DOI:
10.1039/C0PY00163E
(Paper)
Polym. Chem., 2010,
1, 1662-1668
A facile method for the homogeneous synthesis of cyanoethyl cellulose in NaOH/urea aqueous solutions
Received
23rd May 2010
, Accepted 18th July 2010
First published on
6th September 2010
Abstract
A series of cyanoethyl cellulose (CEC) samples were synthesized from cellulose in NaOH/urea aqueous solutions by a homogeneous method. Structure and properties of CECs were characterized with FT-IR, 1H and 13C NMR, DSC, SEC-LLS, polarized light microscopy and solubility measurements. The total DS values of the obtained CECs were in the range of 0.26 to 1.93, and the relative reactivity of hydroxyl groups is in the order C-6 > C-2 > C-3. As the molar ratio of acrylonitrile to anhydroglucose unit (AGU) of cellulose increased to 5
:
1, the C-6 hydroxyl groups of AGU were fully substituted. The total DS value for water-soluble CEC is as low as 0.54. CEC could be dissolved in many organic solvents when its total DS value reached 1.37. Organic-soluble CEC samples displayed thermotropic liquid crystalline behavior in the temperature range of 180 to 280 °C. Moreover, NaOH/urea aqueous solution was proved to be a stable medium for cyanoethylation of cellulose, the reaction products could be easy isolated and purified, and the solvents could be recycled by a simple filtration. Therefore, this work provides a facile method for the homogeneous synthesis of CEC in an aqueous system.
Introduction
Cellulose is the most common and inexhaustible raw material and can be converted into various derivatives and regenerated materials.1 However, cellulose forms unique microcrystal structures through strong hydrogen bonding networks, leading to difficulty of dissolution in common solvents. There is a lack of a green method and the limited number of common solvents that can dissolve cellulose. Therefore, chemical modification reactions continue to play an important role in improving cellulose utilization in polymeric materials.2,3 Over the past 30 years, the discovery of the novel solvents has attracted great interest for both science research and commercial applications.4 Various cellulose derivatives have been successfully synthesized through homogeneous functionalization in N,N-dimethylacetamide (DMAc)/LiCl,5–7 dimethyl sulfoxide (DMSO)/tetrabutylammonium fluoride trihydrate (TBAF),8,9 LiCl/1,3-dimethyl-2-imidazolidinone (DMI),10 and N-methylmorpholine-N-oxide monohydrate (NMMO·H2O).11,12 Recently, some ionic liquids (ILs) were found to dissolve cellulose efficiently.13,14 ILs, as the “green” reaction media for efficient homogeneous chemical modification of cellulose, have drawn much attention from around the world.15–18 In our laboratory, NaOH/urea aqueous solution has been proved to be a good and non-toxic solvent for cellulose. Regenerated cellulose fibers and films with excellent mechanical properties have been prepared from the solvent system.19,20 Moreover, NaOH/urea aqueous solution is a suitable homogeneous reaction medium for the etherification of cellulose because of the basicity of the solvent system. Various cellulose ethers such as methyl and hydroxypropyl cellulose,21 hydroxyethyl cellulose,22 carboxymethyl cellulose,23 and novel cellulose polyelectrolytes24,25 have been successfully synthesized under homogeneous conditions.
Cyanoethylation has been extensively studied with many macromolecular substances containing free hydroxyl groups, such as cellulose,26,27 ethylcellulose,28 chitosan,29 bagasse30 and wood31 in previous papers. Among the cyanoethylated materials, considerable attention has been focused on the investigations of the cyanoethyl cellulose (CEC) due to its outstanding physical and chemical properties.32–34 The degree of substitution (DS) is usually considered to be one of the most important factors in affecting the properties of CEC. Partial cyanoethylation of cellulose exhibits an increased thermal resistance, microbiological resistance, moisture regain and mechanical properties.35 CEC with a high DS value could be used as dielectric materials due to its unusual dielectric properties, namely high dielectric constant and relatively low dielectric loss factor.36 Moreover, they can also form cholesteric liquid crystalline phase in many organic solvents at high concentrations.37,38 However, cyanoethylation of cellulose usually proceeded heterogeneously with a large amount of acrylonitrile, and only a limited number of solvent systems such as cellulose xanthogenate (viscose medium),39 paraformaldehyde (PF)-DMSO system,40 and NMMO·H2O11,41 have been reported. In the present work, a series of CEC samples with different DS values and solubility were synthesized through homogeneous reaction in 7 wt% NaOH/12 wt% urea aqueous solutions. Our main objective is to investigate the structure and physical properties of the CEC samples, and to estimate the characteristics of this solvent system as a new homogeneous reaction medium for the cyanoethylation of cellulose.
Experimental
Materials
Cellulose (cotton linter pulp) was supplied by Hubei Chemical Fiber Group Ltd. (Xiangfan, China), and the viscosity-average molecular weight (Mη) of the cellulose was determined by viscometry in cadoxen42 to be 10.3 × 104 g mol−1. Acrylonitrile and other reagents were of analytical grade and used without further purification.
Preparation of the cellulose solution
Cellulose solution was prepared according the following method. An adequate amount of NaOH, urea and distilled water (7
:
12
:
81 by weight) were added into a 250 mL beaker, and the resulting mixture was stored in a refrigerator. After the solution was precooled to −12.3 °C, cellulose was added immediately into it with stirring vigorously for 5 min at ambient temperature to obtain a transparent cellulose dope. Before use, the cellulose solution was subjected to centrifugation at 8000 rpm for 20 min at 5–10 °C to exclude the slightly remaining undissolved part (about 5 wt%).
Synthesis of CEC in NaOH/urea aqueous solutions
Typically, a certain amount of acrylonitrile was added dropwise into the 500 g cellulose solution prepared above, and the mixture was stirred at 5–10 °C for 6 h. According to Table 1, by changing the molar ratio of acrylonitrile to anhydroglucose unit (AGU) of cellulose from 1
:
1 to 9
:
1, eight CEC samples were prepared. The reaction products of CEC-1 to CEC-4 were neutralized with acetic acid. CEC-1 and CEC-2 were washed by the mixture solution of water and acetone (1
:
1 in volume), and then dried in vacuum. CEC-3 and CEC-4 were dialyzed with regenerated cellulose tubes (Mw cutoff 8000, USA.) against distilled water for 7 days, and finally freeze-dried with lyophilizer (Christ Alpha 1–2, Osterode am Harz, Germany). The reaction products of CEC-5 to CEC-8 were filtered, and the precipitates were neutralized with acetic acid and washed with distilled water, and then dried under vacuum.
Table 1 Synthesis, nitrogen content and distribution of substitution of CEC samples
Sample |
W
cellulose
/g |
Molar ratiob |
W
CEC
/g |
Content of N (%) |
Total DSd |
DS at positionsf |
C-2 |
C-6 |
C-3 |
The weight of the original cellulose (g).
Molar ratio of the anhydroglucose unit of cellulose to acrylonitrile.
The dry weight of CEC obtained (g).
Calculated from nitrogen content of the samples.
Determined by nitrogen content and 13C NMR spectra.
DS values at positions C-2 and C-6 were determined by 13C NMR, DSC-3 = Total DS − DSC-6 − DSC-2.
|
CEC-1 |
19.2 |
1 : 1 |
20.5 |
2.08 |
0.26 |
— |
— |
— |
CEC-2 |
19.5 |
1 : 1.5 |
21.0 |
2.59 |
0.33 |
— |
— |
— |
CEC-3 |
19.4 |
1 : 2 |
21.3 |
2.86 |
0.54e |
0.07 |
0.46 |
— |
CEC-4 |
19.4 |
1 : 3 |
21.4 |
3.45 |
0.69e |
0.13 |
0.57 |
— |
CEC-5 |
19.3 |
1 : 4 |
23.4 |
8.16 |
1.37 |
0.39 |
0.91 |
0.07 |
CEC-6 |
19.4 |
1 : 5 |
28.4 |
8.75 |
1.51 |
0.48 |
1 |
0.06 |
CEC-7 |
19.4 |
1 : 6 |
27.3 |
9.68 |
1.77 |
0.52 |
1 |
0.25 |
CEC-8 |
19.2 |
1 : 9 |
28.8 |
10.23 |
1.93 |
0.62 |
1 |
0.31 |
Characterizations
FT-IR spectra of samples were performed with a Nicolet 170SX Fourier transform infrared spectrometer. The test specimens were prepared by the KBr-disk method. The elemental analysis of the derivatives was measured with an elemental analyzer (CHN–O-Rapid, Foss Hereaus GmbH, Hanau, Germany). The total DS values of the samples are expressed as follows:40
DS = (162 × N%)/(1400 − 53 × N%) |
1H and 13C NMR measurements of the CEC samples in deuterated DMSO (DMSO-d6) at 65 °C were carried on a Varian INOVA-600 spectrometer (1H frequency = 599.81 MHz, 13C frequency = 150.82 MHz) in the proton noise-decoupling mode with a standard 5 mm probe, and the sample concentration was about 4 wt%. The parameters used were as follows: the pulse angle was 28°, relaxation delay was 2.0 s and spectra width was 36003 Hz. At least 3000 scans were accumulated for each spectrum. The chemical shifts were referenced to the signals of DMSO-d6 and tetramethylsilane (TMS).
The solubility of the CEC samples in different solvents was measured at 25 °C, and the concentration was about 1% (w/v). Size exclusion chromatography (SEC) combined with laser light scattering (LLS), was used to determine the molecular weight of the CEC samples. For water-soluble CEC-3 and CEC-4 samples, SEC-LLS measurements were performed on a multi-angle laser light scattering instrument (DAWN DSP, Wyatt Technology Co. United States) equipped with a He–Ne laser (λ = 632.8 nm), and combined with a pump p100 equipped with TSK GEL G6000 and G4000 PWXL column (MicroPak, TSK) and an Optilab refractometer (Wyatt Technology) at 25 °C. The eluent was 0.1 mol L−1 NaCl aqueous solution at a flow rate of 1.0 mL min−1. The specific refractive-index increments (dn/dc) of CEC-3 and CEC-4 in 0.1 mol L−1 NaCl aqueous solution was 0.148 cm3 g−1, and was taken with an Optilab refractometer (Wyatt Technology) at 632.8 nm and 25 °C. For organic-soluble samples (CEC-5 to CEC-8), the column for SEC-LLS measurement was TSK GEL G6000H6 (MicroPak, TSK). The eluent was redistilled DMSO at a flow rate of 1.0 mL min−1. The dn/dc of CEC-5 to CEC-8 in DMSO was determined to be 0.058 cm3 g−1. Astra software was used for data acquisition and analysis.
Differential scanning calorimetry (DSC) was performed on a DSC-200PC (NETZSCH, Germany) under a nitrogen atmosphere at a heating rate of 10 °C min−1 from 30 to 300 °C. Before the test, the CEC samples were heated from room temperature to 110 °C and annealed for 3 min to remove moisture, and then cooled to room temperature with liquid nitrogen.
The thermotropic liquid crystalline properties of CEC samples were studied using OLMPUS BX51 polarized light microscope with a hot stage at a heating rate of 10 °C min−1.
Results and discussion
Homogeneous synthesis of CEC
Cyanoethylation is the classical example of cellulose etherification by Michael addition of an activated C
C bond to a partially activated cellulosic hydroxyl group in an aqueous alkaline medium.43Scheme 1 illustrates the main and side reactions in the formation of CEC, and Table 1 lists the reaction conditions of homogeneous cyanoethylation of cellulose dissolved in NaOH/urea aqueous solution. Acrylonitrile could react with cellulose directly in NaOH/urea aqueous solution without addition of an extra catalyst attributing to the basicity of the solvent system. The reaction was selected at a temperature between 5 and 10 °C as the cellulose solution gets gelled and the side reactions will occur at the higher temperatures. When the etherification agent of acrylonitrile was added dropwise, the temperature of the reaction system increased slightly, indicating its exothermic behavior. The viscosity of the reaction solution apparently decreased, and generated a lot of white foam, suggesting that the reaction products have good emulsifying effect. As shown in Table 1, eight CEC samples were obtained by changing the molar ratio of acrylonitrile to AGU from 1
:
1 to 9
:
1. For the CEC-5 to CEC-8 reaction systems, a large amount of white powder-like solid precipitated in solution as the reaction proceeded, indicating the four products are water-insoluble. The reaction products of CEC-1 and CEC-2 transferred into gels when neutralized with acetic acid, suggesting that they are also water-insoluble but soluble in an alkaline solvent. CEC-3 and CEC-4 reaction systems remained as a transparent solution when neutralized with acetic acid, demonstrating that the two products are water-soluble.
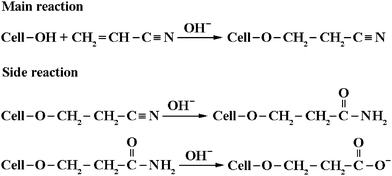 |
| Scheme 1 Main and side reactions in the formation of cyanoethyl cellulose. | |
Fig. 1 shows the dependence of the molar ratio of acrylonitrile to AGU on the nitrogen content of the CEC samples. The nitrogen content of the derivatives increased from 2.08% to 10.23%, and the degree of substitution (DS) calculated from the nitrogen content increased from 0.26 to 1.93, with increasing molar ratio of acrylonitrile to AGU from 1
:
1 to 9
:
1. It is noted that there is a big step for the nitrogen content from CEC-4 to CEC-5. As illustrated in Scheme 1, cyanoethyl can be saponified to carbamoylethyl, and then to carboxyl, and to give carboxyethylcellulose as the stable end-product in an alkaline aqueous medium at elevated temperature.43 Therefore, it is possible that CEC-3 and CEC-4 are hydrolyzed partially to carboxyethylcellulose during the long time of dialysis under slightly alkaline or acid conditions. Moreover, the result will be further proved by NMR technique. Comparing with the traditional heterogeneous procedures and other homogeneous solvent systems,11,39–41 cyanoethylation of cellulose in NaOH/urea aqueous solution displayed quicker reactivity and higher transfer efficiency (above 30%) of etherifying agent. Moreover, because of the CEC samples with higher DS values (>1.37) are insoluble in water and the solvent system, the reaction products can be easy isolated and purified, and the solvents can be recycled by a simple filtration.
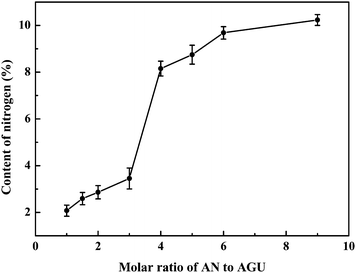 |
| Fig. 1 Effect of molar ratio of acrylonitrile (AN) to the anhydroglucose unit (AGU) of cellulose on the nitrogen content of CEC samples. | |
Structure analysis
Fig. 2 shows the FT-IR spectra of the native cellulose and the CEC samples. All of the as-obtained CEC samples displayed a characteristic absorption band at around 2250 cm−1, which could be assigned to stretching vibration of –C
N groups.41 The intensity of this absorption band increased with increasing molar ratio of acrylonitrile to AGU of cellulose, indicating that the DS value of the samples increase with increasing acrylonitrile addition. Moreover, the strong band at around 3400–3500 cm−1, which is referenced as the stretching vibration of –OH groups for the native cellulose, became weaker and narrower for the CEC-5 and CEC-8 samples attributing to the introduction of a large amount of cyanoethyl groups.
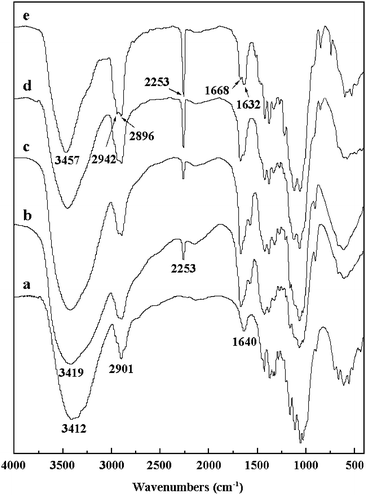 |
| Fig. 2 FT-IR spectra of the original cellulose (a) and CEC samples (b, CEC-1; c, CEC-3; d, CEC-5; e, CEC-8). | |
Fig. 3 shows 1H NMR spectra of CEC samples in DMSO-d6 at 65 °C. The possible assignments of the peaks are shown according to Nakayama and Azuma.44 Signals at 2.6–2.9 ppm were assigned to protons of methylene that connected to cyanoethyl groups (–CH2CN), which increased with the increasing DS values of CEC samples. The broad peaks at 3.0–5.8 ppm were attributed to methylene of the substituents and all the protons of anhydroglucose ring, which were overlapped with H2O at 3.0–3.2 ppm.
13C NMR spectra of CEC samples in DMSO-d6 at 65 °C are shown in Fig. 4, and the possible assignments of the peaks are also shown according to previous work.44 The chemical shifts at 119.9 ppm and 18.8 ppm are assigned to the carbon signals of –CN and –CH2CN, respectively. The peaks C-6 (61.2 ppm) and C-6s (69.0 ppm) were assigned to C-6 carbons bearing unsubstituted and substituted hydroxyl groups, respectively, whereas the chemical shifts of 103.6 ppm (C-1) and 101.8 ppm (C-1s) were due to C-1 carbons adjacent to C-2 bearing unsubstituted and substituted hydroxyl groups, respectively. The peak intensity of C-6 (61.2 ppm) was found to become weaker, whereas a new peak C-6s at 69.0 ppm appeared with increase of the total DS value. As the molar ratio of acrylonitrile to AGU reached to 5
:
1, the peaks at 61.2 ppm disappeared, indicating the C-6 hydroxyl groups of AGU were fully substituted. Moreover, for the spectra of CEC-3 and CEC-4, the signals at 36.6 ppm and 173.2 ppm are the chemical shifts of carbons for –CH2CH2COO− and –CH2CH2COO−, respectively, which attributed to the partially hydrolysis of cyanoethyl to carboxyethyl groups. The chemical shifts of –CH2CH2COO− for CEC-3 and CEC-4 are overlapped with the carbon signals of –CH2CH2CN (66.2 ppm). According to the 13C NMR spectra, the DS values of C-6 and C-2 could be estimated from the relative intensities of peaks C-6 and C-6s, C-1 and C-1s, respectively. Therefore, the DS values of C-3 could be calculated from the total DS values obtained by the element analysis and the DS values of C-6 and C-2. The total DS and the relative DS values at C-2, C-3 and C-6 carbon positions for the CEC samples are summarized in Table 1. It can be concluded that the relative reactivity of hydroxyl groups is in the order C-6 > C-2 > C-3 in this aqueous solvent system, which are similar to carboxymethyl cellulose (CMC) prepared in Ni(tren)(OH)2 aqueous solutions (a fully homogeneous process) and the commercial production of CMC prepared in a highly swollen states.3 The result further proved that both simple activation of cellulose with aqueous NaOH and the complete dissolution of the polysaccharide leads to reactive sites with a similar accessibility.45
Solubility and molecular weight
Table 2 presents the solubility of CEC samples in different solvents. CEC-1 and CEC-2 samples were only soluble in dilute alkali solutions and swelled in water and DMSO due to their low DS values. CEC-3 and CEC-4 samples showed good solubility in water, DMSO, DMF and dilute alkali solution. So far water-soluble cyanoethylated cellulose has only been known to be prepared by homogeneous reaction in viscose medium,39 PF-DMSO40 and NMMO·H2O11,41 systems. It has been reported that CEC with DS = 0.77 prepared by homogeneous reaction in viscose medium is soluble in water.39 In the present work, the total DS value for water-soluble CEC is as low as 0.54. As the DS value increased to 1.37, CEC samples (CEC-5 to CEC-8) cannot be dissolved in water and dilute alkali solution, but have good solubility in many organic solvents, such as DMSO, DMF and pyridine. It is mentioning that alkali-soluble products in the DS region of about 0.4 and water-soluble CEC in the DS range between 0.7 and 1.0 have been prepared in NMMO·H2O by a suitable preactivation of the starting material.41 CEC obtained in a thoroughly homogeneous procedure exhibited organosolubilty at a DS above 0.8, while a DS above 2.0 was necessary along a heterogeneous route. It is also well known that the solubility of cellulose ethers in various solvents is not only governed by the total DS, but also the substituent distribution within an AGU unit and along the molecular chain plays an important role.43 A homogeneous procedure ensures a more uniform distribution of the substituents because of greater accessibility of –OH. Though the reaction reactivity of C-6 hydroxyl groups is higher than that of C-2 and C-3, CEC prepared in NaOH/urea aqueous solutions probably possessed the more uniform substituent distribution along the cellulose backbone than the products prepared from the heterogeneous procedures and other homogeneous methods, which resulted in the relative lower total DS value for water-soluble and organic soluble products.
Table 2 Solubility, Mw, Mn and d Values of CEC Samples
Sample |
Solubilitya |
M
w × 10−4/g mol−1 |
M
n × 10−4/g mol−1 |
d
|
0.1 M NaOH |
H2O |
DMSO |
− insoluble, + soluble, ○ swelling for a 1% (w/v) solution at 25 °C.
|
CEC-1 |
+ |
○ |
○ |
— |
— |
— |
CEC-2 |
+ |
○ |
○ |
— |
— |
— |
CEC-3 |
+ |
+ |
+ |
10.63 |
8.85 |
1.20 |
CEC-4 |
+ |
+ |
+ |
11.41 |
8.70 |
1.31 |
CEC-5 |
− |
− |
+ |
12.22 |
9.27 |
1.32 |
CEC-6 |
− |
− |
+ |
15.01 |
12.37 |
1.21 |
CEC-7 |
− |
− |
+ |
14.06 |
10.45 |
1.35 |
CEC-8 |
− |
− |
+ |
13.92 |
11.10 |
1.25 |
SEC-LLS chromatograms for CEC-3 in 0.1 mol L−1 NaCl aqueous solution and CEC-7 in DMSO at 25 °C are shown in Fig. 5. From the signals detected by LLS and differential refractometry, the weight-average molecular weight (Mw), the number-average molecular weight (Mn), and the polydispersity index (d = Mw/Mn) of CEC samples were determined, and they are summarized in Table 2. The Mw and Mn values of the CEC samples are in good agreement with the molecular weight calculated from Mη of the original cellulose, suggesting that the 7 wt% NaOH/12 wt% urea aqueous solution was a stable system for cyanoethylation of cellulose.
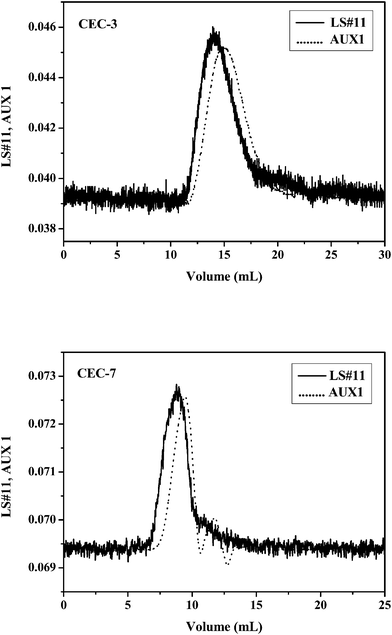 |
| Fig. 5 LLS-SEC chromatograms of CEC-3 in 0.1 mol L−1 NaCl aqueous solution and CEC-7 in DMSO at 25 °C, as detected by LLS and differential refractometry. LS#11 and AUX1 represent signals from LLS at 90° and the refractive-index detection. | |
Thermotropic behavior
DSC thermograms of CEC samples and the raw cellulose are shown in Fig. 6. Due to the release of free water during the first DSC scanning, a broad endothermic peak appeared at 130 °C on the second DSC scanning was related to the crystallization of water for cellulose. Similar to cellulose, the CEC-1 to CEC-4 samples exhibited endothermic peaks in the range of 150 °C to 175 °C due to the release of crystal water. In addition, the endothermic peaks for CEC-1 to CEC-4 shifted toward higher temperature and became more significant, attributing to the increasing of hydrophilicity of the products with an increase of DS value. For CEC-5, an endothermic peak could be found at the temperature range of 130 to 170 °C (the peak temperature was 139 °C), and the enthalpy value was calculated to be 44.8 J g−1, which probably be due to the melting of crystalline and the release of crystal water. As to CEC-6 to CEC-8, the characteristic crystalline melting peak could be found at 140 °C, 160 °C and 181 °C, and the enthalpy values were 8.5 J g−1, 20.2 J g−1 and 25.1 J g−1, respectively, which increased with increasing DS value of the sample. The second endothermic peak appeared above 250 °C was assigned to the partial decomposition of CEC samples.
Fig. 7 shows the polarized light micrographs of CEC-8 recorded in the temperature range of 180 to 280 °C. On the heating procedure, CEC-8 started to melt at 180 °C and the strong birefringence was observed between 180 and 280 °C. Similar to CEC-8, the samples CEC-6 and CEC-7 also displayed thermotropic liquid crystalline behavior at the temperature range of 180 to 280 °C. However, CEC-5 did not show obvious mobility and a liquid crystalline phase at the temperature range of 180 to 250 °C. It was also observed that CEC-5 started to melt and birefringence appeared at around 270 °C, with accompanied carbonization and decomposition. Because of the low DS value, CEC-1 to CEC-4 samples could not be melted and no birefringence could be observed under crossed polarizing light microscope even after they were heated to 280 °C on the hot stage. All the samples carbonized obviously when they were heated above 280 °C. The observations of polarized light microscope agreed well with the results from DSC measurements.
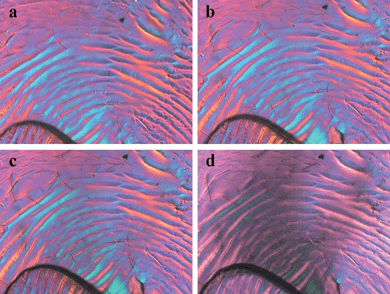 |
| Fig. 7 Polarized light micrographs of CEC-8 (DS = 1.93) at (a) 180 °C, (b) 220 °C, (c) 250 °C and (d) 280 °C, respectively. The images were obtained using a polarized light microscope at the magnification of 400×. | |
Conclusions
Eight CEC samples were homogeneously synthesized in NaOH/urea aqueous solutions under moderate conditions. The total DS values of the CEC samples increased from 0.26 to 1.93 with increasing molar ratio of acrylonitrile to AGU from 1
:
1 to 9
:
1. The relative reactivity of hydroxyl groups at the C-6 position was higher than that of C-2 and C-3 positions. As the molar ratio of acrylonitrile to AGU exceeded to 5
:
1, the C-6 hydroxyl groups of AGU were fully substituted. The DS value for water-soluble CEC was as low as 0.54, but exhibited good solubility in organic solvents as the DS value increased to 1.37. CEC with high DS value displayed apparent thermotropic liquid crystalline behavior at the temperature above its melting point. Compared with the traditional heterogeneous procedures, homogeneous cyanoethylation of cellulose in NaOH/urea aqueous solution displays quicker reactivity and higher transfer efficiency of etherifying agent. This work provided a new environmentally friendly method to synthesize water-soluble and organic-soluble cyanoethyl celluloses.
Acknowledgements
This work was financially supported by the National Basic Research Program of China (973 Program, 2010CB732203), the National Natural Science Foundation of China (50973085), the Fundamental Research Funds for the Central Universities (2081005) and Engineering Research Center for Biomass Modified Materials, Sichuan Province, China.
Notes and references
- D. Klemm, B. Heublein, H.-P. Fink and A. Bohn, Angew. Chem., Int. Ed., 2005, 44, 3358 CrossRef CAS.
- T. Heinze, T. Liebert, P. Klüfers and F. Meister, Cellulose, 1999, 6, 153 CrossRef CAS.
- V. Jollet, F. Chambon, F. Rataboul, A. Cabiac, C. Pinel, E. Guillon and N. Essayem, Green Chem., 2009, 11, 2052 RSC.
- T. Heinze and T. Liebert, Prog. Polym. Sci., 2001, 26, 1689 CrossRef CAS.
- C. L. McCormick and P. A. Callais, Polymer, 1987, 28, 2317 CrossRef CAS.
- T. Liebert and T. Heinze, Biomacromolecules, 2001, 2, 1124 CrossRef CAS.
- D. Fenn and T. Heinze, Cellulose, 2009, 16, 853 CrossRef CAS.
- B. A. P. Ass, E. Frollini and T. Heinze, Macromol. Biosci., 2004, 4, 1008 CrossRef CAS.
- M. A. Hussain, T. Liebert and T. Heinze, Macromol. Rapid Commun., 2004, 25, 916 CrossRef CAS.
- A. Takaragi, M. Minoda, T. Miyamoto, H. Liu and L. Zhang, Cellulose, 1999, 6, 93 CrossRef CAS.
-
D. L. Johnson, Compounds dissolved in cyclic amine oxides, US Pat., 3447939, 1969.
- B. Volkert and W. Wagenknecht, Macromol. Symp., 2008, 262, 97 CrossRef CAS.
- R. P. Swatloski, S. K. Spear, J. D. Holbrey and R. D. Rogers, J. Am. Chem. Soc., 2002, 124, 4974 CrossRef CAS.
- H. Zhang, J. Wu, J. Zhang and J. He, Macromolecules, 2005, 38, 8272 CrossRef CAS.
- J. Wu, J. Zhang, H. Zhang, J. He, Q. Ren and M. Guo, Biomacromolecules, 2004, 5, 266 CrossRef CAS.
- K. Schlufter, H.-P. Schmauder, S. Dorn and T. Heinze, Macromol. Rapid Commun., 2006, 27, 1670 CrossRef CAS.
- M. Gericke, T. Liebert and T. Heinze, J. Am. Chem. Soc., 2009, 131, 13220 CrossRef CAS.
- C. Yan, J. Zhang, Y. Lv, J. Yu, J. Wu, J. Zhang and J. He, Biomacromolecules, 2009, 10, 2013 CrossRef CAS.
- J. Cai, L. Zhang, J. Zhou, H. Qi, H. Chen, T. Kondo, X. Chen and B. Chu, Adv. Mater., 2007, 19, 821 CrossRef CAS.
- H. Qi, C. Chang and L. Zhang, Green Chem., 2009, 11, 177 RSC.
- J. Zhou, L. Zhang, Q. Deng and X. Wu, J. Polym. Sci., Part A: Polym. Chem., 2004, 42, 5911 CrossRef CAS.
- J. Zhou, Y. Qin, S. Liu and L. Zhang, Macromol. Biosci., 2006, 6, 84 CrossRef CAS.
- H. Qi, T. Liebert, F. Meister and T. Heinze, React. Funct. Polym., 2009, 69, 779 CrossRef CAS.
- Y. Song, Y. Sun, X. Zhang, J. Zhou and L. Zhang, Biomacromolecules, 2008, 9, 2259 CrossRef CAS.
- Y. Song, J. Zhou, L. Zhang and X. Wu, Carbohydr. Polym., 2008, 73, 18 CrossRef CAS.
- A. Hebeish, A. H. Zahran and A. M. Kh. EI-Naggar, J. Appl. Polym. Sci., 1985, 30, 4057 CrossRef CAS.
- M. Z. Sefain, M. H. Fadl, N. A. EI-Wakil and M. M. Naoum, Polym. Int., 1993, 32, 251 CrossRef CAS.
- Y. Huang, J. Loos, Y. Yang and J. Petermann, J. Polym. Sci., Part B: Polym. Phys., 1998, 36, 439 CrossRef CAS.
- Y. Dong, Y. Qing and Y. Huang, J. Polym. Sci., Part B: Polym. Phys., 2000, 38, 980 CrossRef CAS.
- M. L. Hassan, N. A. EL-Wakil and M. Sefain, J. Appl. Polym. Sci., 2001, 79, 1965 CrossRef CAS.
- Y. Yamawaki, M. Morita and I. Sakata, J. Appl. Polym. Sci., 1990, 40, 1757 CrossRef CAS.
- P. K. Chatterjee and C. M. Conrad, J. Polym. Sci., Part A-1, 1966, 4, 233 CrossRef CAS.
- W. Tsuji, T. Nakao, K. Ohigashi, K. Maegawa, N. Kobayashi, S. Shukri, S. Kasai and K. Miyanaga, J. Appl. Polym. Sci., 1986, 32, 5175 CrossRef CAS.
- A. K. Saha, S. Das, R. K. Basak, D. Bhatta and B. C. Mitra, J. Appl. Polym. Sci., 2000, 78, 495 CrossRef CAS.
- A. K. Saha and B. C. Mitra, J. Appl. Polym. Sci., 1996, 62, 733 CrossRef CAS.
- G. R. Saad, Polym. Int., 1994, 34, 411 CrossRef CAS.
- L. Wang and Y. Huang, Macromolecules, 2002, 35, 3111 CrossRef CAS.
- S. A. Vshivkov and E. V. Rusinova, Polym. Sci., 2008, 50, 725 Search PubMed.
- J. H. MacGregor, J. Soc. Dyers Colour., 1951, 67, 66 CAS.
- T. Morooka, M. Norimoto and T. Yamada, J. Appl. Polym. Sci., 1986, 32, 3575 CrossRef CAS.
- B. Philipp, B. Lukanoff, H. Schleicher and W. Wagenknecht, Z. Chem., 1986, 26, 50 CAS.
- W. Brown and R. Wiskstön, Eur. Polym. J., 1965, 1, 1 CrossRef CAS.
-
D. Klemm, B. Philipp, T. Heinze, U. Heinze and W. Wagenknecht, Comprehensive cellulose chemistry. Functionalization of cellulose, WILEY-VCH Verlag GmbH, Weinheim, 1998, vol. 2, pp. 250–255 Search PubMed.
- E. Nakayama and J. Azuma, Cellulose, 1998, 5, 175 CrossRef CAS.
- T. Heinze and K. Pfeiffer, Angew. Makromol. Chem., 1999, 266, 37 CrossRef CAS.
|
This journal is © The Royal Society of Chemistry 2010 |
Click here to see how this site uses Cookies. View our privacy policy here.