DOI:
10.1039/C0PY00194E
(Paper)
Polym. Chem., 2010,
1, 1644-1649
Synthesis and fluorescence properties of hyperbranched poly(amidoamine)s with high density tertiary nitrogen
Received
28th June 2010
, Accepted 27th July 2010
First published on
21st September 2010
Abstract
Hyperbranched poly(amidoamine)s with high density tertiary nitrogen (HPAMAMs-OH) were prepared via the two-step reactions. Firstly, hyperbranched poly(amidoamine)s (HPAMAMs) were synthesized by Michael addition of 1-(2-aminoethyl) piperazine (AEPZ) and methyl acrylate (MA) and then alkylation of the residual amino protons of hyperbranched poly(amidoamine)s (HPAMAMs) with propylene oxide (PO) in ethanol was performed. The fluorescence properties of HPAMAMs and HPAMAMs-OH were investigated. It was found that the fluorescence intensity of the polymers increased correspondingly with the increasing of the tertiary nitrogen in the molecular structure. The tertiary nitrogen with lone pair electrons inside the molecular structure played an important role in the photoluminescence. The complex with nano gold encapsulated in the interior of the HPAMAMs-OH presented greatly enhanced emission. Those results show that the nano gold may be used to adjust the fluorescence properties of HPAMAMs-OH and may extend potential applications of HPAMAMs-OH and gold nanoparticles.
1. Introduction
A vital challenge in cell biology and biochemistry is to decipher cellular structures, behaviors, and physiological functions through visual methods involving minimal perturbation to the biological system.1 During the past decades, fluorescent tagging has been proven to be a powerful implement for cell imaging or tracking the drug delivery process.2–4 Because small fluorophores and fluorescent proteins suffer from low photo bleaching thresholds that limit their effectiveness in long-term and three-dimensional imaging,1,5 synthesized photo luminescent polymers have emerged as a category of bright and photo stable alternatives. Among these photo luminescent polymers, water-soluble fluorescent polymers are particularly useful tracers for their acting as analogs in understanding the behavior of biological macromolecules.6,7 In general, there are two ways to synthesize such fluorescent polymers. One of them is the polymerization of polymerizable fluorescent monomers with some common monomers,8,9 the other is the chemical modification of polymers by fluorescent molecules or fluorescent oligomers.10,11 However, the synthesis of these fluorescent polymers requires multiple steps and it is cumbersome to synthesize and purify these polymers.
Hyperbranched polymers are three-dimensional macromolecules with good solubility and high reactivity. They are usually prepared by one-pot synthesis from specific monomers with branched potential.12–14 If they could fluoresce strongly, this would simplify the synthesis process and widen application areas for these polymers. The weak fluorescence of PAMAM dendrimers was first found by Larson and Tucker in 2001.15 It is reported that tertiary amines in the main chain of PAMAM dendrimers may have an important impact on the fluorescence property.16,17 Disulfide-functionalized hyperbranched poly(amidoamine)s were reported by Pan's group,18 the novel hyperbranched polymers displayed bright fluorescence, and the emission bands cover nearly the whole visible wavelength range. We have reported that hyperbranched poly(amidoamine)s, synthesized by Michael addition of 1-(2-aminoethyl) piperazine (AEPZ) and methyl acrylate (MA), can emit bright blue fluorescence intensity under excitation wavelength.19 Until now, several kinds of dendritic polymers and even linear polymers with a large amount of amino groups have been claimed to emit fluorescence strongly.20–22 Despite these attempts, the relatively weak fluorescence of hyperbranched polymers is still an obstacle for their practical application.
Increasing the tertiary nitrogen content of the molecular structure is a visible option for improving the fluorescence intensity of polymers. Wan et al.23–25 reported that the amino protons of hyperbranched polyethylenimine (HPEI) can be alkylated with 2-dodecyloxymethyloxirane and complete alkylation of the residual amino protons with propylene oxide (PO), leading to high density tertiary nitrogen in molecular structure. On the other hand, lots of works have concentrated on nano gold, such as GNPs and GNDs or nanoclusters and their applications in many different fields,26–28 such as biological detection, biomarkers, molecular recognition, catalysis, chemical sensors, nanoelectrodes and so on.29,30 Among these increased works, some literature reported that the composite of dendrimers with encapsulated nanoparticles can enhance their fluorescent emission,31 which can be utilized as a basis for ultrasensitive analytical techniques in biology and medicine.32,33
In this research, hyperbranched poly(amidoamine)s with high density tertiary nitrogen (HPAMAMs-OH) were synthesized by alkylation of the residual amino protons of hyperbranched poly(amidoamine)s (HPAMAMs) with propylene oxide (PO) in ethanol. The fluorescence properties of HPAMAMs and HPAMAMs-OH were investigated. It was found that the tertiary nitrogen with lone pair electrons inside the molecular structure played an important role in the photoluminescence. The complex with gold nanodots (GNDs) encapsulated in the interior of the HPAMAMs-OH presented greatly enhanced emission. Those results show that nano gold may be used to adjust the fluorescence properties of HPAMAMs-OH and may extend potential applications of HPAMAMs-OH and gold nanoparticles.
2. Experimental section
2.1 Materials
1-(2-Aminoethyl) piperazine (AEPZ) and methyl acrylate (MA) were purchased from Sigma-Aldrich and used as received. Propylene oxide (PO) was purchased from East-China Chemical Co. and used as received. All reagents and solvents of analytical grade are purchased from commercial suppliers and used without further purification unless stated otherwise.
2.2 Synthesis of HPAMAMs-OH
1 g of HPAMAMs (6.5 × 10−5 mol) in ethanol (5 mL) was mixed with propylene oxide (0.5 g, 7.8 mmol) and stirred at room temperature for 2 days. The volatile components were removed on a rotary evaporator at reduced pressure to yield a colorless viscous solid.
2.3 Preparation of nano gold
To synthesize nano gold, appropriate amounts of HPAMAMs-OH and hydrochloroauric acid were dissolved in distilled water and mixed magnetically. Au3+ ions can enter into the interior of the HPAMAMs-OH and form coordination binding between unoccupied orbital of Au3+ and lone pair electrons of N atom.34 Then Au3+ ions sequestered into the HPAMAMs-OH were reduced by slowly adding an equivalent of fresh NaBH4 into the solution. The solution was magnetically stirred for 2 days in the dark until reaction and aggregation processes were completed. Reduced gold atoms aggregated to form small nano gold in the interior of the HPAMAMs-OH, while some large gold nanoparticles formed outside of the HPAMAMs-OH. The resulting solution was subsequently purified through centrifugation to remove the large gold nanoparticles, leaving a clear, colorless, dendrimer encapsulated nano gold solution.
2.4 Measurement
UV/vis spectra were recorded on a 760 CRT UV/vis spectrometer (Shanghai Analytical Instrument Factory). 1H NMR spectra were recorded on Bruker (600 MHz), with TMS as reference. Fluorescence measurements were performed on a LS55 luminescence spectrometer (Perkin Elmer, America) using a 10 mm path quartz cell. Unless additional emphasis, excitation and emission slit widths were set to 10 nm and 5nm, respectively. The images of transmission electron microscopy (TEM) were obtained by a JEOL-2100 microscope. Ultrasonic mix was achieved by SK1200H (Shanghai KuDos Ultrasonic Instrument Co., Ltd.). The gel permeation chromatography (GPC) measurements were carried out on a Waters 201 with a μ-styragel column and H2O as an eluent, and the molecular weight was calibrated with standard polyethylene glycol (PEG).
3. Results and discussion
3.1 Synthesis of HPAMAMs-OH
The synthesis of HPAMAMs with high density tertiary nitrogen is outlined in Scheme 1. HPAMAMs were firstly synthesized by Michael addition of AEPZ and MA and then alkylation of the residual amino protons of HPAMAMs with PO in ethanol to obtain HPAMAMs-OH. Adjusting the reaction ratio of HPAMAMs and PO, HPAMAMs-OH with different tertiary nitrogen contents can be collected. The molecular weight (Mn) and molecular weight distribution (Mw/Mn) of A–C are also obtained by GPC measurement, and the data are collected in Table 1. The Mn of HPAMAMs is 1.54 × 104 g mol−1. After alkylation with PO, the Mn of products are 1.65 × 104 g mol−1 for B and 2.08 × 105 g mol−1 for C, respectively.
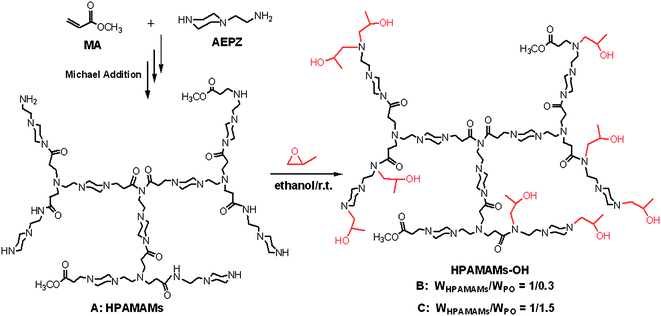 |
| Scheme 1 An outline of the synthesis of hyperbranched poly(amidoamine)s with high density tertiary nitrogen (HPAMAMs-OH). | |
Table 1 Molecular structures of A, B and Ca
Samples |
W
HPAMAMs/WPO |
M
n
|
PDI (Mw/Mn)b |
Yield %c |
DF %d |
Reaction at room temperature for 2 days for sample B and C.
Determined by GPC in H2O at 25 °C.
Yield % = (WHPAMAMs-OH/WHPAMAMs) × 100%.
DF % is the degree of functionalization by PO, , where IHPAMAMs and IHPAMAMs-OH are the signal intensity at 2.75–2.90 ppm in 1H NMR.
|
HPAMAMs, A |
— |
1.54 × 104 |
1.57 |
90.3 |
— |
HPAMAMs-OH, B |
1 : 0.3 |
1.65 × 104 |
1.60 |
95.6 |
78.5 |
HPAMAMs-OH, C |
1 : 1.5 |
2.08 × 104 |
1.62 |
100 |
100 |
Fig. 1 shows the 1H NMR spectra of HPAMAMs and HPAMAMs-OH. For the HPAMAMs sample, the protons neighboring primary and secondary amines appeared at 2.75–2.90 ppm. After alkylation of HPAMAMs with PO, new signal at 1.15 ppm appears, which is due to the methyl group of the ring opened propylene oxide; the signal intensities at 3.8 ppm (OH) and 3.4 ppm (CH) are also enhanced due to signal overlapping of HPAMAMs-OH with the ring opened propylene oxide. By comparing the signal intensity peaks at 2.75–2.90 ppm, the degree of functionalization (DF) by PO can be calculated. For the sample B, the signal intensity peaks at 2.75–2.90 ppm is lower than that of sample A. The DF of B is 78.5% according to the formula in Table 1. By comparing the signal intensity peaks at 1.15 ppm and 2.75–2.90 ppm in Fig. 2C, it can be derived that the residual amino protons in C have been completely alkylated after reaction of HPAMAMs with excess of PO.
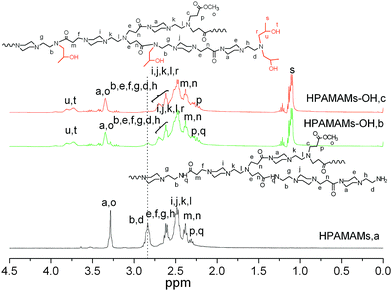 |
| Fig. 1
1H NMR spectra of HPAMAMs (A, bottom, CDCl3), and HPAMAMs-OH (B and C, upper, CDCl3). | |
3.2 Fluorescence properties of HAMAMs-OH
The UV-vis absorptions of these polymers are investigated. Fig. 2 shows the UV-vis spectra of the hyperbranched polymers HPAMAMs and HPAMAMs-OH samples in H2O solution. Polymer HPAMAMs have two absorption peaks with wavelengths λmax at 285 and 350 nm, respectively. The maximum absorption peak at 285 nm is due to the n → σ* transition of amino and imino groups. While, the weaker absorption peak at 350 nm is due to the transition of lone pair electrons inside tertiary nitrogens. After alkylation of the residual amino protons of HPAMAMs with PO, the absorption peak at 285 nm decreased with improving the degree of functionalization (DF) by PO, and the peak almost completely disappeared in sample HPAMAMs-OH (Fig. 2C). From these phenomena, it can be derived that the residual amino protons in C have been completely alkylated by PO.
Since the resultant polymers do not contain fluorescent functional groups in the classical viewpoint, then exploring the source of the fluorescence should be interesting.18,35 To further prove the fluorescence mechanism and to explore its fluorescence properties in aqueous solution, the emission and excitation spectra of HPAMAMs and HPAMAMs-OH samples were investigated without any treatment or functionalization. The aqueous solutions of hyperbranched poly(aminoester)s with concentration at 0.5 wt% similar to those dendrimers reported36,37 were prepared for photoluminescence characterization. Fig. 3 shows that HPAMAMs and HPAMAMs-OH samples have maximum excited bands at 240, 243, and 246 nm with emission bands at 420, 424, and 430 nm, respectively. Either the maximum excited bands or emission bands exhibits red-shift with increasing the content of tertiary nitrogen in the polymers. These results indicated that the hyperbranched polymer structure or the microenvironment of the functional group significantly influenced the fluorescence properties. This may result from that the tertiary amines of HPAMAMs-OH were easier to protonate in aqueous solution, and the compacted spatial morphologies became very open.35
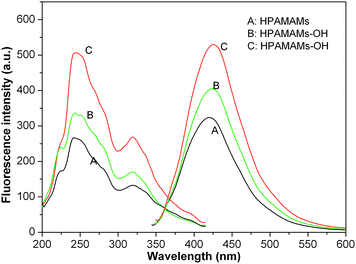 |
| Fig. 3 Emission and excitation spectra of 0.5 mM aqueous solutions of HPAMAMs (A) and HPAMAMs-OH (B and C) at pH 7 with concentration 0.5 wt%. | |
As expected, the fluorescence intensity of HPAMAMs and HPAMAMs-OH depends on the concentration. Fig. 4 shows the contradistinctive fluorescence emission intensity of HPAMAMs and HPAMAMs-OH with different concentrations. In this experiment, a series of HPAMAMs and HPAMAMs-OH solutions with the final concentrations of 0.5, 1.0, 2.0, 3.5, and 5.0 wt% were prepared and then fluorescence spectra were examined. All the emission intensities increased with increased concentrations similar to that from PAMAM.38 However, the grow rate of fluorescence emission intensities is different for three samples. It indicates that the hyperbranched polymer with high content of tertiary amines is a more efficient fluorescence agent. For more intuitive observation the fluorescence properties of obtained polymers, the illumination photographs of HAMAMs and HPAMAMs-OH aqueous solution irradiated under 365 nm are recorded with a digital camera. As shown in Fig. 5A, no obvious blue photoluminescence from HAMAMs solution can be observed when its concentration is below 5.0 wt%. However, for HPAMAMs-OH aqueous solution, stronger blue photoluminescence can be observed for all concentrations as shown in Fig. 5B. These results indicated that tertiary nitrogen with lone pair electrons inside the molecular structure plays an important role in the photoluminescence.
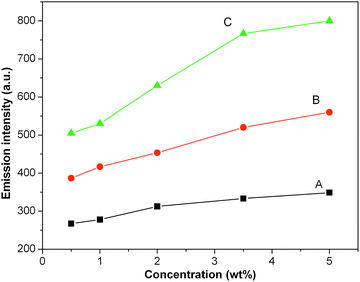 |
| Fig. 4 The contradistinctive fluorescence emission intensity of HPAMAMs (A) and HPAMAMs-OH (B and C) with the final concentrations of 0.5, 1.0, 2.0, 3.5, and 5.0 wt%. | |
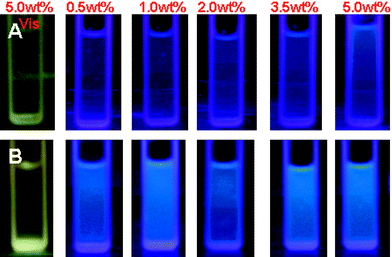 |
| Fig. 5 Illumination photographs of HAMAMs (A) and HPAMAMs-OH (B, sample C) aqueous solution irradiated under 365 nm. | |
3.3 Influence on fluorescence properties of HAMAMs-OH by nano gold
Metal nanoparticles have a variety of interesting spectroscopic, electronic, and chemical properties that arise from their small sizes and high surface-to-volume ratios.39 Therefore; nano gold may be used to adjust the fluorescence properties of fluorescence polymer and may extend potential applications of fluorescence polymer and gold nanoparticles. We investigated the enhancement of fluorescence properties of resultant polymers by nano gold. The mean diameter of nano gold used in this paper is less than 5 nm, as shown in Fig. 6. Fig. 7 shows the absorbance spectra of pure HPAMAMs-OH and HPAMAMs-OH with encapsulated nano gold, which presented strong absorption band at 285 nm for HPAMAMs with encapsulated nano gold. There is almost no plasmon band around 500–550 nm for the small particles size.
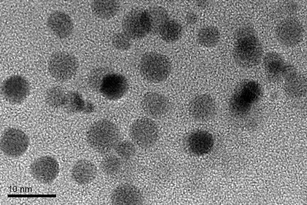 |
| Fig. 6 The morphology and size of nano gold measured by TEM. | |
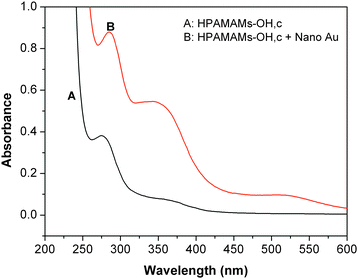 |
| Fig. 7 UV-vis absorption spectra of pure HPAMAMs-OH (A, sample C) and HPAMAMs-OH with encapsulated GNDs (B). The concentrations of HAMAMs (A and B) and nano gold were 0.25 wt% and 2.5 × 10−5 M, respectively. | |
Fig. 8 reveals that more than 1.5-fold enhancement of fluorescence intensity of HPAMAMs-OH by nano gold, while the emission peaks were red-shifted from 425 nm in pure HPAMAMs-OH to 430 nm. The fluorescence intensity increase and these spectral changes may be attributed to the interaction of gold particles with backbone of the HPAMAMs-OH, which induced the conformational arrangement of the HPAMAMs-OH.
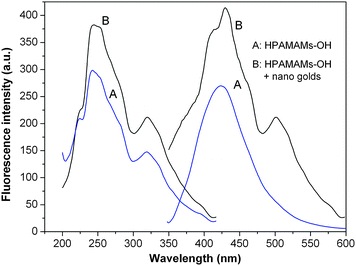 |
| Fig. 8 Fluorescence emission spectra of pure HPAMAMs-OH (A, sample C) and HPAMAMs-OH complexed with nano gold (B). The pH value of the samples was about 7. The concentrations of HAMAMs (A and B) and nano gold were 0.5 wt% and 2.5 × 10−5 M, respectively. | |
The hyperbranched polymers with a flexible nature have a non-rigid plane,40 while the existence of gold nanodots in the interior of the hyperbranched polymer make its non-rigid plane become rigid, which causes the fluorescence intensity of HPAMAMs-OH to be raised and the red shift of emission peak. The other reason for the enhancement of fluorescence intensity of HPAMAMs-OH may be on account of the nano gold. Some literature reports that fluorescence occurs only when the size of the metal nanoclusters is sufficiently small (<5 nm). The luminescence maximum moves to lower energy or disappears with increased core size, and only the smaller nanodots were observed to luminesce.41 The luminescence from gold nanodots is thought to arise from transitions between the filled d band and sp conduction bands.42–44 Murray's group reported that for 451 nm excitation, gold clusters with 1.8 nm diameter cores and protected by monolayer of tiopronin thiolate can produce luminescence.45 In this study, the diameter of nano gold is around 5 nm, which may generate fluorescence and enhance the fluorescence intensity of the complex associated with interband transitions between the filled 5d10 band and 6(sp)1 conduction band, as shown in Scheme 2. Because both components (HPAMAMs-OH and nano gold) have a good biocompatibility, the nano-materials composed of hyperbranched poly(amidoamine)s and nano gold will benefit practical applications in biological analysis and detection.
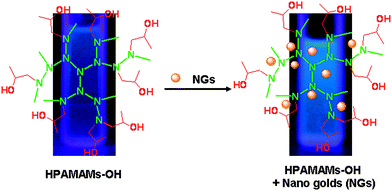 |
| Scheme 2 The structural representation of HPAMAMs-OH encapsulated with nano gold (NG). | |
4. Conclusion
Hyperbranched poly(amidoamine)s with high density tertiary nitrogen (HPAMAMs-OH) were prepared by alkylation of the residual amino protons of the HPAMAMs in ethanol with PO. The fluorescence properties of HPAMAMs and HPAMAMs-OH were investigated. Their fluorescence intensity increased correspondingly with the increase of the tertiary nitrogen in the molecular structure. The tertiary nitrogen with lone pair electrons inside the molecular structure played an important role in the photoluminescence. Because two components (HPAMAMs-OH and nano gold) have a good biocompatibility, and the complex with nano gold encapsulated in the interior of the HPAMAMs-OH provides better stability as a composite photoluminescence nano-material.
Acknowledgements
This work was financially supported by the National Natural Science Foundation of China (20604024), Natural Science Foundation of Zhejiang Province (Y406008, Y4100045), Qianjiang Talents Project of Zhejiang Province (2010R10023), Scientific Research Foundation for the Returned Overseas Chinese Scholars, State Education Ministry (1001603-C), Preferentially Financing projects of scientific and technological activities of overseas students in Zhejiang (0903677-M), Scientific Research Foundation of Zhejiang Sci-Tech University (0901808-Y) and Program for Changjiang Scholars and Innovative Research Team in University (PCSIRT: 0654).
References
- Kan-Yi Pu, Kai Li, Jianbing Shi and Bin Liu, Chem. Mater., 2009, 21, 3816–3822 CrossRef CAS.
- S. A. Kushon, K. D. Ley, K. Bradford, R. M. Jones, D. McBranch and D. Whitten, Langmuir, 2002, 18, 7245–7249 CrossRef CAS.
- H. Gao, C. Wang, W. Yang and S. Fu, J. Macromol. Sci., Part A: Pure Appl. Chem., 2004, 41, 357–371 Search PubMed.
- P. Wang, X. Wang, K. Meng, S. Hong, X. Liu, H. Cheng and C. C. Han, J. Polym. Sci., Part A: Polym. Chem., 2008, 46, 3424–3428 CrossRef CAS.
- J. W. Lichtman and J. A. Conchello, Nat. Methods, 2005, 2, 910–919 CrossRef CAS.
- N. J. Turro and K. S. Arora, Polymer, 1986, 27, 783–796 CrossRef CAS.
- C. Xue, S. P. Jog, P. Murthy and H. Liu, Biomacromolecules, 2006, 7, 2470–2474 CrossRef CAS.
- L. F. Campo, F. S. Rodembusch and V. Stefani, J. Appl. Polym. Sci., 2006, 99, 2109–2116 CrossRef CAS.
- Q. Xu, N. Li, F. Yan, X. Xia, J. Lu and X. Wen, Eur. Polym. J., 2008, 44, 1874–1880 CrossRef CAS.
- S. Saffarian, Y. Li, E. L. Elson and J. Pike, Biophys. J., 2007, 93, 1021–1031 CrossRef CAS.
- O. Valicka, I. Gryczynski, J. Fang and J. R. Lakowicz, Anal. Biochem., 2003, 317, 136–146 CrossRef.
- G. Jiang, L. Wang and W. Chen, Eur. Polym. J., 2006, 42, 3333–3340 CrossRef CAS.
- G. Jiang, L. Wang, H. Yu, C. Chen, X. Dong, T. Chen and Q. Yang, Polymer, 2006, 47, 12–17 CrossRef CAS.
- D. Yan, Y. Zhou and J. Hou, Science, 2004, 303, 65–67 CrossRef CAS.
- C. L. Larson and S. A. Tucker, Appl. Spectrosc., 2001, 55, 679–683 CAS.
- D. Wang and T. Imae, J. Am. Chem. Soc., 2004, 126, 13204–13205 CrossRef CAS.
- D. Wang, T. Imae and M. Miki, J. Colloid Interface Sci., 2007, 306, 222–227 CrossRef CAS.
- W. Wang and C.-Y. Pan, Macromol. Rapid Commun., 2009, 30, 2096–2101 CrossRef.
- G. Jiang, Y. Wang, X. Sun and J. Shen, Polym. Chem., 2010, 1, 618–620 RSC.
- L. Pastor-Pérez, Y. Chen, Z. Shen, A. Lahoz and S.-E. Stiriba, Macromol. Rapid Commun., 2007, 28, 1404–1409 CrossRef CAS.
- P. Wang, X. Wang, K. Meng, H. Song, X. Liu, H. Cheng and C. C. Han, J. Polym. Sci., Part A: Polym. Chem., 2008, 46, 3424–3428 CrossRef CAS.
- B. Fei, Z. Yang, S. Shao, S. Wan and J. H. Xin, Polymer, 2010, 51, 1845–1852 CrossRef CAS.
- D. Wan, G. Wang, H. Pu and M. Jin, Macromolecules, 2009, 42, 6448–6456 CrossRef CAS.
- D. Wan, J. Yuan and H. Pu, Macromolecules, 2009, 42, 1533–1540 CrossRef CAS.
- D. Wan, H. Pu and M. Jin, Macromolecules, 2010, 43, 3809–3816 CrossRef CAS.
- Y. Sun and Y. Xia, Science, 2002, 298, 2176–2178 CrossRef CAS.
- S. Bharathi, N. Fishelson and O. Lev, Langmuir, 1999, 15, 1929–1937 CrossRef CAS.
- L. Maya, G. Muralidaran, T. G. Thundat and E. A. Kenik, Langmuir, 2000, 16, 9151–9154 CrossRef CAS.
- M. Ozsoz, A. Erdem, K. Kerman, D. Ozkan, B. Tuqrul, N. Topcuoglu, H. Ekren and M. Taylan, Anal. Chem., 2003, 75, 2181–2187 CrossRef CAS.
- M. A. P. Dekkers, M. J. Lippits and B. E. Nieuwenhuys, Catal. Today, 1999, 54, 381–390 CrossRef CAS.
- W. I. Lee, Y. Bae and A. J. Bard, J. Am. Chem. Soc., 2004, 126, 8358–8359 CrossRef CAS.
- M. Bruchez Jr., M. Moronne, P. Gin, S. Weiss and A. P. Alivisatos, Science, 1998, 281, 2013–2106 CrossRef CAS.
- R. Gill, I. Willner, I. Shweky and U. Banin, J. Phys. Chem. B, 2005, 109, 23715–23719 CrossRef CAS.
- Y. A. Lee, J. E. McGarrah, R. J. Lachicotte and R. Eisenberg, J. Am. Chem. Soc., 2002, 124, 10662–10663 CrossRef CAS.
- Y. Lin, J.-W. Gao, H.-W. Liu and Y.-S. Li, Macromolecules, 2009, 42, 3237–3246 CrossRef CAS.
- W. I. Lee, Y. J. Bae and A. J. Bard, J. Am. Chem. Soc., 2004, 126, 8358–8359 CrossRef CAS.
- D. Wang and T. Imae, J. Am. Chem. Soc., 2004, 126, 13204–13205 CrossRef CAS.
- D. Wu, Y. Liu, C. He and S. H. Goh, Macromolecules, 2005, 38, 9906–9909 CrossRef CAS.
- M. C. Daniel and D. Astruc, Chem. Rev., 2004, 104, 293–346 CrossRef CAS.
- H. Wang, H. Wang, S. Chen and X. Li, Synth. Met., 2008, 158(11), 437–441 CrossRef CAS.
- J. P. Wilcoxon, J. E. Martin, F. Parsapour, B. Wiedenman and D. F. Kelley, J. Chem. Phys., 1998, 108, 9137–9143 CrossRef CAS.
- J. K. Gimzewski, J. K. Sass, R. R. Schlitter and J. Schott, Europhys. Lett., 1989, 8, 435–440 CrossRef.
- M. Brust, J. Fink, D. Bethell, D. J. Schiffrin and C. Kiely, J. Chem. Soc., Chem. Commun., 1995, 8, 1655–1656 Search PubMed.
- M. R. V. Sahyun and N. Serpone, J. Phys. Chem. A, 1997, 101, 9877–9883 CrossRef CAS.
- T. Huang and R. W. Murray, J. Phys. Chem. B, 2001, 105, 12498–12502 CrossRef CAS.
|
This journal is © The Royal Society of Chemistry 2010 |