DOI:
10.1039/C0PY00096E
(Paper)
Polym. Chem., 2010,
1, 1048-1055
Synthesis and preliminary photovoltaic behavior study of a soluble polyimide containing ruthenium complexes
Received
24th March 2010
, Accepted 1st April 2010
First published on
14th May 2010
Abstract
Aiming to prepare photovoltaic materials with light and thermal stability, ruthenium complexes with broad absorption in the visible region were introduced to a polyimide, bpy-PI, which contains a bipyridine ligand in each repeating unit. The chemical structures of the monomers and polymers were confirmed by 1H NMR, IR, and elemental analysis. The prepared polyimide containing ruthenium complexes (bpy-PI–Ru) showed good solubility in common organic solvents such as DMF, NMP, DMSO and DMAc. UV-vis measurements revealed that bpy-PI–Ru exhibits very broad absorptions in the range 350–750 nm due to the introduction of ruthenium complexes. Such absorption enhancement would enable the polymer to harvest solar light in the visible region. The onset oxidation potential of bpy-PI–Ru was measured as 0.68 V (vs. SCE), which is about 0.06 V lower than that of bpy-PI. The band gap of bpy-PI–Ru is 1.35 eV as measured by cyclic voltammetry. Solar cell devices were fabricated based on bpy-PI–Ru or the blend of bpy-PI–Ru and PCBM. Current density-voltage (J-V) measurement of the devices showed a typical rectifying behavior under a 55 mW cm−2 compact white arc lamp. The open-circuit voltages and short-circuit densities were measured to be in the ranges 0.19–0.52 V and 0.0015–0.38 mA cm−2, respectively. The short-circuit density could be further improved by anode modification through deposition of PEDOT:PSS on ITO surface.
Introduction
Photovoltaic cells (PVCs) or solar cells are one of the most attractive ways to use solar energy, an inexhaustible renewable clean energy. Polymeric solar cells (PSCs) have received considerable attention over the past decade for their potential in large-scale power generation based on materials that provide the possibilities of being flexible, light in weight and cheap in price.1 As a potential competitor to silicon-based PVCs, the power conversion efficiencies (PCEs) of PSCs have exceeded 6% with a single-layer PSC device based on fluorinated PTB4/PC61BM.2 Despite these amazing progresses, further significant enhancement of PCE is necessary to meet the requirements for large scale commercialization. Except for the efficiency, the lifetime of PSCs is also an important factor to be concerned. Conjugated organic polymers play a key role in the study of PSCs, while they are normally unstable upon being subjected to thermal, chemical and photophysical actions, which would give a negative impact to the service life of PSCs.3 Therefore, the design of photoactive polymers that have not only an extended absorption edge to match the solar terrestrial radiation, higher carrier mobility and better energy alignment with acceptors to reach a higher circuit voltage, but also good thermal stability to improve the lifetime of photovoltaic devices is highly desired.4
Following the pioneering work of O’Regan and Grätzel,5 dye-sensitized solar cells (DSSCs) have received considerable attention. Classic ruthenium complex photosensitizers, such as cis-di(thiocyanato)-bis(2,2′-bipyridyl-4,4′-dicarboxylate) ruthenium(II), coded as N3 or N719 depending on the number of protons contained,6 have been proven to be able to produce a higher short-circuit current due to the broad UV-vis absorption and long enough excited-state lifetime, which resulted in respectable energy conversion efficiencies (the η value higher than 10%) with fairly good stability.7 Inspired by these peculiar results, ruthenium complexes have been incorporated into polymeric materials either in the main chain8 or side chain9 of a conjugated polymer, and efficient bulk heterojunction (BHJ) PVCs with energy conversion efficiency higher than 4% have been reported.10
On the other hand, polyimides have been proven to be very stable to thermal, chemical and photophysical actions. Polyimide chains consist of alternating electron acceptor diimide fragments (A) and electron donor arylene rests of starting diamines (D).11 Also, polyimides have been widely used in many different technological areas such as microelectronics, coatings, composites, membranes and fibers. The application of polyimides as solar cell materials has also been found in a report,12 where Rhodamine 6G or a perylene moiety was used as the photosensitizer.
Aiming to prepare photovoltaic materials with light and thermal stability, this paper reports the preparation of a polyimide containing ruthenium complexes (bpy-PI–Ru), in which ruthenium complexes were introduced to the polyimide bpy-PI via coordination through the bipyridine ligand in each repeating unit of the polymer chain. With such a molecular design, the light harvesting ability of ruthenium complexes would be combined with the excellent thermal stability of polyimide in one molecule. Solubility, thermal stability and photophysical properties of the obtained bpy-PI–Ru were characterized, and preliminary photovoltaic behaviors were investigated through fabricating solar cell devices based on bpy-PI–Ru or the blend of bpy-PI–Ru with PCBM.
Experimental
Materials
2,2′-Bipyridine-4,4′-dicarboxylic acid [bpy(COOH)2], isoquinoline, m-cresol, [RuCl2(p-cymene)]2, 5% Pd/C and tetrabutylammonium (TBAP) were purchased from Alfa Aesar. 4,4′-(Hexafluoroisopropylidene)-diphthalic anhydride (6FDA) was purchased from Acros. The other chemical reagents were obtained with analytical or chemical purity from Chinese chemical reagent companies. All these chemicals were used as received unless otherwise stated. N,N-dimethylformamide (DMF) was dried with CaH2 overnight, then distilled over CaH2 under reduced pressure before use.
Instruments
1H NMR (400 MHZ) spectra were recorded on a Bruker DPX-400 spectrometer. IR spectra (KBr tablets) were measured on a Nicolet Protégé 460 infrared spectrophotometer. Elemental analysis was measured on an EA 1110 CHNSO instrument. Relative molecular weights were determined by gel permeation chromatography (GPC) on a Waters M515 instrument at 40 °C calibrated with polystyrene standards using tetrahydrofuran as eluent. The melting point was determined using an X-5A melting point measurement instrument. Thermogravemetric analysis (TG) was conducted on a NETZSCH DSC-204 calorimeter with a heating rate of 10 °C min−1. UV-vis spectra were measured on a Shimadzu UV-3010 instrument. Fluorescent (FL) spectra, including excitation and emissive spectrum, were recorded on a Hitachi F-4500 fluorescence spectrophotometer. Electrochemical properties were measured at a scan rate of 100 mV s−1 by cyclic voltammetry (CV) on a RST 3000 electrochemistry workstation with a three-electrode configuration. Polymer films for UV-vis and J-V measurements were prepared by spin coating the polymer solution onto quartz glasses or indium-tin-oxide (ITO) coated glass substrates using a KW-4A spin coater.
Fabrication and characterization of photovoltaic devices
ITO coated glass slides with surface resistivity of 15 Ω sq−1 were used as the substrate for photovoltaic devices. The substrate was pre-cleaned by acetone, ethanol and deionized water in ultrasonic bath, respectively, and then was dried by nitrogen flow. The ITO substrate was directly used as the anode, or upon modification with spin-casting a layer of poly(3,4-ethylenedioxy-thiophene):poly(styrenesulfonate) (PEDOT:PSS) (50 nm). Photoactive polymeric films were prepared by casting a solution of bpy-PI–Ru with concentration of 10 mg mL−1 or a blend solution of bpy-PI–Ru with [6,6]-phenyl-C61-butyric-acid-methyl-ester (PCBM) in CHCl3. The devices were dried in a vacuum oven for 4 h at 60 °C to remove the remaining solvent in polymer films. A layer of aluminium with thickness of 100 nm as the cathode was finally deposited on the polymer film surface using a ZZX-500 apparatus in high vacuum (3 × 10−4 Pa). The thickness of the films was determined to be about 100 nm for all samples by a surface profiler (Ambios Technology XP-2).
The effective area of one cell was about 9 mm2. J-V characteristics of the devices were measured using a Keithley 2410 source meter at a 55 mW cm−2 compact white arc lamp.
Synthesis
Synthetic routes for the diamine monomer, the ligand polymer bpy-PI, and the polymer containing ruthenium complexes bpy-PI–Ru are shown in Schemes 1, 2 and 3, respectively.
 |
| Scheme 1 The synthetic route for the diamine monomer compound 5. | |
 |
| Scheme 2 The synthetic route for polyimide containing bipyridine, bpy-PI. | |
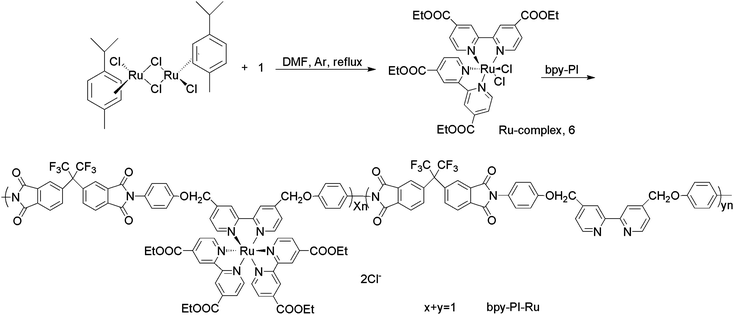 |
| Scheme 3 The synthetic route for polyimide containing ruthenium complexes, bpy-PI–Ru. | |
2,2′-Bipyridine-4,4′-dicarboxydiethyl ester (1)14
Compound 1 was prepared by esterification of bpy(COOH)2 with ethanol. 4.3 mL of concentrated sulfuric acid was added to a suspension of bpy(COOH)2 (1.90 g, 7.8 mmol) in 100 mL of anhydrous ethanol. The mixture was refluxed for 8 h to obtain a clear solution and then cooled to room temperature. The reaction mixture was poured into 200 mL of ice-water, and then adjusted to neutral with 6.25 mol L−1 NaOH solution. The white precipitate was filtered and washed with water (pH = 7). Further purification was conducted by recrystallization from 95.0% ethanol to afford compound 1 (2.12 g, 90.0% yield, mp = 149.0–150.0 °C) as white needle crystals (Found: C, 63.63; H, 5.54. C12H16N2O4 requires C, 63.99; H, 5.37%). δH(400 MHz; CDCl3; Me4Si): 1.45 (6 H, t, J 7.2, 2 × Me), 4.47 (4 H, q, J 6.96, 2 × MeCH2), 7.92 (2 H, d, J 3.68, aryl H on C5 and C5′), 8.88 (2 H, d, J 4.92, aryl H on C6 and C6′), 8.96 (2 H, s, aryl H on C3 and C3′).
Compound 2 was prepared by the reduction of the ester group in compound 1 with sodium borohydride. To a suspension of compound 1 (1.0 g, 3.3 mmol) in 70 mL of anhydrous ethanol cooled with ice-bath to 0 °C, sodium borohydride (2.80 g, 73 mmol) was added in one portion, and stirred for 1 h. The mixture was heated to reflux for 3 h, and then cooled to room temperature. 70 mL of saturated ammonium chloride aqueous solution was added to decompose the excess sodium borohydride. Ethanol was removed under reduced pressure, the remained solid was dissolved in a minimum amount of water and extracted with ethyl acetate (3 × 100 mL). The extract was dried over anhydrous sodium sulfate, and solvent was removed by rotary evaporation. Further purification was conducted by recrystallization from anhydrous ethanol to afford compound 2 (0.72 g, 81.0% yield, mp > 260 °C) as white powder (Found: C, 66.43; H, 5.69. C12H12N2O2 requires C, 66.65; H, 5.59%). δH(400 MHz; DMSO; Me4Si): 4.62 (4 H, d, J 5.73, 2 × CH2OH), 5.53 (2 H, t, J 5, 2 × OH), 7.36 (2 H, d, J 4.65, aryl H on C5 and C5′), 8.39 (2 H, s, aryl H on C3 and C3′), 8.60 (2 H, d, J 4.89, aryl H on C6 and C6′).
Compound 3 was prepared by a nucleophilic substitution of compound 2 with hydrobromic acid. Compound 2 (0.48 g, 4.2 mmol) was dissolved in a mixture of 40% HBr (15 mL) and concentrated sulfuric acid (4 mL). The solution was refluxed for 6 h, then cooled to room temperature, and 20 mL of water was added. The pH value was adjusted to neutral with 6.25 mol L−1 NaOH solution. The precipitate was filtered, washed with water, and air-dried. The crude product was dissolved in chloroform (20 mL), and the insolubles were filtered. The filtrate was evaporated to dryness to obtain compound 3 as white powders (0.44 g, 57.8% yield, mp = 127.0–128.0 °C) (Found: C, 42.05; H, 2.98. C12H10N2Br2 requires C, 42.14; H, 2.95%). δH(400 MHz; CDCl3; Me4Si): 4.49 (4 H, s, 2 × CH2Br), 7.38 (2 H, d, J 5, aryl H on C5 and C5′), 8.45 (2 H, s, aryl H on C3 and C3′), 8.68 (2 H, d, J 5.04, aryl H on C6 and C6′).
Compound 4 was synthesized by a Williamson reaction of compound 3 with 4-nitrophenol. 4-Nitrophenol (1.39 g, 10 mmol) was dissolved in 100 mL of DMF whilst stirring at room temperature, and then KOH (0.56 g, 10 mmol) was added. The solution was heated to 90 °C, and kept at such temperature for 1 h, then compound 3 (1.71 g, 5 mmol) in 10 mL of DMF was dropwise added. The mixture was heated to 110 °C and stirred for 48 h. Once cooled to room temperature, the reaction mixture was poured into distilled water (1000 mL), faint yellow precipitate was formed and filtered. Compound 4 (1.54 g, 67% yield, mp = 254.0–255.5 °C) was obtained as faint yellow needles through recrystallization from DMF (Found: C, 62.55; H, 4.03. C24H18O6N4 requires C, 62.88; H, 3.93%). δH(400 MHz; DMSO; Me4Si): 5.48 (4 H, s, CH2O), 7.28 (4 H, d, J 7.16, Ph), 7.53 (2 H, d, J 4.84, aryl H on C5, C5′), 8.25 (4 H, d, J 7.32, Ph), 8.49 (2 H, s, aryl H on C3 and C3′), 8.72 (2 H, d, J 5 Hz, aryl H on C6 and C6′).
The diamine monomer, compound 5, was prepared by the reduction of compound 4 with hydrazine catalyzed by Pd/C. Compound 4 (2.29 g, 5 mmol) and 0.40 g of 5% Pd/C were suspended in 30 mL of DMF, then 80% hydrazine hydrate (1.87 g, 30 mmol) was added. The suspension was stirred at reflux for 10 h. After cooled to room temperature, 20 mL of DMF was added to dissolve the solid product. Catalyst was removed by filtration, and the filtrate was poured into 500 mL of distilled water. Compound 5 (1.54 g, 77.2% yield, mp = 222.0–223.5 °C) was obtained as white precipitate (Found: C, 71.23; H, 5.54. C24H22O2N4 requires C, 72.36; H, 5.53%). δH(400 MHz; DMSO; Me4Si): 4.64 (4 H, s, CH2O), 5.12 (4 H, s, NH2), 6.51 (4 H, d, J 6.72, Ph), 6.76 (4 H, d, J 6.64, Ph), 7.47 (2 H, d, J 4.04, aryl H on C5, C5′), 8.44 (2 H, s, aryl H on C3, C3′), 8.66 (2 H, d, J 5, aryl H on C6, C6′).
Synthesis of the ligand polymer bpy-PI17
The ligand polymer, polyimide containing bipyridine (bpy-PI), was synthesized by a one-step polycondensation as depicted in Scheme 2. A stoichiometric amount of the diamine monomer, compound 5 (0.40 g, 1 mmol), and the dianhydride monomer, 6FDA (0.44 g, 1 mmol) were added into a solution composed of 2 mL of isoquinoline and 8 mL of m-cresol. The mixture was stirred at ambient temperature for 10 h under argon, and then at 200 °C for another 10 h. After being cooled to room temperature, the viscous solution was poured slowly into 200 mL of methanol under stirring to isolate the polymer. Further purification was carried out by reprecipitation twice from m-cresol solution into methanol, and then thoroughly washed with methanol in a Soxhlet extractor for one day. The product was dried in vacuo yielding bpy-PI as pink solid (0.65 g, 80.6% yield) (Found: C, 63.15; H, 3.23. C43H24F6N4O6 requires C, 64.02; H, 2.98%). IR (KBr, cm−1), 1784 (C
O, υas), 1718 (C
O, υs), 1375 (C–N, υs), 722 (C
O, υ).
Synthesis of the Ru-complex, compound 6
[RuCl2(p-cymene)]2 (0.05 g, 0.08 mmol) was dissolved in dry DMF (15 mL) in dark under argon atmosphere, compound 1 (0.10 g, 0.32 mmol) was added. The reaction mixture was heated to reflux, and stirred vigorously for 4 h. After being cooled to room temperature, solvent was removed by using a rotary evaporator. Distilled water was added and the insolubles were collected on a sintered glass crucible by suction filtration. The solid product was washed with distilled water and diethyl ether, and then dried. Compound 6 was obtained as black powders (0.09 g, 80.2% yield) (Found: C, 49.79; H, 4.16. C32H32Cl2N4O8 Ru requires C, 49.74; H, 4.15%). δH(400 MHz; DMSO; Me4Si): 1.30 (t, J = 7.04 Hz, 6H), 1.45 (t, J = 7.00 Hz, 6H), 4.34 (quart, J = 7.12 Hz, 4H), 4.52 (quart, J = 7.00 Hz, 4H), 7.48 (d, J = 4.72 Hz, 2H), 7.76 (d, J = 6.00 Hz, 2H), 8.25 (d, J = 4.80 Hz, 2H), 8.94 (s, 2H), 9.12 (s, 2H), 10.10 (d, J = 5.88 Hz, 2H).
Synthesis of polyimide containing ruthenium complexes (bpy-PI–Ru)18
Polyimide containing ruthenium complexes (bpy-PI–Ru) was synthesized by the reaction of the ligand polymer bpy-PI with the Ru-complex, compound 6, as depicted in Scheme 3. Compound 6 (0.12 g, 0.16 mmol) was dissolved in 15 mL of DMF, then bpy-PI (0.13 g, 0.16 mmol of bipyridine calculated form the molar mass of repeating unit) in 5 mL of DMF was dropwise added. The reaction mixture was heated to 90 °C and stirred for 4 h. The reaction solution was homogeneous with a dark green color. After condensing through evaporation under reduced pressure, the solution was then dropwise added into ethyl acetate under stirring to precipitate the product. The precipitate was filtrated, washed with ethyl acetate, and dried in vacuo yielding bpy-PI–Ru as brownish black powders (0.16 g, 66.7% yield) (Found: C, 57.08; H, 3.67. C43H24O10N4F6, required: C, 56.99; H, 3.55%).
Results and discussion
Monomer synthesis
The synthetic route for diamine monomer, compound 5 and other intermediates are illustrated in Scheme 1. Their chemical structures were identified by 1H NMR and elemental analysis. The diamine monomer, compound 5, 4,4′-bis[(4-aminophenoxy)-methyl]-2,2′-bipyridine, was synthesized by hydrazine reduction of compound 4 catalyzed with Pd/C. As shown in Fig. 1, 1H NMR spectrum of the diamine monomer 5 illustrates that the nitro groups in compound 4 were completely reduced, in which the signal of amino groups appeared at around δ 5.12 as a singlet; signals at δ 8.66, 7.47 and 8.44 are derived from the bipyridine ring; signals of the benzene ring appeared as two doublets at δ 6.76 and 6.51, and the singlet at δ 4.64 belongs to the oxymethylene protons (–CH2O–).
Synthesis of the ligand polymer bpy-PI.
The ligand polymer, polyimide containing bipyridine (bpy-PI) was prepared by the polycondensation of fluoro-containing dianhydride (6FDA) and the diamine monomer compound 5 using isoquinoline as catalyst in m-cresol at 200 °C. The generated water was removed through a gentle argon flow. The completion of imidization in the obtained polymer bpy-PI was confirmed by IR spectrum and elementary analysis. In the IR spectrum, no absorption in the range 3,000–3,500 cm−1 for O–H vibration band was observed. While three absorptions at 1,784, 1,718 and 1,375 cm−1 for C
O stretching and C–N stretching vibrations of the imide groups were observed. Elemental analysis measured values for C, H and N of the obtained polymer are in good agreement with the calculated values for bpy-PI. The number molecular weight (Mn) and molecular weight distribution (Mw/Mn) measured by GPC in THF using polystyrene as the standard were 9.3 × 103 and 1.51, respectively.
Synthesis of bpy-PI–Ru.
In general, when a polymer chain contains many ligand units, the inter-chain crosslinking through coordination with metal ions may occur, which decreases the solubility of metal-containing polymers. To avoid this kind of complexion, a Ru-complex, compound 6, was carefully selected, in which four coordination sites of ruthenium ion have been occupied by the bipyridine ligand of compound 1, 2,2′-bipyridine-4,4′-dicarboxydiethyl ester, and only two coordination sites were left to react with only one bipyridine unit in the polymer chain. Thus, the resulting polyimide containing bipyridine ruthenium complexes, bpy-PI–Ru, still have good solubility. Interestingly, the complexation of Ru complex compound 6 with the ligand polymer bpy-PI was found to be a stoichiometric reaction. From the elemental analysis results of bpy-PI–Ru, the content of C, H and N was 56.99, 3.55 and 7.09%, respectively, which are very close to the theoretical values for complete functionalization (57.08, 3.67 and 7.12%). That is to say, the bpy-PI–Ru molecule showed in Scheme 3 has an x value of 1 and a y value of 0.
Solubility of bpy-PI and bpy-PI–Ru.
The solubility of bpy-PI and bpy-PI–Ru (10 mg mL−1) was tested at room temperature in common organic solvents, and the results are listed in Table 1. Both bpy-PI and bpy-PI–Ru showed good solubility in polar aprotic solvents such as DMF, NMP, DMSO and DMAc, which can be attributed to the introduction of flexible linkages in the polymer main chain. The flexible moieties, the ether bonds and the hexafluoroisopropylidene groups (–C(CF3)2–), could increase the chain flexibility and the affinity of the polymer to polar solvents. Bpy-PI–Ru shows somewhat poorer solubility than bpy-PI, especially in solvents with a lower boiling point such as THF, CHCl3 and 1,2-dichloroethane (DCE).
Table 1 Solubility of bpy-PI and bpy-PI–Ru at room temperaturea
l |
Solubilityc |
Solventb |
Solubilityc |
bpy-PI |
bpy-PI–Ru |
|
bpy-PI |
bpy-PI–Ru |
Tested with polymer concentration of 10 mg mL−1.
NMP, N-methyl-2-pyrrolidone; DMSO, dimethyl sulfoxide; DMF, N,N-dimethylformamide; DMAc, N,N-dimethylacetamide; THF, tetrahydrofuran; TCE, 1,1,2,2-tetrachloroethane; DCE, dichloroethane.
Solubility: +, Soluble; −, insoluble; ±, partially soluble.
|
NMP |
+ |
+ |
CH2Cl2 |
+ |
± |
DMSO |
+ |
+ |
TCE |
+ |
+ |
DMF |
+ |
+ |
DCE |
+ |
− |
DMAc |
+ |
+ |
Pyridine |
+ |
+ |
THF |
+ |
± |
Nitromethane |
− |
− |
CHCl3 |
+ |
± |
Toluene |
− |
− |
TGA curves of bpy-PI, Ru-complex 6 and bpy-PI–Ru under N2 atmosphere are given in Fig. 2. Both bpy-PI and bpy-PI–Ru showed two stages of thermal decomposition. The first stage decomposition of bpy-PI started from 345 °C, which is probably due to the breakdown of the flexible segments, 1-ethoxy-4-methylbenzene, the weakest linkages along the polymer main chain. The second stage decomposition of bpy-PI started from 514 °C corresponding to the decomposition of the aromatic imido groups, which is typical for aromatic polyimides in general. Bpy-PI–Ru is less stable than its ligand polymer bpy-PI. The first onset decomposition temperature was found as 295 °C, which is quite close to that of the Ru complex, compound 6. We suggest that the decomposition at this temperature is due to the detachment of the Ru complex. The second decomposition onset temperature was 374 °C, which is also lower than that of the metal free polymer bpy-PI because of the presence of metal complexes. Despite the decrease in thermal stability, bpy-PI–Ru is thermally stable up to 295 °C, which can be considered stable enough for application in PSCs.
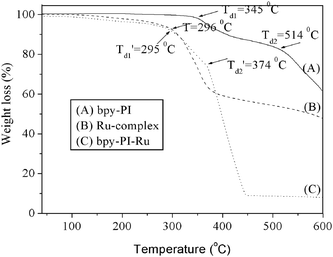 |
| Fig. 2 TG curves of bpy-PI, Ru-complex and bpy-PI–Ru in N2 atmosphere at a heating rate of 10.0 °C min−1. | |
The UV-vis absorption spectra of bpy-PI, Ru-complex compound 6 and bpy-PI–Ru were measured both in solution and in films, respectively. Fig. 3 shows the UV-vis absorption spectra of bpy-PI in DMF with different concentrations. In a given concentration, only one strong absorption band was observed, which is attributed to the ligand-centered (LC) π–π* transition of the 2,2′-bipyridine ligand. In addition, the absorption maximum was blue-shifted from 313 nm to 285 nm as the concentration of bpy-PI decreased from 1 mg mL−1 to 0.01 mg mL−1.
Fig. 4 shows the comparison of the UV-vis absorptions of bpy-PI, bpy-PI–Ru and compound 6 in DMF. Two similar absorption bands were observed for both compound 6 and bpy-PI–Ru at longer wavelengths in the visible region centered approximately at 435 and 593 nm, respectively, which are ascribable to the metal-to-ligand charge transfer (MLCT). Such absorptions owing to MLCT is regarded as the promotion of an electron from the metal-centered d orbital to an unfilled ligand-centered π* orbital. Besides, bpy-PI–Ru showed another absorption peaked at 692 nm, which is probably due to the aggregation of metal complex moieties. This evidence clearly demonstrated that the Ru complex moieties have been successfully incorporated onto the polymer main chain. Hence, the objective to enhance the photosensitivity of polyimide materials into the visible region has been realized through the introduction of Ru complexes.
Fig. 5 shows the comparison of UV-vis absorption spectra of bpy-PI and bpy-PI–Ru in thin films prepared by spin coating on a quartz glass of DMF solution (1 mg mL−1) at 2,500 rpm for 30 s at room temperature. Only two absorptions peaked at 226 and 274 nm were observed for bpy-PI, while three additional absorptions centered at 435, 598 and 698 nm were found for bpy-PI–Ru. Comparing with Fig. 4, all the absorptions in solution for bpy-PI and bpy-PI–Ru could be identified in films, while the spectra in the range 250–320 nm appeared quite different, which may originate from the presence of intra- and inter-chain charge transfer complexes (CTC) in film state.19
Photoluminescence properties
Photoluminescence spectra of the polymers and the Ru-complex compound 6 are shown in Fig. 6. Bpy-PI exhibited strong π*-π emission bands centered at approximately 431 nm, while the Ru-complex compound 6 showed very weak fluorescence at approximately 357 nm. The incorporation of ruthenium complex into bpy-PI induced a severe change in photoluminescence, emissions from the polymer chain in bpy-PI–Ru were quenched significantly and blue-shifted for 42 nm as compared with that in bpy-PI. This may be due to the presence of an energy transfer process from the main chain to the ruthenium complex with low-lying energy levels, which may facilitate the sensitization broadening in visible region.
Electrochemical properties
The HOMO and LUMO levels of bpy-PI, compound 6 and bpy-PI–Ru were estimated by cyclic voltammetry (CV) at room temperature. Glass carbon electrode (ϕ = 4 mm), saturated calomel electrode (SCE) and Pt wire were used as the working, the reference and the counter electrodes, respectively. Redox processes of the complex and polymers in DMF were performed using Bu4NCl4 (0.1 M) as a supporting electrolyte. As shown in Fig. 7, compound 6 showed two couples of distinct reversible oxidation peaks, and the onset of oxidation and reduction potentials were estimated as 0.33 V and −0.52 V (vs. SCE), respectively. While, both bpy-PI and bpy-PI–Ru showed similar CV curves except for the difference in the onset of oxidation potential. For bpy-PI–Ru, one oxidation was reversible and the other was irreversible. The onset oxidation of bpy-PI–Ru started at around 0.68 V (vs. SCE), which was lower than that of bpy-PI (around 0.74 V), and the onset reduction potential was similar as approximately −0.67 V. These information suggested that an active species was formed in the chelation process of bpy-PI with the Ru complex.20 The energy levels of HOMO (EHOMO) of bpy-PI, compound 6 and bpy-PI–Ru were estimated as −5.48, −5.07 and −5.42 eV, respectively, from the onset of oxidation according to an empirical formula, EHOMO = −(Eonox + 4.74) (eV).21 The energy levels of LUMO (ELUMO) of bpy-PI, compound 6 and bpy-PI–Ru were estimated as −4.07, −4.22 and −4.07 eV, respectively, from the onset of reduction according to an empirical formula, ELUMO = −(Eonred + 4.74) (eV).21 These results suggested that the introduction of Ru-complexes made the polymer bpy-PI–Ru easier to oxidize than the ligand polymer bpy-PI.
 |
| Fig. 7 CV curves of bpy-PI, Ru-complex and bpy-PI–Ru in 0.1 M TBAP DMF solution at 100 mV s−1 scan rate. | |
Photovolatic behavior of Bpy-PI–Ru
Photovoltaic cells were fabricated by using bpy-PI–Ru as the active layer, their J-V curves are illustrated in Fig. 8 and the corresponding open circuit voltage (VOC), short-circuit current (JSC), fill factor (FF) and energy conversion efficiency (ηe) are collected in Table 2. Device 1 exhibited a higher VOC (0.52 V), a lower JSC (0.0015 mA cm−2) and FF (0.28). The lower JSC may result from the molecular internal lower carrier mobility of polyimide, and the lower FF may be induced by high series resistance or by small shunt resistance. Compared with device 1, the JSC and ηe of device 3 based on a blend of bpy-PI–Ru and PCBM (1
:
2, w/w) were increased by one order of magnitude and the FF was increased to 0.38, which may be derived from the contribution of the higher charge mobility of PCBM dopant. These results suggested that an interpenetrating network structure has been formed between bpy-PI–Ru and PCBM in device 3, which may facilitate the separation of excitons generated from the ruthenium complexes upon shining by light. However, the VOC was decreased to 0.19 V, which may result from the lower LUMO level of bpy-PI–Ru than that of PCBM.22 Based on the results of devices 1 and 3, a thin layer of PEDOT:PSS was deposited between the ITO electrode and the photoactive layer (devices 2 and 4), since a blend of PEDOT:PSS is commonly used for modification of polymer-electrode interface in various opto-electronic devices.23 The JSC and ηe were clearly enhanced in comparison with those of devices 1 and 3, respectively, because the potential barrier of hole injection was lowered and the injection and transport of holes and electrons were balanced by the PEDOT:PSS layer inserted between the anode and the polymer active layer. However, the VOC of device 2 (0.33 V) was lower than that of device 1, while the VOC of device 4 (0.31 V) was higher than that of device 3. These may be due to the alignment of the polymer HOMO level with the work function of ITO. Meanwhile, no significant improvement in FFs was observed. All the results indicated that the performance of photovoltaic device from bpy-PI–Ru may be improved to some extent through dopant introduction and anode modification. However, there is still a lot of room for device improvement from the viewpoint of practical application, which needs our further effort in materials design.
Table 2 Properties of photovoltaic devices fabricated with bpy-PI–Ru illuminated under a compact white arc lamp
Cell structure |
V
OC
/V |
J
SC
/mA cm−2 |
FF |
ηe (%) |
(1) ITO/bpy-PI–Ru/Al |
0.52 |
0.0015 |
0.28 |
4.01 × 10−4 |
(2) ITO/PEDOT:PSS/bpy-PI–Ru/Al |
0.33 |
0.0042 |
0.28 |
7.06 × 10−4 |
(3) ITO/bpy-PI–Ru:PCBM(1 : 2)/Al |
0.19 |
0.021 |
0.38 |
2.76 × 10−3 |
(4) ITO/PEDOT:PSS/bpy-PI–Ru:PCBM(1 : 2)/Al |
0.31 |
0.038 |
0.22 |
4.71 × 10−3 |
 |
| Fig. 8 Current density-voltage (J-V) curves of devices with different structure from bpy-PI–Ru under dark and illumination. | |
Conclusions
A novel bipyridine-containing diamine monomer, 4,4′-bis((4-aminophenoxy)-methyl)-2,2′-bipyridine, was designed and synthesized. A polyimide containing bipyridine ligands, bpy-PI, was prepared by polycondensation of the bipyridine-containing diamine monomer with fluoro-containing dianhydride 6FDA. A polyimide containing Ru complexes, bpy-PI–Ru, was successfully prepared through complexation of bpy-PI as the ligand polymer with a Ru complex compound. Both bpy-PI and bpy-PI–Ru have good thermal stability, and good solubility in common organic solvents, such as DMF, NMP, DMSO and DMAc. Bpy-PI–Ru showed strong UV-vis absorptions in the visible region due to the presence of the Ru complex. Emissions from the polymer main chain in bpy-PI–Ru were quenched by the Ru complex, which suggests the presence of energy transfer processes from polymer main chain to Ru-complex. The onset oxidation potential of bpy-PI–Ru is 0.68 V (vs. SCE), which is lower than that of bpy-PI, indicating that bpy-PI–Ru is easier to oxidize. Polymer photovoltaic cells were fabricated by using bpy-PI–Ru as the photoactive material. These simple devices showed maximum JSC and ηe of 0.038 mA cm−2 and 4.71 × 10−3 (%), respectively. Optimization in device structure and molecular design will be a part of our future work for the improvement of device performance with materials combining polyimide and ruthenium complexes.
Acknowledgements
We are grateful to the National Natural Science Foundation of China for the financial support (20604025, 20874091).
References
- A. Hagfeldt and M. Grätzel, Acc. Chem. Res., 2000, 33, 269–277 CrossRef CAS; M. Grätzel, Nature, 2001, 414, 338–344 CrossRef CAS; W. K. Chan, Coord. Chem. Rev., 2007, 1–15; Y. y. Liang, Y. Wu, D. Q. Feng, S. T. Tsai, H. J. Son, G. Li and L. P Yu, J. Am. Chem. Soc., 2009, 131, 56–57 CrossRef CAS.
- J. Y. Kim, K. Lee, N. E. Coates, D. Moses, T. Q. Nguyen, M. Dante and A. J. Heeger, Science, 2007, 317, 222–225 CrossRef CAS; Y. Y. Liang, D. Q. Feng, Y. Wu, S. T. Tsai, G. Li, C. Ray and L. P. Yu, J. Am. Chem. Soc., 2009, 131, 7792–7799 CrossRef CAS.
- K. M. Coakley and M. D. McGehee, Chem. Mater., 2004, 16, 4533–4542 CrossRef CAS.
- J. H. Hou, M. H. Park, S. Q. Zhang, Y. Yao, L. M. Chen, J. H. Li and Y. Yang, Macromolecules, 2008, 41, 6012–6018 CrossRef CAS; Y. F. Li and Y. P. Zou, Adv. Mater., 2008, 20, 2952–2958 CrossRef CAS; J. W. Chen and Y. Cao, Acc. Chem. Res., 2009, 42, 1709–1718 CrossRef CAS.
- B. O’Regan and M. Grätzel, Nature, 1991, 353, 737–740 CrossRef CAS.
- M. K. Nazeeruddin, A. Key, I. Rodicio, R. Humphry-Barker, E. Müller, P. Liska, N. Vlachopoulous and M. Grätzel, J. Am. Chem. Soc., 1993, 115, 6382–6390 CrossRef CAS; M. K. Nazeeruddin, S. M. Zakeeruddin, R. Humphry-Baker, M. Jirousek, P. Liska, N. Vlachopoulos, V. Shklover, C. H. Fischer and M. Grätzel, Inorg. Chem., 1999, 38, 6298–6305 CrossRef CAS.
- M. Grätzel, J. Photochem. Photobiol., C, 2003, 4, 145–153 CrossRef CAS; M. Grätzel, Inorg. Chem., 2005, 44, 6841–6851 CrossRef; M. Grätzel, Progr. Photovolt.: Res. Appl., 2006, 14, 429–442 Search PubMed; H. N. Tian, X. C. Yang, R. K. Chen, R. Zhang, A. Hagfeldt and L. C. Sun, J. Phys. Chem. C, 2008, 112, 11023–11033 CrossRef CAS.
- P. K. Ng, X. Gong, S. H. Chan, L. S. M. Lam and W. K. Chan, Chem.–Eur. J., 2001, 7, 4358–4367 CrossRef CAS; K. Y. K. Man, H. L. Wong, W. K. Chan, C. Y. K. wong and A. B. Djurišić, Chem. Mater., 2004, 16, 365–367 CrossRef CAS; K. K. Y. Man, H. L. Wong, W. K. Chan, A. B. Djurišić, E. Beach and S. Rozeveld, Langmuir, 2006, 22, 3368–3375 CrossRef CAS.
- C. W. Tse, L. S. M. Lam, K. Y. K. Man, W. T. Wong and W. K. Chan, J. Polym. Sci., Part A: Polym. Chem., 2005, 43, 1292–1308 CrossRef CAS; K. W. Cheng, C. S. C. Mak, W. K. Chan, A. M. C. Ng and A. B. Djurišić, J. Polym. Sci., Part A: Polym. Chem., 2008, 46, 1305–1317 CrossRef CAS.
- W. Y. Wong, X. Z. Wang, Z. He, A. B. Djurišić, C. T. Yip, K. Y. Cheung, H. Wang, C. S. K. Mak and W. K. Chan, Nat. Mater., 2007, 6, 521–527 CrossRef CAS.
- B. V. Kotov, Russ. J. Phys. Chem., 1988, 62, 1408–1417.
- W. J. Li, H. Osora, L. Otero, D. C. Duncan and M. A. Fox, J. Phys. Chem. A, 1998, 102, 5333–5340 CrossRef CAS; D. Mühlbacher, C. J. Brabee, N. S. Sariciftci, B. V. Kotov, V. I. Berendyaev, B. M. Rumyantsev and J. C. Hummelen, Synth. Met., 2001, 121, 1609–1610 CrossRef CAS; B. V. Kotov, B. M. Rumyantsev, V. I. Berendyaev, E. V. Lunina, B. P. Bespalov, E. L. Frankevich and M. M. Triebel, Synth. Met., 2001, 121, 1553–1554 CrossRef CAS; H. J. Niu, X. D. Bai, Y. D. Huang and C. Wang, Chin. J. Chem., 2005, 63, 1391–1396 CAS.
- G. S. Liou, S. HueiI, Hsiao, M. Iishida, M. Kakimoto and Y. Imai, J. Polym. Sci., Part A: Polym. Chem., 2002, 40, 3815–3822 CrossRef CAS.
- S. G. Sun, X. J. Peng, Y. Q. Xu, Y. L. Gao and L. C. Sun, Chem. J. Chinese U., 2004, 25(1), 67–70 Search PubMed.
- I. Gillaizeau-Gauthier, F. Odobel, M. Alebbi, R. Argazzi, E. Costa, C. A. Bignozzi, P. Qu and G. J. Meyer, Inorg. Chem., 2001, 40, 6073–6079 CrossRef CAS.
- M. J. Jeong, J. H. Park, C. J. Lee and J. Y. Chang, Org. Lett., 2006, 8, 2221–2224 CrossRef CAS; S. E. Velu, C. H. Luan, L. J. Delucas, C. G. Brouillette and W. J. Brouillette, J. Comb. Chem., 2005, 7, 898–904 CrossRef CAS.
- W. J. Kuo, M. C. Chang, T. Y. Juang, C. P. Chen, C. T. Chen, H. L. Chang and R. J. Jeng, Polym. Adv. Technol., 2005, 16, 515–523 CrossRef CAS; S. H. Cheng, S. H. Hsiao, T. H. Su and G. S. Liou, Macromolecules, 2005, 38, 307–316 CrossRef CAS.
- S. C. Yu, X. Gong and W. K. Chan, Macromolecules, 1998, 31, 5639–5646 CrossRef CAS; P. Wang, S. M. Zakeeruddin, J. E. Moser, M. K. Nazeeruddin, T. Sekiguchi and M. Grätzel, Nat. Mater., 2003, 2, 402–407 CrossRef CAS.
- M. Hasegawa and K. Horie, Prog. Polym. Sci., 2001, 26, 259–335 CrossRef CAS.
- W. M. Xue, W. J. Perez and D. P. Rillema, Inorg. Chim. Acta, 1999, 114–126, 296; V. Marin, E. Holder, R. hoogenboom and U. S. Schubert, J. Polym. Sci., Part A: Polym. Chem., 2004, 42, 4153–4160 CrossRef CAS.
- S. Toriu, T. Kaneko, H. Nishide and E. Tsuchida, Polym. Adv. Technol., 1995, 6, 707–710 CrossRef CAS.
- J. H. Hou, C. H. Yang, J. Qiao and Y. F. Li, Synth. Met., 2005, 150, 297–304 CrossRef CAS.
- L. Groenendaal, F. Jonas, D. Freitag, H. Pielartzik and J. R. Reynolds, Adv. Mater., 2000, 12, 481–494 CrossRef CAS; M. K. Fung, S. L. Lai, S. W. Tong, M. Y. Chan, C. S. Lee and S. T. Lee, Appl. Phys. Lett., 2002, 81(8), 1497–1499 CrossRef CAS; A. M. Nardes, M. Kemerink, R. A. J. Janssen, J. A. M. Bastiaansen, N. M. M. Kiggen, B. M. W. Langeveld, A. J. J. M. van Breemen and M. M. de Kok, Adv. Mater., 2007, 19, 1196–1200 CrossRef CAS.
|
This journal is © The Royal Society of Chemistry 2010 |
Click here to see how this site uses Cookies. View our privacy policy here.