and B. bronchiseptica two gram negative bacteria. This suggests a potential therapeutic use of modified Lg as functional food or infant formula. Several drugs do cause problems when taken orally, however, modified Lg carry microbicidal peptides through the GI tract and release it after natural digestion of the carrier protein in blood, offers a great advantage.
7.3. Lactoferrin
Lactoferrin is an iron binding protein of the transferrin family having broad spectrum of antimicrobial properties. It is considered as an important component of the host defence system active at mucosal surfaces and in colostrum and milk.83–85 The lactoferrin concentration is higher than 2 mg ml−1 in human milk86 and is in the range of 0.02–0.2 mg ml−1 in bovine milk.87,88 Human lactoferrin has a molecular weight of 82.4 KDa, and is composed of 70289 or 692 amino acid residues.90,91 Bovine lactoferrin has a molecular weight of 83.1 KDa, and is composed of 689 aminoacid residues.92,93
Lactoferrin inhabits the growth of many gram positive and gram negative bacteria, some yeast and moulds and some kind of parasites. Lactoferrin is known to act synergistically with lysozyme94 and IGA95 in various secretary fluids. The bacteriostatic effect of lactoferrin are thought to be due to its ability to sequester environmental iron96 because some kind of bacteria such as E. coli secrete chelators to enhance iron uptake. Lactoferrin molecule can bind to Fe3+ ions, one within each lobe and HCO3− ion necessary for iron binding. Normally lactoferrin contains iron ions and the degree of iron saturation is 10 to 30% in milk. However, apo-lactoferrin has been shown to bind the outer membrane of gram-negative bacteria, causing the release of LPS and direct destruction of the bacterial cells.97
7.3.1. Antimicrobial peptide derived from lactoferrin.
The hydrolysates produced by pepsin cleavage of bovine and human lactoferrin were found to contain a potent bactericidal peptide lactoferricin B and lactoferricin H.98 The microbial effects of these peptides were found to be 10 to 100 times stronger than that of undigested lactoferrin. Further, lactoferricin B should show stronger antimicrobial activity in vitro than lactoferricin H.99 Lactoferricin B is derived from a region away from the iron binding site, providing a new antibacterial mechanism for lactoferrin, which seems to be more complex than a simple binding of iron.
Lfcin B is a 25 amino acid peptide with the sequence FKCRRWQWRMKKLGAPSITCVRRAF. The two cysteines are linked by a disulfide which is also present in intact lactoferrin but this is not essential for bactericidal activity.100
It is highly cationic peptide with 8 basic residues, considerable potential exists for the wide spread commercial use of lactoferricin B as a natural antimicrobial agent in a variety of product applications. Bovine lactoferrin is now readily available as a product of dairy industry and with this protein as the starting material, the large scale manufacture of Lfcin B is readily achievable.101
7.3.2. Antifungal activity of Lfcin-B.
Dermatophytes are a group of specialized filamentous fungi possessing keratinolytic activity. This unusual feature enables them to infect fully keratinized epidermal tissue (i.e. skin, hair and nails) in humans and animals causing diseases known as tineas or ringworm. A number of yeast and filamentous fungi including agents of skin disease (dermatophytes) are susceptible to inhibition by lactoferricin B in the concentration range of 3–60 μg ml−1 depending on the strain and medium used. Lfcin B inhibited fungal uptake of H-glucose with effectiveness similar to polymyxin B. This suggests that the cell binding events may lead to a disruption of normal cell membrane functions.101
7.3.3. Antiviral activity of lactoferrin.
Lactoferrin was found to inhibit viral infection from herpes simplex virus, cytomegalovirus, HIV and rotavirus. Antiviral activity of lactoferrin was observed with the apo-form and the protein saturated with iron and other metals. The antiviral activity of lactoferrin appears to be the molecular recognition of the protein moiety. Lactoferrin binds to viral surface proteins, for e.g. to the V3100p of the gp120 envelope protein of HIV. Lactoferrin therefore could represent an ideal antiviral agent since it is a natural immunity factor interfering with viral replication at the level of the viral attachment, without affecting normal host cell metabolism.102
7.3.4. Mechanism of action (binding of lactoferricin B to the cell).
Lactoferricin B binds to the surface of both E. coli and B. subtilis and maximal binding occurred within 10 min. This rate of binding was consistent with the rapid rate of killing.98 The extent of binding was dose dependent and at high concentrations the number of peptide molecules was greater than 106 per cell with each strain, indicating the binding of Lfcin B to highly repeated surface component of the cell. The number of peptide molecules bound per cell was about 5-fold higher with B. subtilis than with E. coli. The cell binding of C14 Lfcin B was optimum at PH 6 with E. coli and pH 7.5 with B. subtilis. The presence of negatively charged teichoic and teichuronic acid on gram positive bacteria were found to be the potential binding sites of Lfcin B.
There are two possible modes in which lactoferricin binds to the membranes:
Formation of oligomeric pores: Lfcin B would insert into the membrane so that its extended strands lie perpendicular to the membrane surface. Several Lfcin B monomers would multimerise so that the outer hydrophobic surface would interact with the membrane, while the inner hydrophilic surface forms the inside of a transmembrane channel. This mechanism has also been proposed for the defensins.103
On an alternative mode, Lfcin B interacts with a single leaflet of the lipid bilayer, so that its back bone lies parallel to the membrane surface. This mode of binding would be similar to that determined for the antimicrobial peptide meganin-2.100
Role of aminoacids.
A short hexa peptide fragment of Lfcin B RRWQWR-NH2 emphasizes the importance of Trp and Arg residues for antimicrobial activity. Trp has partly hydrophobic-hydrophilic character so that it prefers to partition at the hydrophobic-hydrophilic boundary of the membrane acting as membrane anchors. The hexa peptide might be expected to lie parallel to the membrane surface in an extended conformation so that the Arg and Gln interact with the hydrophilic components, while the Trp residues are placed at the membrane interface of Lfcin B were to lie parallel to the membrane, all of these residues could be placed on the same side of the β-sheet. The β-sheet structure of Lfcin B seems to be better suited for making initial contact with membrane than the more helical structure found in intact lactoferrins.
The hydrophobic residues could interact with the fatty acyl group of the lipid bilayer while the positively charged Arg and Lys side chains interact with phosphodiester groups. Upon membrane binding, an even more efficient partitioning of hydrophobic and hydrophilic residues would be expected, Phe l, Lys 2, Met 10, Ala 24 and Phe 25 would all be able to adjust to the membrane environment, along with the long Lys and Arg side chains.100
7.4. Glycomacropeptide (GMP)
The casein macropeptide is found in sweet whey. It is derived from the action of chymosin on κ-casein at Phe-105-Met-106 bond.104 It is more hydrophilic with C terminal portion of the molecule containing oligosaccharides that are O-linked to threonine and serine. GMP is lacking in aromatic amino acids and shows no absorption at 280 nm, therefore it is used in the diets of phenyl ketunuria (PKU) patients who lack the ability to Metabolize Phenyl alanine amino acid. GMP retains a net negative charge even at pH 3. Therefore, it is not collected on cation exchanger nor does it move with rest of proteins in native PAGE. Quantification procedures of GMP require tricholoroacetic acid precipitation of other whey proteins leaving only GMP in solution.105
The biological and physiological properties attributed to GMP or peptides derived from GMP includes:
• Dental plaque and dental caries inhibition by preventing the adhesion of oral actinomyces (A. viscosus) and streptococci (S. mutans, S. sanguis and S. sobrinus).106
• Inhibition of influenza virus haemagglutinin.107
• Inhibition of binding of cholera toxin and E. coli enterotoxins to its receptors.108
• Growth promoting activity for a bifidobacteria.108
Most of the studies of the biological activity of GMP have been done in vitro and require in vivo conformation. In some cases such as inhibition of bacterial adhesion106 or regulation activity in the digestive tract, the GMP act without being adsorbed. Other actions such as immunomodulation or interactions with blood components would be expected to require absorption. This would occur following the action of digestive enzymes with the formation of smaller fragments with or without associated carbohydrates.109
Potential clinical/commercial applications of GMP.
Reliable application of GMP could benefit human health as well as provide technical support for functional food types produced by dairy industry. Kawasaki et al.112 obtained a patent for the use of GMP to neutralize endotoxin. Dosako et al.113 obtained a patent related to prevention of E. coli adhesion and protein against Epstein–Barr virus of 1 mg of SMP per day was enough to protect a mouse from bacterial toxins then one might protect a child. However, Nesser et al.106 showed that the presence of O-lined instead of N-linked saccharide makes it ineffective against E. coli adhesion. Perhaps, GMP could provide an inexpensive source for free oligosaccharide or synthetic oligosaccharide derivatives which could afford antibacterial protection.
Stan et al.114 proposed that reducing gastric secretions and stomach mixing would reduce proteolysis in young animals thus preserving milk derived immunoglobulins, lactoferrin and lysozyme to pass through the stomach intact. These proteins could then provide protection in the intestine. If extended to humans, it would benefit infant feeding and immune-compromised patients.109
8. Other activities of milk bioactive peptides
Yamauchi116 has reported that two peptides derived from serum albumin and β-lactoglobulin induced contraction of guinea pig ileum longitudinal muscle when the test was completed without electric stimulation in the absence of agonist. The peptides were referred to as “peptides acting on smooth muscle” and they contained serum albumin f208–216 (albutensin A) and β-lactoglobulin f146–149 (β-lactotensin). β-lactotensin was released during hydrolysis with chymotrypsin and it had a non-opioid contracting effect on guinea pig smooth muscle.117
Some of the peptides derived from milk proteins have more than one functional significance,117 peptides from the sequence 60–70 of β-casein show immunostimulatory, opioid and ACE-inhibitory activities. Such sequence is defined as strategic zone.59 This sequence is protected from proteolysis because of its high hydrophobicity and the presence of proline residues. In addition to the strategic zone, some other multifunctional peptides can be liberated from milk proteins, for example αs1-casein f194–199 and β-casein f177–183 have immunomodulatory and ACE-inhibitory activity. Multifunctional peptides such as β-lactoglobulin f102–105 (β-lactorphin) showing both ACE-inhibitory and opioid activity can also be liberated from whey proteins. Sieber et al.118 reported the ACE-inhibitory activity and ACE-inhibiting peptides in different cheese varieties.
Among the most intriguing functions of milk is its ability to discourage the growth of undesirable or enteropathogenic bacteria (such as coliform bacteria) in the gut of the neonate or growing suckling while enhancing the growth of beneficial bacteria such as bifidobacteria and lactobacilli. Several factors are known to enhance the growth of bifidobacteria, milk especially colostrum milk contains a family of oligosaccharides comprised of lactose or N-acetyllactosamine oligomers with side glycosylation by fucose or sialic acid. The bioactive peptides may interact with non-peptide components of milk for a particular bioactivity.119,120 A similar set of peptide and non peptide milk components interact to provide broad antiviral defences in the gut; immunoglobulins provide specific antibodies and protection against a given virus, lactoferrin may nonspecifically block infection of gut epithelial cells by some but probably not all viruses, and milk oligosaccharides, glycosaminoglycans, and glycolipids inhibit viral infection by completely blocking virus binding to intestinal epithelial cell surfaces.
9. Possible applications of bioactive peptides
Peptides with biological activity could be produced in several ways.3 The most common methods are: processing of foods using heat, alkali or acid conditions that hydrolyze proteins, enzymatic hydrolysis of food proteins and microbial activity of fermented foods. Although bioactive peptides do exist in a number of processed and fermented foods, their true physiological functions in humans are unknown.121 In a healthy individual, eating a varied diet, the presence of bioactive peptides may help keep the nervous, immune and digestive systems in a well-maintained state. The future potential value of bioactive peptides in the diet may be their ability to affect certain pathological conditions, although this has yet to be proven. Casein derived peptides have already found interesting applications as dietary supplements (phosphopeptides) and as pharmaceutical preparations (phosphopeptides, β-casomorphins).122,123 The efficacy and safe conditions of use of these peptides in animals and in humans remain to be proven. At present, ACE-inhibitory peptides and phosphopeptides are an important area31,32 in which bioactive peptides may be found to be useful ingredients for dietary applications.
10. Conclusions
Enzymatic digestion of milk proteins represents an important supply of numerous peptides that may have biological activity. The physiological role of these peptides is not yet fully understood. Peptides have been shown to exert beneficial physiological effects i.e. regulation of blood pressure, immunomodulation etc. These activities are mostly targeting the pathways like opoid system (agonist and antagonist), ACE inhibition, immune stimulation (infections etc.)/suppression (allergic diseases) and anti-thrombic actions. These findings introduce new perspectives in the nutritional and technological evaluation of milk and milk products. These milk peptides may be considered as food additive and perhaps as starting components for some drug developments. Casein derived peptides have already found interesting applications as dietary supplements and pharmaceutical preparations. Today, we know that some of the biologically active peptides can be released during the in vivo digestion, however we don't know if these peptides have physiological function. More research is needed to fully understand the functional significance of these substances.
11. References
- M. Dziuba, B. Dziuba and A. Iwaniak, Acta Sci. Pol., Technol. Aliment., 2009, 8, 71–90 Search PubMed.
- F. Tidona, A. Criscione, A. M. Guastella, A. Zuccaro, S. Bordonaro and D. Marletta, Italian Journal of Animal Science, 2009, 8, 315–340 Search PubMed.
- H. Korhonen and A. Pihlanto, Int. Dairy J., 2006, 16, 945–960 CrossRef CAS.
- P. Jäkälä, A. Hakala, A. Turpeinen, R. Korpela and H. Vapaatalo, J. Funct. Foods, 2009, 1, 366–374 Search PubMed.
- S. V. Silva and F. X. Malcata, Int. Dairy J., 2005, 15, 1–15 CrossRef CAS.
- P. Minkiewicz, J. Dziuba, A. Iwaniak, M. Dziuba and M. Darewicz, J. AOAC Int., 2008, 91, 965–980 CAS.
- H. J. Korhonen, J. Funct. Foods, 2009, 1, 177–187 Search PubMed.
- R. Quirion and A. S. Weiss, Peptides, 1983, 4, 445–449 CrossRef CAS.
- V. Brantl, H. Teschemacher, A. Henschen and F. Lottspeich, Hoppe Seylers Zournal Physiology and Chemistry, 1979, 360, 1211–6 Search PubMed.
- K. J. Chang, Y. F. Su, D. A. Brent and J. K. Chang, J. Biol. Chem., 1985, 260, 9706–12 CAS.
- E. Schlimme and H. Meisel, Nahrung, 1995, 39, 1–2 CrossRef CAS.
- S. Srinivas and V. Prakash, Int. J. Pept. Res. Ther., 2010, 16, 7–15 Search PubMed.
- S. Loukas, D. Varoucha, C. Zioudrou, R. A. Streaty and W. A. Klee, Biochemistry, 1983, 22, 4567–7 CrossRef CAS.
- C. Zioudrou, R. A. Streaty and W. A. Klee, J. Biol. Chem., 1979, 254, 2446–9 CAS.
- M. Yoshikawa, F. Tani, T. Yoshimura and H. Chiba, Agricultural Biology and Chemistry, 1986, 50, 2419–2421 Search PubMed.
- A. Pihlanto-Leppälä, P. Antila, P. Mäntsälä and J. Hellman, Int. Dairy J., 1994, 4, 281–301.
- F. Addeo, L. Chianese, A. Salzano, R. Sacchi, U. Cappuccio, P. Ferranti and A. Malorni, J. Dairy Res., 1992, 59, 401–411 CrossRef CAS.
- H. Meisel, FEBS Lett., 1986, 196, 223–227 CrossRef CAS.
- J. Svedberg, J. de Haas, G. Leimenstoll, F. Paul and H. Teschemacher, Peptides, 1985, 6, 825–830 CrossRef CAS.
- A. R. Madureira, C. I. Pereira, A. M. P. Gomes, M. E. Pintado and F. Xavier Malcata, Food Res. Int., 2007, 40, 1197–1211 CrossRef CAS.
-
F. Tani, A. Shiota, H. Chiba and M. V. Yoshikawa. Brantl and H. Teschemcaher, Weinheim, VCH-Verlag, 1994, pp. 49–53.
- P. Antila, I. Paakkari, A. Järvinen, M. J. Mattila, M. Laukkanen, A. Pihlanto-Leppälä, P. Mäntsälä and J. Hellman, Int. Dairy J., 1991, 1, 215–229 CrossRef CAS.
- S. M. Kamau, S. C. Cheison, W. Chen, X. M. Liu and R. R. Lu, Compr. Rev. Food Sci. Food Saf., 2010, 9, 197–212 CrossRef CAS.
-
I. Paakkari, A. Järvinen, P. Antila, M. J. Mattila and A. Pihlanto-Leppälä, V. Brantl and H. Teschemacher. Weinheim, New York, VCH, 1994, pp. 33–37.
- H. Teschemacher, M. Umbach, U. Hamel, K. Praetorius, G. Ahnert-Hilger, V. Brantl, F. Lottspeich and A. Henschen, J. Dairy Res., 1986, 53, 135–138 CrossRef CAS.
- D. Tome, A. M. Dumontier, M. Hautefeuille and J. F. Desjeux, Am. J. Physiol., 1987, 253, G737–G744 CAS.
- H. Daniel, M. Vohwinkel and G. Rehner, J. Nutr., 1990, 120, 252–257 CAS.
- H. Teschemacher, G. Koch and V. Brantl, Biopolymers, 1997, 43, 99–117 CrossRef CAS.
- H. Meisel, A. Goepfert and S. Gunther, Milchwissenschaft, 1997, 52, 307–311 Search PubMed.
- J. M. Steijns, Int. J. Dairy Technol., 2001, 54, 81–88 CrossRef CAS.
- M. Foltz, L. van Buren, W. Klaffke and G. S. M. J. E. Duchateau, J. Food Sci., 2009, 74, 243–251.
- J. Meyer, U. Bütikofer, B. Walther, D. Wechsler and R. Sieber, J. Dairy Sci., 2009, 92, 826–836 CrossRef CAS.
- H. Meisel, Int. Dairy J., 1998, 8, 363–373 CrossRef CAS.
- Y. Nakamura, N. Yamamoto, K. Sakai, A. Okubo, S. Yamazaki and T. Takano, J. Dairy Sci., 1995, 78, 777–783 CrossRef CAS.
- P. Jäkälä and H. Vapaatalo, Pharmaceuticals, 2010, 3, 251–272 Search PubMed.
- M. del Mar Contreras, R. Carrón, M. J. Montero, M. Ramos and I. Recio, Int. Dairy J., 2009, 19, 566–573 CrossRef.
- T. Jauhiainen, T. Pilvi, Z. J. Cheng, H. Kautiainen, D. N. Müller, H. Vapaatalo, R. Korpela and E. Mervaala, Journal of Nutritional Metabolism, 2010 DOI:10.1155/2010/287030.
- R. Lehtinen, T. Jauhiainen, E. Kankuri, K. Lindstedt, P. T. Kovanen, O. Kerojoki, R. Korpela and H. Vapaatalo, Arzneimittelforschung, 2010, 60, 182–5 CAS.
- M. A. Ondetti and D. W. Cushman, Annu. Rev. Biochem., 1982, 51, 283–308 CrossRef CAS.
- Y. Nakamura, N. Yamamoto, K. Sakai and T. Takano, J. Dairy Sci., 1995, 78, 1253–1257 CrossRef CAS.
- M. S. Nielsen, T. Martinussen, B. Flambard, K. I. Sørensen and J. Otte, Int. Dairy J., 2009, 19, 155–165 CrossRef CAS.
- P. W. deLeeuw, K. van der Zander, A. A. Kroon, R. M. Rennenberg and M. M. Koning, Blood Press, 2009, 18, 44–50 CrossRef CAS.
- E. Boelsma and J. Kloek, Br. J. Nutr., 2009, 101, 776–786 CrossRef CAS.
- T. Saito, Adv. Exp. Med. Biol., 2008, 606, 295–317 CAS.
-
R. Sanchez, Q. Del, H. Ledesma, G. Ruiz, J. Angel, M. Castro, A. Garrido, M. Lourdes, L. Exposito, R. Gonzalez, M. Mercedes, A. De, A. Amaya and G. M. Contreras, Bioactive peptides identified in enzymatic hydrolyzates of milk caseins and method of obtaining same, 2010, United States Patent Application 20100048464 (Application Number: 11/921797; Publication Date: 02/25/2010) Search PubMed.
- S. Fosset and D. Tome, Dietary protein-derived peptides with antithrombotic activity, Bull. Int. Dairy Fed., 1998, 353, 11–15 Search PubMed.
- D. A. Clare and H. E. Swaisgood, J. Dairy Sci., 2000, 83, 1187–1195 CrossRef CAS.
- K. J. Rutherford, H. S. Gill and J. Br., Nutrition., 2000, 84, 99–102.
- H. Meisel, Aust. J. Dairy Technol., 2001, 56, 83–93 Search PubMed.
- V. Gagnaire, D. Molle, M. Herrouin and J. Leonil, J. Agric. Food Chem., 2001, 49, 4402–4413 CrossRef CAS.
- R. Berrocal, S. Chanton, M. A. Juilleart, B. Pavillard, J. C. Scherz and R. Jost, J. Dairy Res., 1989, 56, 335–341 CrossRef CAS.
- R. Sato, T. Noguchi and H. Naito, J. Nutr. Sci. Vitaminol., 1986, 32, 67–76 Search PubMed.
- H. Meisel, S. Behrens and E. Schlimme, Kiel. Milchwirtsch. Forschungsber., 1991, 43(3), 199–212 Search PubMed.
- H. Meisel and H. Frister, J. Dairy Res., 1989, 56, 343–349 CrossRef CAS.
- F. Roudot-Algaron, D. LeBars, L. Kerhoas, J. Einhorn and J. C. Gripon, J. Food Sci., 1994, 59, 544–549 CrossRef CAS.
- T. K. Singh, P. F. Fox and A. Healy, J. Dairy Res., 1997, 64, 433–443 CrossRef CAS.
- G. E. Vegarud, T. Langsurd and C. Svenning, Br. J. Nutr., 2000, 84, S91–S98 CAS.
- R. J. FitzGerald, Int. Dairy J., 1998, 8, 451–457 CrossRef CAS.
- D. Migliore-Samour and P. Jollés, Experientia, 1988, 44, 188–193 CrossRef CAS.
- D. Migliore-Samour, F. Floc'h and P. Jollés, J. Dairy Res., 1989, 56, 357–362 CrossRef CAS.
- F. Parker, D. Migliore-Samour, F. Floc'h, A. Zerial, G. H. Werner, J. Jollés, M. Casaretto, H. Zahn and P. Jollés, Eur. J. Biochem., 1984, 145, 677–682 CrossRef CAS.
- A. M. Fiat, D. Migliore-Samour, P. Jolles, L. Drouet, C. Baldit Sollier and J. P. Caen, J. Dairy Sci., 1993, 76, 301–310 CrossRef CAS.
- H. Kayser and H. Meisel, FEBS Lett., 1996, 383, 18–20 CrossRef CAS.
- E. Laffineur, N. Genetet and J. Leonil, J. Dairy Sci., 1996, 79, 2112–2120 CrossRef CAS.
- Y. Sütas, E. Soppi, H. Korhonen, E.-L. Syväoja, M. Saxelin, T. Rokka and E. J. Isolauri, J. Allergy Clin. Immunol., 1996, 98, 216–224 CrossRef CAS.
- T. Rokka, E.-L. Syväoja, J. Tuominen and H. Korhonen, Milchwissenschaft, 1997, 52, 675–678 Search PubMed.
- I. Pagelow and H. Werner, Experimental and Clinical Pharmacology, 1986, 8, 91–95 Search PubMed.
- E. Haque and R. Chand, Eur. Food Res. Technol., 2008, 227, 7–15 CrossRef CAS.
- A. R. Madureira, T. Tavares, A. M. P. Gomes, M. E. Pintado and F. X. Malcata, J. Dairy Sci., 2010, 93, 437–455 CrossRef CAS.
- M. Phelan, A. Aisling, R. J. Fitzgerald and N. M. O'Brien, Int. Dairy J., 2009, 19, 643–654 CrossRef CAS.
- H. D. Zucht, M. Raida, K. Adermann, H. J. Magret and W. G. Forssmann, FEBS Lett., 1995, 372, 185–188 CrossRef CAS.
- E. Lahov, D. Edelsten, M. T. Sode-Morgensen and E. Sofer, Milchwissenschaft, 1971, 26, 489–495 Search PubMed.
- R. D. Hill, E. Lahov and D. Givol, J. Dairy Res., 1974, 41, 147–153 CrossRef CAS.
- P. Jäkälä, T. Jauhiainen, R. Korpela and H. Vapaatalo, J. Funct. Foods, 2009, 1, 266–273 Search PubMed.
- P. Jäkälä, E. Pere, R. Lehtinen, A. Turpeinen, R. Korpela and H. Vapaatalo, Journal of Physiology and Pharmacology, 2009, 60, 11–20 Search PubMed.
- E. Lahov and W. Regelson, Food Chem. Toxicol., 1996, 34, 131–145 CrossRef CAS.
- P. L. H. McSweeney, N. F. Olson, P. F. Fox, A. Healy and P. Hojrup, Food Biotechnol., 1993, 7, 143–158 Search PubMed.
- A. Haug, A. T. Hostmark and O. M. Harstad, Lipids Health Dis., 2007, 6, 25 CrossRef.
- I. Recio and S. Visser, J. Chromatogr., A, 1999, 831, 191–201 CrossRef CAS.
- A. Pellegrini, Curr. Pharm. Des., 2003, 9, 1225–1238 CrossRef CAS.
- G. Kontopidis, C. Holt and L. Sawyer, J. Dairy Sci., 2004, 87, 785–796 CrossRef CAS.
- B. Hernandez-Ledesma, I. Recio and L. Amigo, Amino Acids, 2008, 35, 257–265 CrossRef CAS.
- J. H. Brock, Arch. Dis. Child., 1980, 55, 417–421 CrossRef CAS.
- J. J. Bullen, Review of Infectious Diseases, 1981, 3, 1127–1138 Search PubMed.
- B. Reiter, International Journal of Tissue Reactions, 1983, 5, 87–96 Search PubMed.
- T. Nagasawa, I. Kiyosawa and K. Kuwahara, J. Dairy Sci., 1972, 55, 1651–1659 CrossRef CAS.
- P. L. Masson and J. F. Heremans, Comp. Biochem. Physiol. B., 1971, 39, 119–129 CrossRef CAS.
- T. Suzuki, M. Nonaka, I. Kiyosawa and K. Ogasa, Nutrition and Food, 1977, 30, 317–322 Search PubMed.
- M. H. Metz-Boutigue, J. Jolles and J. Mazuries, Eur. J. Biochem., 1984, 145, 659–676 CrossRef CAS.
- M. J. Powell and J. E. Ogden, Nucleic Acids Res., 1990, 18, 4013–4018 CrossRef CAS.
- M. W. Rey, S. L. Woloshuk, H. A. deBoer and F. R. Pieper, Nucleic Acids Res., 1990, 18, 5288 CrossRef CAS.
- P. E. Mead and J. W. Tweedie, Nucleic Acids Res., 1990, 18, 7167 CrossRef CAS.
- A. Pierce, D. Colavizza, M. Benaissa, P. Maes, A. Tartra, J. Montreuil and G. Spik, Eur. J. Biochem., 1991, 196, 177–184 CrossRef CAS.
- J. Carlsson, J. Porath and B. Lönnerdal, FEBS Lett., 1977 Mar 15, 75(1), 89–92 Search PubMed.
- D. T. Akin, M. Q. Lu, S. J. Lu, S. Kendall, J. Rundegren and R. R. Arnold, Adv.
Exp. Med. Biol., 1994, 357, 61–70 CAS.
- R. R. Arnold, M. Brewer and J. J. Gauthier, Immunity, 1980, 28, 893–898 CAS.
- R. T. Ellison, III, Adv. Exp. Med. Biol., 1994, 35, 771–90.
- W. Bellamy, M. Takase, H. Wakabayashi, K. Kawase and M. Tomita, J. Appl. Bacteriol., 1992, 73, 472–479 CAS.
- W. Bellamy, H. Wakabavashi, M. Takase, K. Kawase, S. Shimamura and M. Tomita, Med. Microbiol. Immunol., 1993, 182, 97–105 CAS.
- P. M. Hwang, N. Zhon, X. Shan, C. H. Arrowsmith and H. J. Vogel, Biochemistry, 1998, 37, 4288–4298 CrossRef CAS.
- W. Bellamy, K. Yamauchi, H. Wakabayashi, M. Takase, N. Takakura, S. Shimamura and M. Tomita, Lett. Appl. Microbiol., 1994, 18, 230–233 CrossRef CAS.
- K. Shimazaki, Animal Science Journal, 2000, 71, 329–347 Search PubMed.
- C. P. Hill, J. Yee, M. E. Selsted and D. Eisenberg, Science, 1991, 251, 1481–85 CAS.
- A. Kumar, S. Grover, J. K. Sharma and V. K. Batish, Crit. Rev. Biotechnol., 2010, 30, 243–58 CrossRef CAS.
- S. K. Sharma, A. R. Hill and G. S. Mittal, Milchwissenschaft, 1993, 48, 71–73 Search PubMed.
- J. R. Nesser, A. Chambaz, S. D. Vedovo and M. J. Prigent, Guggenheim B. Infection and Immunology, 1988, 56, 3201–3208 Search PubMed.
- Y. Kawasaki, H. Isoda, H. Shimoto, M. Tanimoto, S. Dosako and T. Idota, Biosci., Biotechnol., Biochem., 1993, 57, 1214–1215 CrossRef CAS.
-
H. Isoda, Y. Kawasaki, M. Tanimoto, S. Dosako and T. Idota, Use of compounds containing or binding sialic acid to neutralize bacterial toxins, 1999 Search PubMed.
- E. Brody, Br. J. Nutr., 2000, 84, S39–S46 CAS.
- A. M. H. El-Salam, H. El-Shibiny and W. Buchheim, Int. Dairy J., 1996, 6, 327–341 CrossRef.
- S. C. Marshall, Food Research Quarterly, 1991, 51, 86–91 Search PubMed.
-
I. Kawasaki, K. Ykio, D. Sunichi and H. Otani, Neutralizing agent for an endotoxin comprising a fraction containing k-casein glycomacropeptide (GMP) as an active ingredient, 1995 Search PubMed.
-
S. Dosako, H. Kusano, E. Deya and T. Idota, Infection protectant, 1992 Search PubMed.
- E. Y. Stan, S. D. Groisman, K. B. Krasil'shchikov and M. P. Chernikov, Byulleten' Eksperimental'noi Biologii I. Metisiney, 1983, 96, 10–12 Search PubMed.
- K. Yamauchi, Bulletin of the IDF, 1992, 272, 51–58 Search PubMed.
- A. Pihlanto-Leppälä, I. Paakkari, M. Rinta-Koski and P. Antila, J. Dairy Res., 1997, 64, 149–155 CrossRef CAS.
- E. Haque, R. Chand and S. Kapila, Food Rev. Int., 2009, 25, 28–43 CrossRef CAS.
- R. Sieber, U. Bütikofer, C. Egger, R. Portmann, B. Walther and D. Wechsler, Dairy Science and Technology, 2010, 90, 47–73 Search PubMed.
- N. P. Möller, K. E. Scholz-Ahrens, N. Roos and J. Schrezenmeir, Eur. J. Nutr., 2008, 47, 171–182 CrossRef CAS.
- R. Nagpal, P. V. Behare, M. Kumar, D. Mohania, M. Yadav, S. Jain, S. Menon, O. Parkash, F. Marotta, E. Minelli, C. J. K. Gebrt and H. Yadav, Critical Reviews of Food Science and Nutrition, 2010 Search PubMed (in press).
- Ponstein-Simarro Doorten, A. Y. vdWiel and J. A. G. Jonker, Food Chem. Toxicol., 2009, 47, 55–61 CrossRef.
-
G. Brulé, L. Roger, J. Fauquant and M. Piot, Phosphopeptides from casein derived material, 1982, 4, 358, p. 465 Search PubMed.
- E. C. Reynolds, J. Dent. Res., 1987, 66, 1120–1127 CrossRef CAS.
- M. M. Mullally, H. Meisel and R. J. Fitzgerald, Biol. Chem. Hoppe-Seyler, 1996, 377, 259–60 CAS.
|
This journal is © The Royal Society of Chemistry 2011 |