DOI:
10.1039/C1CY00091H
(Communication)
Catal. Sci. Technol., 2011,
1, 569-573
C–S cross-coupling of thiols with aryl iodides under ligand-free conditions using nano copper oxide as a recyclable catalyst in ionic liquid†
Received
15th March 2011
, Accepted 27th April 2011
First published on 12th May 2011
Abstract
A recyclable copper nanopowder catalyst in ionic liquid is effective for promotion of the cross-coupling reaction of various thiols with aryl iodides under ligand-free conditions. Compared to the usual organic solvents, [bmmim]BF4 exhibited higher performance with the advantage of being able to be reused for up to four successive runs.
The formation of C–N, C–O, and C–S by transition-metal-catalyzed cross-coupling reactions of aryl halides with nitrogen, oxygen, and sulfur nucleophiles is a powerful tool in organic synthesis.1 For the past decade aryl sulfides have been important intermediates in several organic transformations and this class of compounds and their derivatives have been found in numerous biological and pharmaceutically active compounds.2 Nevertheless, an integral part of numerous drugs in therapeutic areas such as diabetes, inflammation, Alzheimer's and Parkinson's disease,3 cancer,4 and HIV2a contain aryl sulfide as the functional group. Among the various cross-coupling reactions, S-arylation is comparatively less studied.5 There are some factors which hinder this process: first, S–S oxidative coupling reactions are more favorable, which result in the undesired formation of disulfides, and second, organic sulfur compounds can be effective metal binders, which leads to catalyst modification or deactivation.6 Although several transition metals have been used to perform C–S cross-coupling such as palladium,7nickel,8copper,9cobalt,10iron,11 and indium12 there are some limitations associated with these catalytic systems, for example, the high cost of palladium salts and phosphine ligands, metal toxicity and handling problems. Copper-catalyzed Ullmann-type C–S coupling reactions can be accelerated using certain ligands such as phosphazene,13ethylene glycol,14neocuproine,15N-methylglycine,16oxime-phosphine oxide ligand,17 tripod ligand,18benzotriazole,191,2-diaminocyclohexane,20β-ketoester,21L-proline,22 and BINAM.23 Indeed, significant progress has been achieved through the use of ligands in these processes. The most accepted explanation for this behavior is that the use of ligands may increase the solubility of copper salts in the solvents.
Very recently, copper-catalyzed reactions at high temperature without the use of a ligand have been reported.21
Additionally, organic reactions catalyzed by metallic nanostructures are currently an area of intensive research, with many reports in the literature.24 Generally, the catalysts in a nanoscale afford a more effective process and allow genuine benefits over traditional methodologies. Nanomaterials with high surface areas and reactive morphologies have been studied as effective catalysts for organic synthesis.25 In this new intensive area, CuO appears as a useful catalyst for various transformations.26 Furthermore, the nanomaterial-catalyzed reactions provide the advantages of simplified isolation of the product, and easy recovery and recyclability of the catalysts. In addition, ionic liquids have frequently been used in recent few years as alternative reaction media for a broad range of chemical transformations. They offer various interesting properties in terms of alleviating environmental hazards such as no effective vapor pressure, nonvolatility, nonflammability, excellent chemical and thermal stability, and recyclability, which make them attractive media for organic reactions.27
In this context, and in connection with our ongoing interest in the synthesis of organochalcogen derivatives and their application in biological and asymmetric transformations,28 herein we report the combination of nanotechnology and ionic liquids to perform C–S cross-coupling with CuO nanopowder as a catalyst in [bmmim]BF4 in excellent yields. This efficient and ligand-free methodology has the advantages of high atom efficiency, simplified isolation of the product, and easy recovery and recyclability of the catalysts and solvent, as depicted in Scheme 1.
 |
| Scheme 1 | |
In order to optimize the protocol and to understand the influence of different variables on this reaction, several components were studied to increase its efficiency. To this aim, we carried out the reaction employing 4-methoxy iodobenzene and phenyl thiol as a model reaction. In the first set of experiments we used 10 mol% of CuO nanopowder and 2.0 equiv. of Cs2CO3 at 110 °C for 2 hours, and four different ionic liquids were evaluated (Table 1). In all of these experiments the desired product was obtained, with yields ranging from 76 to 99% showing [bmmim]BF4 as the best solvent for this reaction (entry 4). Furthermore, when the reaction was performed in organic solvent DMSO the expected coupling product was afforded in lowest 80% yield (Table 1, entry 5).
Table 1 Optimization of solvent for the C–S cross coupling
Entry |
Ionic liquid |
Yielda (%) |
Yields for isolated pure products.
|
1 |
[bmim]BF4 |
78 |
2 |
[bmim]NTf2 |
85 |
3 |
[bmim]PF6 |
76 |
4 |
[bmmim]BF4 |
99 |
5 |
DMSO
|
80 |
Although the improved capability of ionic liquids to accelerate many organic reactions compared to other organic solvents has been extensively reported, the origin of their behavior is still an intriguing subject of study. Properties such as strong dipolar and dispersion forces, hydrogen bond acidity (related to the cationic portion), and hydrogen bond basicity (related to the anionic portion) would account for the complex–solvent interactions exhibited by ILs.27k
We can rationalize the difference between the reactivity of [bmim] and [bmmim]BF4 in terms of their Lewis acid abilities. This characteristic feature of ILs could certainly influence the coupling reaction, allowing a possible explanation regarding the differences in the reaction yield. Taking this into account, we studied the Lewis acid character of the related ILs by density functional theory calculations. According to the hard and soft acids and bases (HSAB) principle,29 hard (soft) Lewis acids preferentially interact with hard (soft) Lewis bases. The global hardness (η) and global softness (S) of a given molecule can be determined through total energy calculations as30
where
I and
A represent the vertical ionization potential and electronic affinity of the molecule. The local counterparts of these quantities, called local hardness,
η(
r), and softness,
s(
r), allow one to determine the electrophilic and/or the nucleophilic centers of each molecule participating in a given chemical reaction.
31
It can be shown that the electrophilic, s′+(r), and the nucleophilic, s−+(r), local softness of a given molecule can be determined through appropriate charge density differences, according to the following formulas:31
s+(r) ≅ S(ρN+1(r) − ρN(r)) |
s+(r) ≅ S(ρN(r) − ρN−1(r)) |
where
ρX(
r) represents the charge density of a system with
X electrons at point
r. It has recently been shown that both local hardness and local softness yield the same information, representing the local abundance of their global properties.
32
We have used the density functional theory and the B3LYP33 hybrid exchange and correlation functional to calculate the equilibrium geometry, and the global hardness and softness, as well as the electrophilic and nucleophilic softness, of the [bmim] and [bmmim] cations. The Ahldrichs-VDZ basis set,34 augmented with a polarization and a diffuse function (excluding the H atoms), has previously been employed, as implemented in the ORCA suite of programs.35
The calculated global hardness values for [bmim] and [bmmim] are 5.21 and 5.16 eV, respectively, thus indicating very similar behaviors. Furthermore, [bmim] and [bmmim] cations act as Lewis acids in the coupling reaction, and their most reactive sites should be evident on a map of the s+(r) function. In Fig. 1 we show the s+(r) maps for [bmim] and [bmmim] and it can be clearly seen that for [bmim] and [bmmim]BF4 the most electrophilic regions (higher values of s′+(r)) are at similar sites, indicating Lewis acid centers, as depicted in Fig. 1.
![Calculated local softness maps, s+(r), for the (a) [bmim] and (b) [bmmim] cations.](/image/article/2011/CY/c1cy00091h/c1cy00091h-f1.gif) |
| Fig. 1 Calculated local softness maps, s+(r), for the (a) [bmim] and (b) [bmmim] cations. | |
We believe that this ability of ILs to act as Lewis acids may allow these compounds to function as promoters, making the aromatic iodides more reactive in the catalytic cycle (Scheme 2). In order to understand this rationalization, we evaluated the global hardness of the representative iodobenzene. This value for iodobenzene is 2.88 eV, i.e., a soft value. Although the global hardness of [bmim] and [bmmim] are close to each other, the latter has a softer character and, hence, according to the HSAB principle, there would be a slight preference toward the interaction with soft bases, such as the aromatic iodides. Therefore, there should be a slight tendency for higher reaction yields for [bmmim] compared to [bmim], as seen.
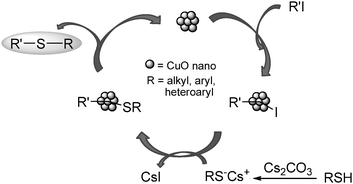 |
| Scheme 2 Plausible reaction pathway. | |
Continuing the protocol optimization, we performed another set of experiments to evaluate the influence of diverse bases and the reaction time (Table 2). In this context, a variety of inorganic (Table 2, entries 1–4) and organic bases (entries 5 and 6) were used to afford aryl sulfides. Despite that the respective sulfide could be detected employing the related bases screened, Cs2CO3 showed the best performance, furnishing the desired product in quantitative yield (entry 1). Noteworthy is that in the absence of a base no formation of product was observed (Table 2, entry 7). Cesium bases show more effectiveness to this kind of transformation, allowing the formation of the desired thiolates more efficiently. As a result, Cs2CO3 was selected as the optimum base. It was also verified that decreasing the amount of Cs2CO3 up to 1.2 equiv. had no impact on the formation of the product (entries 1 and 9, 10).
Table 2 Optimization of base, time and temperature
Entry |
Base/Equiv. |
Time/h |
Yielda (%) |
Yields for isolated products.
Reaction conducted at rt.
|
1 |
Cs2CO3 |
2.0 |
2 |
99 |
2 |
K2CO3 |
2.0 |
2 |
27 |
3 |
CsOH
|
2.0 |
2 |
85 |
4 |
KOH
|
2.0 |
2 |
34 |
5 |
Et3N
|
2.0 |
2 |
63 |
6 |
Pyridine
|
2.0 |
2 |
42 |
7 |
None |
2.0 |
2 |
0 |
8 |
Cs2CO3 |
1.0 |
2 |
86 |
9 |
Cs2CO3 |
1.2 |
2 |
99 |
10 |
Cs2CO3 |
1.5 |
2 |
99 |
11 |
Cs2CO3 |
1.2 |
1 |
81 |
12 |
Cs2CO3 |
1.2 |
0.5 |
67 |
13 |
Cs2CO3 |
1.2 |
24b |
25 |
The time required for the completion of the reaction was then evaluated. Decreasing the reaction time from 2 hours to 1 and 0.5 h afforded the product with yields of 99%, 81% and 67%, respectively (entries 9 and 11, 12). An experiment at rt was also conduced, but the desired product was obtained in only 25% yield, even after 24 hours (entry 13).
The amount of catalyst required to promote the reaction efficiently was also studied and we found that varying the amount of CuO nanopowder has an influence on the product formation. There was a slight increase in the yield from 60 to 70% when the amount of CuO nanopowder was changed from 1.0 to 2.0 mol%. No significant alteration was verified using 5.0 mol% of CuO nanopowder, affording the respective compound in 76% yield. Finally, on increasing the amount of CuO nanopowder up to 10 mol% the highest yield of the desired product was obtained in 99%, as can be observed in Table 3.
Table 3 Optimization of Nano CuO catalyst
Entry |
CuO nanopowder/mol% |
Yielda (%) |
Yields for isolated products.
|
1 |
10.0 |
99 |
2 |
5.0 |
76 |
3 |
2.0 |
70 |
4 |
1.0 |
60 |
Using the optimized conditions, the present reaction was further expanded to a broader range of aryl thiols and aryl iodides in order to evaluate the scope and limitations of the method, as outlined in Table 4. In general, the reactions are very clean and high yielding. No ligand has been used.
Table 4
CuO-catalyzed S-arylation of aryl iodides with aryl thiols
Entry |
R |
ArI
|
t/h |
Product |
Yielda (%) |
Yields for isolated products.
|
1 |
|
|
2 |
|
99 |
2 |
|
|
4 |
|
99 |
3 |
|
|
4 |
|
99 |
4 |
|
|
2 |
|
99 |
5 |
|
|
4 |
|
97 |
6 |
|
|
3 |
|
96 |
7 |
|
|
3 |
|
99 |
8 |
|
|
2 |
|
99 |
9 |
|
|
3 |
|
99 |
10 |
|
|
3 |
|
80 |
11 |
|
|
3 |
|
99 |
12 |
|
|
4 |
|
76 |
13 |
|
|
8 |
|
80 |
14 |
|
|
4 |
|
70 |
15 |
|
|
5 |
|
53 |
Initially, a set of reactions was performed with phenyl thiol and different aryl iodides (entries 1–7). In most examples quantitative yields were achieved, except for 2-iodoaniline and 3-iodopyridine, which produced the desired sulfides in 97 and 96% yields, respectively (entries 5 and 6). The formation of the products seems not to be affected by steric and electronic effects in the aryl moiety, since in both cases the products were formed with the same level of efficiency (entries 1 vs. 4 and 1 vs. 3). Full selectivity toward the iodo derivative was found (entry 3), leading to 4-bromophenyl phenyl thioether as the only product through a clean and selective reaction. In another set of experiments we promoted the coupling using a variety of sulfides and, once again, the products were formed with high levels of efficiency (entries 8–13). Even alkyl sulfides (entries 11 and 12) successfully underwent C–S coupling. To check the scope of our methodology, we next subjected the developed protocol to a more complex system. Biologically active sulfides, namely captropil (entry 14) and N-Boc protected L-penicillanime (entry 15), were employed as starting materials for the coupling with 4-methoxy iodobenzene under standard conditions. In both examples the products were obtained in good yields, 70 and 53%, respectively, showing the versatility of the methodology in the presence of more complexes functionalities. Nevertheless, this coupling was not addressed by earlier methods. Furthermore, our approach allows the preparation of the desired products in a shorter time. Although in the other related procedures with CuO the products were successfully obtained,5a,f,h,l,m,11a,26a these protocols require long reaction time under higher temperature.
The development of economic and environmentally friendly methods is one of our prime concerns. This prompted us to further improve the importance of our protocol and perform an evaluation of the recyclability of the ionic liquid and CuO catalyst employed in our reactions. After the work-up, the recovered [bmmim]BF4 was reused for further reactions and found to have comparable efficiency for up to 4 recycling experiments, as shown in Fig. 2. The CuO nanopowder was also recovered from the reaction mixture. It was reused for three further runs and no loss of activity was observed, providing the product in very good yields (Fig. 2).
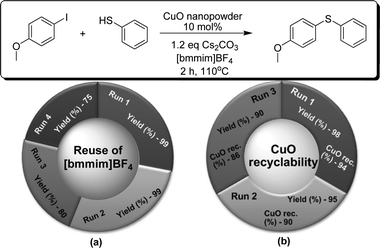 |
| Fig. 2 Recyclability tests: (a) reuse of IL; (b) CuO recyclability. | |
Based on previous plausible mechanisms, a reaction pathway for CuO nanoparticle-catalyzed C–S cross-coupling of aryl iodides and phenyl thiol is proposed in Scheme 2.
In conclusion, we have developed a clean, inexpensive and efficient methodology to obtain diaryl or alkyl aryl sulfides from aryl iodides and thiols using CuO nanopowder in ionic liquid as a reusable medium. Reactivity indexes associated with the reaction catalysts have been calculated, suggesting higher reaction yields for [bmmim]BF4. The features of this method include the following: (i) use of easily accessible alkylating agents; (ii) use of a small amount of catalyst; (iii) good to excellent yields were obtained in a short time, comparing to other protocols described in the literature; (iv) effective for complex functionalities; (v) the solvent/ionic liquid and the catalyst can be easily recovered and reused.
Acknowledgements
The authors gratefully acknowledge CNPq, INCT-Catalise, INCT-NANOBIOSIMES and FAPESC for financial support and Centro de Supercomputação, CESUP/Porto Alegre, for the calculations. Devender Singh thanks TWAS-CNPq for a PhD fellowship.
Notes and references
-
(a) A. R. Muci and S. L. Buchwald, Top. Curr. Chem., 2002, 219, 131;
(b)
Metal-Catalyzed Cross-Coupling Reactions, ed. F. Diederich and A. de Meijere, Wiley-VCH, Weinheim, 2004 Search PubMed;
(c) J. F. Hartwig, Synlett, 2006, 1283 CrossRef CAS.
-
(a) S. W. Kaldor, V. J. Kalish, J. F. Davies II, B. V. Shetty, J. E. Fritz, K. Appelt, J. A. Burgess, K. M. Campanale, N. Y. Chirgadze, D. K. Clawson, B. A. Dressman, S. D. Hatch, D. A. Khalil, M. B. Kosa, P. P. Lubbehusen, M. A. Muesing, A. K. Patick, S. H. Reich, K. S. Su and J. H. Tatlock, J. Med. Chem., 1997, 40, 3979 CrossRef CAS;
(b) L. Liu, J. E. Stelmach, S. R. Natarajan, M.-H. Chen, S. B. Singh, C. D. Schwartz, C. E. Fitzgerald, S. J. O'Keefe, D. M. Zaller, D. M. Schmatz and J. B. Doherty, Bioorg. Med. Chem. Lett., 2003, 13, 3979 CrossRef CAS.
-
(a) S. F. Nielsen, E. Ø. Nielsen, G. M. Olsen, T. Liljefors and D. Peters, J. Med. Chem., 2000, 43, 2217 CrossRef CAS;
(b) G. Liu, J. R. Huth, E. T. Olejniczak, F. Mendoza, S. W. Fesik and T. W. von Geldern, J. Med. Chem., 2001, 44, 1202 CrossRef CAS.
- G. De Martino, M. C. Edler, G. La Regina, A. Cosuccia, M. C. Barbera, D. Barrow, R. I. Nicholson, G. Chiosis, A. Brancale, E. Hamel, M. Artico and R. Silvestri, J. Med. Chem., 2006, 49, 947 CrossRef CAS.
- For some S-arylations of thiols, see
(a) F. Y. Kwong and S. L. Buchwald, Org. Lett., 2002, 4, 3517 CrossRef CAS;
(b) A. K. Verma, J. Singh and R. Chaudhary, Tetrahedron Lett., 2007, 48, 7199 CrossRef CAS;
(c) M. Carril, R. SanMartin, E. Domínguez and I. Tellitu, Chem.–Eur. J., 2007, 13, 5100 CrossRef CAS;
(d) Y. Zhang, K. C. Ngeow and J. Y. Ying, Org. Lett., 2007, 9, 3495 CrossRef CAS;
(e) L. Rout, T. Sen and T. Punniyamurthy, Angew. Chem., Int. Ed., 2007, 46, 5583 CrossRef CAS;
(f) B. C. Ranu, A. Saha and R. Jana, Adv. Synth. Catal., 2007, 349, 2690 CrossRef CAS;
(g) E. Sperotto, G. P. M. van Klink, J. G. de Vries and G. van Koten, J. Org. Chem., 2008, 73, 5625 CrossRef CAS;
(h) Z. Jiang, J. She and X. Lin, Adv. Synth. Catal., 2009, 351, 2558 CrossRef CAS;
(i) J. She, Z. Jiang and Y. Wang, Tetrahedron Lett., 2009, 50, 593 CrossRef CAS;
(j) Y. Jiang, Y. Qin, S. Xie, X. Zhang, J. Dong and D. Ma, Org. Lett., 2009, 11, 5250 CrossRef CAS;
(k) S. Bhadra, B. Sreedhar and B. Ranu, Adv. Synth. Catal., 2009, 351, 2369 CrossRef CAS;
(l) S. N. Murthy, B. Madhav, V. P. Reddy and Y. V. D. Nageswar, Eur. J. Org. Chem., 2009, 5902 CrossRef CAS;
(m) C.-K. Chen, Y.-W. Chen, C.-H. Lin, H.-P. Lin and C.-F. Lee, Chem. Commun., 2010, 46, 282 RSC.
- For a review dealing with the metal-catalyzed formation of carbon–sulfur bonds, see T. Kondo and T. Mitsudo, Chem. Rev., 2000, 100, 3205 Search PubMed.
-
(a) T. Ishiyama, M. Mori, A. Suzuki and N. Miyaura, J. Organomet. Chem., 1996, 525, 225 CrossRef CAS;
(b) G. Y. Li, Angew. Chem., Int. Ed., 2001, 40, 1513 CrossRef CAS;
(c) M. Murata and S. L. Buchwald, Tetrahedron, 2004, 60, 7397 CrossRef CAS;
(d) T. Itoh and T. Mase, Org. Lett., 2004, 6, 4587 CrossRef CAS;
(e) M. A. Fernández-Rodríguez, Q. Shen and J. F. Hartwig, Chem.–Eur. J., 2006, 12, 7782 CrossRef CAS;
(f) M. A. Fernández-Rodríguez, Q. Shen and J. F. Hartwig, J. Am. Chem. Soc., 2006, 128, 2180 CrossRef CAS.
-
(a) Y. Zhang, K. N. Ngeow and J. Y. Ying, Org. Lett., 2007, 9, 3495 CrossRef CAS;
(b) S. Jammi, P. Barua, L. Rout, P. Saha and T. Punniyamurthy, Tetrahedron Lett., 2008, 49, 1484 CrossRef CAS;
(c) Y. Q. Cao, Z. Zhang, Y. X. Guo and G. Q. Wu, Synth. Commun., 2008, 38(9), 1325 CrossRef CAS.
- For reviews:
(a) S. V. Ley and A. W. Thomas, Angew. Chem., Int. Ed., 2003, 42, 5400 CrossRef CAS;
(b) I. P. Beletskaya and A. V. Cheprakov, Coord. Chem. Rev., 2004, 248, 2337 CrossRef CAS.
- Y. C. Wong, T. T. Jayanth and C. H. Cheng, Org. Lett., 2006, 8, 5613 CrossRef.
-
(a) A. Correa, M. Carril and C. Bolm, Angew. Chem., Int. Ed., 2008, 47, 2880 CrossRef;
(b) W. Y. Wu, J. C. Wang and F. Y. Tsai, Green Chem., 2009, 11, 326 RSC.
-
(a) V. P. Reddy, K. Swapna, A. V. Kumar and K. R. Rao, J. Org. Chem., 2009, 74, 3189 CrossRef CAS;
(b) V. P. Reddy, A. V. Kumar, K. Swapna and K. R. Rao, Org. Lett., 2009, 11, 1697 CrossRef CAS.
- C. Palomo, M. Oiarbide, R. Lopez and E. Gomez-Bengoa, Tetrahedron Lett., 2000, 41, 1283 CrossRef CAS.
-
(a) F. Y. Kwong and S. L. Buchwald, Org. Lett., 2002, 4, 3517 CrossRef CAS;
(b) C. Enguehard- Gueiffier, I. Thery, A. Gueiffier and S. L. Buchwald, Tetrahedron, 2006, 62, 6042 CrossRef CAS.
- C. G. Bates, R. K. Gujadhur and D. Venkataraman, Org. Lett., 2002, 4, 2803 CrossRef CAS.
- W. Deng, Y. Zou, Y. F. Wang, L. Liu and Q. X. Guo, Synlett, 2004, 1254 CAS.
- D. Zhu, L. Xu, F. Wu and B. Wan, Tetrahedron Lett., 2006, 47, 5781 CrossRef CAS.
- Y.-J. Chen and H.-H. Chen, Org. Lett., 2006, 8, 5609 CrossRef CAS.
- A. K. Verma, J. Singh and R. Chaudhary, Tetrahedron Lett., 2007, 48, 7199 CrossRef CAS.
-
(a) M. Carril, R. SanMartin, E. Dominguez and I. Tellitu, Chem.–Eur. J., 2007, 13, 5100 CrossRef CAS;
(b) M. T. Herrero, R. SanMartin and E. Dominguez, Tetrahedron, 2009, 65, 1500 CrossRef CAS.
-
(a) X. Lv and W. Bao, J. Org. Chem., 2007, 72, 3863 CrossRef CAS;
(b) H. Xu, X. Zhao, J. Deng, Y. Fu and Y. Feng, Tetrahedron Lett., 2009, 50, 434 CrossRef CAS.
- H. Zhang, W. Cao and D. Ma, Synth. Commun., 2007, 37, 25 CrossRef CAS.
- D. J. C. Prasad, A. B. Naidu and G. Sekar, Tetrahedron Lett., 2009, 50, 1411 CrossRef CAS.
-
(a) M. Shibasaki and M. Kanai, Chem. Rev., 2008, 108, 2853 CrossRef CAS;
(b) M. Meldal and C. W. Tornøe, Chem. Rev., 2008, 108, 2952 CrossRef CAS;
(c) V. P. Reddy, A. V. Kumar, K. Swapna and K. R. Rao, Org. Lett., 2009, 11, 951 CrossRef CAS;
(d) V. Polshettiwar, B. Baruwati and R. S. Varma, Chem. Commun., 2009, 1837 RSC;
(e) V. Polshettiwar, B. Baruwati and R. S. Varma, Green Chem., 2009, 11, 127 RSC;
(f) A. Saha, D. Saha and B. C. Ranu, Org. Biomol. Chem., 2009, 7, 1652 RSC;
(g) S. Jammi, S. Sakthivel, L. Rout, T. Mukherjee, S. Mandal, R. Mitra, P. Saha and T. Punniyamurthy, J. Org. Chem., 2009, 74, 1971 CrossRef CAS.
-
(a) Y. Li, X. M. Hong, D. M. Collard and M. A. El-Sayed, Org. Lett., 2000, 2, 2385 CrossRef CAS;
(b) H. Bönnemann and R. M. Richards, Eur. J. Inorg. Chem., 2001, 2455 CrossRef CAS;
(c) M. Tamura and H. Fujihara, J. Am. Chem. Soc., 2003, 125, 15742 CrossRef CAS;
(d) Y. Uozumi and R. Nakao, Angew. Chem., Int. Ed., 2003, 42, 194 CrossRef CAS;
(e) M.-K. Chung and M. Schlaf, J. Am. Chem. Soc., 2004, 126, 7386 CrossRef CAS;
(f) C. C. Cassol, A. P. Umpierre, G. Machado, S. I. Wolke and J. Dupont, J. Am. Chem. Soc., 2005, 127, 3298 CrossRef CAS;
(g) L. D. Pachón, M. B. Thathagar, F. Hartl and G. Rothenberg, Phys. Chem. Chem. Phys., 2006, 8, 151 RSC;
(h) Y. Zhu, S. C. Peng, A. Emi, S. Zhenshun, Monalisa and R. A. Kemp, Adv. Synth. Catal., 2007, 349, 1917 CrossRef;
(i) B.-X. Tang, F. Wang, J.-H. Li, Y.-X. Xie and M.-B. Zhang, J. Org. Chem., 2007, 72, 6294 CrossRef CAS;
(j) F.-Z. Su, Y.-M. Liu, L.-C. Wang, Y. Cao, H.-Y. He and K.-N. Fan, Angew. Chem., Int. Ed., 2008, 47, 334 CrossRef CAS.
-
(a) L. Rout, T. K. Sen and T. Punniyamurthy, Angew. Chem., Int. Ed., 2007, 46, 5583 CrossRef CAS;
(b) L. Rout, S. Jammi and T. Punniyamurthy, Org. Lett., 2007, 9(17), 3397 CrossRef CAS;
(c) D. Singh, E. E. Alberto, O. E. D. Rodrigues and A. L. Braga, Green Chem., 2009, 11, 1521 RSC;
(d) V. P. Reddy, A. V. Kumar, K. Swapna and K. R. Rao, Org. Lett., 2009, 11, 951 CrossRef CAS.
-
(a) J. D. Holbrey and K. R. Seddon, Clean Prod. Processes, 1999, 1, 233;
(b) K. R. Seddon, J. Chem. Technol. Biotechnol., 1997, 68, 351 CrossRef CAS;
(c) T. Welton, Chem. Rev., 1999, 99, 2071 CrossRef CAS;
(d) P. Wasserscheid and W. Keim, Angew. Chem., Int. Ed., 2000, 39, 3772 CrossRef;
(e) R. Sheldon, Chem. Commun., 2001, 2399 RSC;
(f) For a comprehensive review about ionic liquids see: J. Dupont, R. F. Souza and P. A. Z. Suarez, Chem. Rev., 2002, 102, 3667 Search PubMed;
(g) C. C. Cassol, G. Ebeling, B. Ferrera and J. Dupont, Adv. Synth. Catal., 2006, 348, 243 CrossRef CAS;
(h) J. Ranke, S. Stolte, R. Störmann, J. Arning and B. Jastorff, Chem. Rev., 2007, 107, 2183 CrossRef CAS;
(i) P. Hapiot and C. Lagrost, Chem. Rev., 2008, 108, 2238 CrossRef CAS;
(j) M. A. P. Martins, C. P. Frizzo, D. N. Moreira, N. Zanatta and H. G. Bonacorso, Chem. Rev., 2008, 108, 2015 CrossRef CAS;
(k) C. M. Gordon, Appl. Catal., A, 2001, 222, 101 CrossRef CAS.
- For selected examples see:
(a) A. L. Braga, D. S. Lüdtke, M. W. Paixão and O. E. D. Rodrigues, Org. Lett., 2003, 5, 2635 CrossRef CAS;
(b) A. L. Braga, D. S. Lüdtke, E. E. Alberto and J. A. Sehnem, Tetrahedron, 2005, 61, 11664 CrossRef CAS;
(c) A. L. Braga, J. A. Sehnem, F. Vargas and R. C. Braga, J. Org. Chem., 2005, 70, 9021 CrossRef CAS;
(d) A. L. Braga, D. S. Lüdtke, M. W. Paixão, E. E. Alberto, H. A. Stefani and L. Juliano, Eur. J. Org. Chem., 2005, 4260 CrossRef CAS;
(e) A. L. Braga, D. S. Lüdtke and E. E. Alberto, J. Braz. Chem. Soc., 2006, 17, 11 CAS;
(f) R. S. Schwab, F. Z. Galetto, J. B. Azeredo, A. L. Braga, D. S. Lüdtke and M. W. Paixão, Tetrahedron Lett., 2008, 49, 5094 CrossRef CAS;
(g) A. L. Braga, E. E. Alberto, L. C. Soares, J. B. T. Rocha, J. H. Sudati and D. H. Ross, Org. Biomol. Chem., 2009, 7, 43 RSC;
(h) A. L. Braga, W. A. S. Filho, R. S. Schwab, O. E. D. Rodrigues, L. Dornelles, H. C. Braga and D. S. Lüdtke, Tetrahedron Lett., 2009, 50, 3005 CrossRef CAS;
(i) E. E. Alberto, L. C. Soares, J. H. Sudati, A. C. A. Borges, J. B. T. Rocha and A. L. Braga, Eur. J. Org. Chem., 2009, 4211 CrossRef CAS;
(j) D. B. Santos, V. P. P. Schiar, M. C. P. Ribeiro, R. S. Schwab, D. F. Meinerz, J. Allebrandt, J. B. T. Rocha, C. W. Nogueira, M. Aschner and N. B. V. Barbosa, Mutat. Res., Genet. Toxicol. Environ. Mutagen., 2009, 676, 21 CrossRef CAS;
(k) R. S. Schwab, L. C. Soares, L. Dornelles, O. E. D. Rodrigues, M. W. Paixão, M. Godoi and A. L. Braga, Eur. J. Org. Chem., 2010, 3574 CrossRef CAS.
-
(a) R. G. Pearson, J. Am. Chem. Soc., 1963, 85, 3533 CrossRef CAS;
(b) R. G. Pearson, Science, 1966, 151, 172;
(c)
R.G. Pearson, Chemical Hardness: Applications from Molecules to Solids, Wiley-VCH, Oxford, 1997 Search PubMed.
- R. G. Parr and R. G. Pearson, J. Am. Chem. Soc., 1983, 105, 7512 CrossRef CAS.
-
(a) W. T. Yang and R. G. Parr, Proc. Natl. Acad. Sci. U. S. A., 1985, 82, 6723 CAS;
(b) M. Berkowitz, J. Chem. Phys., 1985, 83, 2976 CrossRef CAS;
(c)
R. G. Parr and W. Yang, Density-Functional Theory of Atoms and Molecules, Oxford University Press, New York, 1989 Search PubMed.
- M. Torrent-Sucarrat, F. De Proft, P. W. Ayres and P. Geerlings, Phys. Chem. Chem. Phys., 2010, 12, 1072 RSC.
-
(a) A. D. Becke, J. Chem. Phys., 1993, 98, 5648 CrossRef CAS;
(b) C. T. Lee, W. T. Yang and R. G. Parr, Phys. Rev. B, 1988, 37, 785 CrossRef CAS.
- A. Schaefer, H. Horn and R. Ahlrichs, J. Chem. Phys., 1992, 97, 2571 CrossRef.
- F. Neese, J. Chem. Phys., 2001, 115, 11080 CrossRef CAS.
Footnote |
† Electronic supplementary information (ESI) available: Synthetic procedures and compound characterization data. See DOI: 10.1039/c1cy00091h |
|
This journal is © The Royal Society of Chemistry 2011 |
Click here to see how this site uses Cookies. View our privacy policy here.