Bis-cation salt complexation by meso-octamethylcalix[4]pyrrole: linking complexes in solution and in the solid state†
Received
5th August 2009
, Accepted 7th October 2009
First published on 4th November 2009
Abstract
Pyridinium and imidazolium bis-cations are shown to link calix[4]pyrrole anion complexes both in solution and in the solid state. This is accomplished by binding of the bis-cations to the electron-rich bowl shaped cavities formed by two separate calixpyrrole–anion complexes. These resulting sandwich-type structures provide a new way of organising calix[4]pyrrole anion complexes in space.
Introduction
The development of ditopic ion-pair receptors has attracted intense interest recently.1 Calix[4]pyrroles are tetrapyrrole macrocycles first synthesised by Baeyer in 18862via the acid catalysed condensation of pyrrole and a ketone. In 1996, we reported that meso-octamethylcalix[4]pyrrole 1 forms complexes with anionic species in solution and in the solid state.3 Subsequent studies have shown that members of this class of macrocycles can function both as anion sensors and anion separation agents.4 In 2005, we discovered that calix[4]pyrroles can bind ion pairs such as caesium or 1,3-dialkylimidazolium halide salts with the anion bound to the pyrrole NH groups, locking the macrocycle into a cone conformation, and the large charge diffuse cation binding to the electron rich bowl-shaped cavity formed by the calix[4]pyrrole anion complex.5 Subsequent studies in solution with a variety of salts have demonstrated a significant dependence of anion stability constants on both the nature of the solvent and cation used indicating that complexation cannot be regarded as a simple 1
:
1 (anion
:
calix[4]pyrrole) process but rather that the solvent and cation are intimately involved in the binding processes. This hypothesis is supported by evidence from a variety of solid-state structures showing cation inclusion in the calix[4]pyrrole cup.6 It has also been shown that calixpyrroles can extract metal salts from aqueous solution7 and transport caesium chloride salts across lipid bilayer membranes.8
We decided to investigate whether we could use cation complexation as a means of ordering these species relative to one another as a first step towards producing ordered arrays of calixpyrrole ion-pair complexes. We therefore synthesised a series of bis-cation dibromide salts consisting of either two pyridinium or imidazolium groups linked via an alkyl chain (i.e.2–5) and studied whether the bis-cation could be used to link calix[4]pyrrole anion complexes both in solution and the solid state.
Results and discussion
meso-Octamethylcalix[4]pyrrole 1,3 and the pyridinium and imidazolium bis-cation salts 2–5 were synthesised via literature procedures.9
Solid state studies
Crystals of the 1,1′-(butane-1,4-diyl)bis(pyridin-1-ium) bromide 2 complex of meso-octamethylcalix[4]pyrrole 1 were obtained by slow evaporation of a 1
:
1 dichloromethane–ethanol solution of the calixpyrrole in the presence of excess bromide salt. The structure of 12·2 was elucidated by single crystal X-ray diffraction and reveals that the calix[4]pyrrole adopts the cone conformation binding bromide via four NH⋯Br− hydrogen bonds in the range 3.494(2)–3.540(2) Å (Fig. 1). The pyridinium groups of the bis-cation each reside in the bowl shaped calixpyrrole-anion complex cavity (which are equivalent by symmetry). The distance between carbon atoms C30 and C31 on the pyridinium ring and the centroid of the closest pyrrole ring (C15 C16 C17 C18 N3) are 3.531 and 3.796 Å respectively (see ESI for more details).
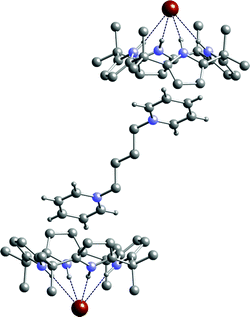 |
| Fig. 1 The X-ray crystal structure of the complex 12·2. Selected hydrogen atoms and dichloromethane solvent molecules have been omitted for clarity. An inversion centre lies at the midpoint of the central bond of the cation. | |
Crystals of the 1,1′-(hexane-1,6-diyl)bis(pyridin-1-ium) bromide 3 complex of meso-octamethylcalix[4]pyrrole 1 were obtained by slow evaporation of a 1
:
1 dichloromethane–ethanol solution of the calixpyrrole in the presence of excess bromide salt. The structure of 12·3 was elucidated by single crystal X-ray diffraction and reveals again that the calix[4]pyrrole adopts the cone conformation binding bromide via four NH⋯Br− hydrogen bonds in the range 3.438(3)–3.503(3) Å (Fig. 2). As was observed in the structure of 12·2, each pyridinium group resides in the bowl shaped cavity of the calix[4]pyrrole anion complex. The distance between carbon atoms C31 and C32 on the pyridinium ring and the centroid of the closest pyrrole ring (C1 C2 C3 C4 N1) are 3.640 and 3.679 Å, respectively (see ESI for more details).
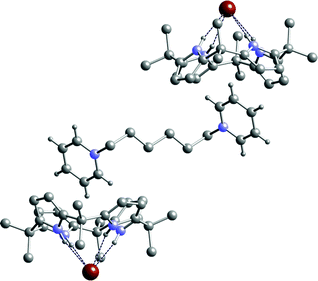 |
| Fig. 2 The X-ray crystal structure of the complex 12·3. Selected hydrogen atoms have been omitted for clarity. An inversion centre lies at the midpoint of the central bond of the cation. | |
Crystals of the 3,3′-(hexane-1,6-diyl)bis(1-methyl-1H-imidazol-3-ium) bromide 5 complex of meso-octamethylcalix[4]pyrrole 1 were obtained by slow evaporation of a solution of the receptor in 1
:
1 acetonitrile–ethanol solution in the presence of excess bromide salt. The structure of 12·3 was elucidated by single crystal X-ray diffraction and reveals again that the calix[4]pyrrole adopts the cone conformation binding bromide via four NH⋯Br− hydrogen bonds in the range 3.410(4)–3.500(4) Å (Fig. 3). The distance between carbon atoms C30 in the imidazolium ring and the centroid of the closest pyrrole ring (C1 C2 C3 C4 N1) is 3.537 Å (see ESI for more details).
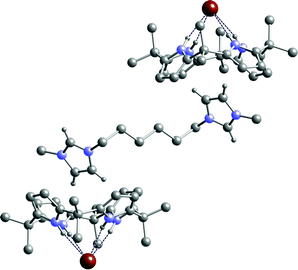 |
| Fig. 3 The X-ray crystal structure of the complex 12·5. Selected hydrogen atoms have been omitted for clarity. An inversion centre lies at the midpoint of the central bond of the cation. | |
1H NMR spectroscopic analyses
Proton NMR experiments were conducted with bis-imidazolium bromide 5 and compound 1 in acetonitrile-d3. Compound 1 was titrated into a solution of the salt and the resulting NMR spectra are shown in Fig. 4. The results show that in the presence of increasing quantities of the macrocycle, the imidazolium CH protons become shielded and move to lower field (e.g., proton originally resonating at ca. 9.41 ppm). Such a finding is consistent with, but not proof of, inclusion of the imidazolium cation in the bromide-induced calix[4]pyrrole cup-shaped cavity. While we favour such an interpretation, which is accord with the X-ray structural analyses discussed above, it is important to appreciate that the observed shielding effect may be due to sequestration of the bromide anion by the calixpyrrole.
In an attempt to quantify the energetics associated with calixpyrrole
:
bis-cation complex formation, we turned to ITC. Unfortunately the bis-pyridinium salts (2 and 3) were not sufficiently soluble in acetonitrile or dichloromethane to allow for ITC analyses in these solvents. When titrations were attempted in DMSO a very weak interaction was observed (Ka < 102 M−1), as would be expected from the previous reports on chloride binding in DMSO and bromide binding in dichloromethane.3,6
The bis-imidazolium salts 4 and 5 were sufficiently soluble in acetonitrile to permit ITC titrations. Representative titrations are shown below in Fig. 5. From the position of the inflection point (ca. 0.5 molar ratio; see Fig. 5) we infer that the binding stoichiometry of the interaction is 1
:
2 (bisimidazolium salt
:
calixpyrrole). When fit to a sequential binding site model (i.e., defining the salt as having two independent binding sites) a good fit is obtained (see Table S1 in the ESI for complete thermodynamic data).
![Left: one-site binding fit of 1,4-bis-(3-methylimidazolium)butane dibromide salt: 4 [30.9 mM effective] titrated into 1 [3.3 mM] and right: one-site binding fit of 1,4-bis-(3-methylimidazolium)hexane dibromide salt 5 [45.3 mM effective] titrated into calix[4]pyrrole 1 [2.9 mM].](/image/article/2010/OB/b916113a/b916113a-f5.gif) |
| Fig. 5 Left: one-site binding fit of 1,4-bis-(3-methylimidazolium)butane dibromide salt: 4 [30.9 mM effective] titrated into 1 [3.3 mM] and right: one-site binding fit of 1,4-bis-(3-methylimidazolium)hexane dibromide salt 5 [45.3 mM effective] titrated into calix[4]pyrrole 1 [2.9 mM]. | |
For comparison, the data obtained for bis-imidazolium salts 4 and 5 was also fitted to a one site binding equation. This model can give an accurate representation of multiple binding sites provided the sites are identical to one another. Again a good fit is obtained using this method, and the data is summarized in Table 1 (see ESI for complete thermodynamic data). This data reveals that within experimental error there are no differences in the energetics of binding for studies involving the linked species 4 and 5.
Salt |
N
a
|
n
a
|
ΔΓ/kcal mol−1 |
ΔH/kcal mol−1 |
TΔS/kcal mol−1 |
K
a/M−1 |
N = number of independent titrations, n = stoichiometry at equivalence point (salt : calix[4]pyrrole).
Data from NMR titration in dichloromethane-d2.6d
Data obtained at 303 K.6e
Data from reference.6f Adjusted table to include n-value from the ITC titrations. Also multiplied the values for the one site data by the n value so they can be compared directly to the interactions with the mono-imidazole salts.
|
4
|
6 |
0.502 ± 0.004 |
−2.44 ± 0.05 |
−7.74 ± 0.35 |
−5.30 ± 0.34 |
1790 ± 320 |
5
|
5 |
0.486 ± 0.012 |
−2.42 ± 0.03 |
−7.75 ± 0.61 |
−5.32 ± 0.59 |
2220 ± 230 |
BMIMBr |
|
1.01 |
−4.83 |
−7.99 |
−3.16 |
3500 |
BMIMBrb |
|
|
— |
— |
— |
280 |
BMIMCl |
|
0.91 |
−6.67 |
−10.51 |
−3.84 |
75 000 |
BMIMClb |
|
|
— |
— |
— |
6000 |
TBABrc |
|
|
— |
— |
— |
3400 |
TBACld |
|
|
−7.29 |
−10.16 |
−2.91 |
22 000 |
As a control study, designed to probe the effect that tethering two imidazolium salts has on the binding energetics, the mono-imidazolium salts, 1-butyl-3-methyl imidazolium chloride (BMIMCl) and 1-butyl-3-methyl imidazolium bromide (BMIMBr), were investigated by ITC in acetonitrile at similar concentrations as used above. In these cases the inflection point occurs near a molar ratio of 1.0, a finding that is consistent with a 1
:
1 (1:BMIM salt) binding interaction. This behavior is also in accord with previous data6 obtained in dichloromethane (see Table 1 and Fig. 6).
![ITC heat signatures and associated binding curves obtained when BMIMCl [31.0 mM] is titrated into 1 [3.3 mM] (left) and BMIMBr [37.8 mM] titrated into 1 [3.5 mM] (right).](/image/article/2010/OB/b916113a/b916113a-f6.gif) |
| Fig. 6 ITC heat signatures and associated binding curves obtained when BMIMCl [31.0 mM] is titrated into 1 [3.3 mM] (left) and BMIMBr [37.8 mM] titrated into 1 [3.5 mM] (right). | |
Conclusions
Equations predicated on first a sequential binding interaction and then a single set of binding sites were both used to fit these data for the complexation of bisimidazolium salts 4 and 5 with calix[4]pyrrole. The results obtained allowed some limited conclusions about the complexation process in solution to be drawn. For example, the position of the inflection point can be indicative of the binding stoichiometry, which in the case of the bisimidazolium salts was found to occur around a molar ratio of 0.5. Such a molar ratio is expected for a 2
:
1 (calixpyrrole
:
guest) binding interaction. In contrast, the mono-imidazolium salts BMIMCl and BMIMBr were found to interact with calix[4]pyrrole in a 1
:
1 binding stoichiometry.6 Although the nature of the interaction is not fully defined, the solution data are consistent with the notion that binding of the bisimidazolium salts occurs such that a 1
:
2 salt
:
calixpyrrole complex forms wherein the cationic portions of the two-component substrate are bound within the calixpyrrole cup. Such an inference is fully supported by the single crystal X-ray diffraction analyses, which reveal the inclusion of the cation into the calixpyrrole cup and the formation of a 5-membered anion-calixpyrrole-biscation-calixpyrrole-anion supramolecular ensemble in the solid state.
Acknowledgements
PAG thanks the EPSRC for funding and for access to the crystallographic facilities at the University of Southampton. JLS thanks the National Institutes of Health (GM 58907) for financial support. CC would like to thank Italian Ministero dell'Istruzione, dell'Università e della Ricerca Scientifica (MIUR) for financial support (Project PRIN-2007C8RW53).
Notes and references
- For recent contributions and reviews see for example:
(a) C. Caltagirone and P. A. Gale, Chem. Soc. Rev., 2009, 38, 520–563 RSC;
(b) M. D. Lankshear, I. M. Dudley, K.-M. Chan, A. R. Cowley, S. M. Santos, V. Felix and P. D. Beer, Chem.–Eur. J., 2008, 14, 2248–2263 CrossRef CAS;
(c) P. A. Gale, S.E. García-Garrido and J. Garric, Chem. Soc. Rev., 2008, 37, 151–190 RSC;
(d) M. Cametti, M. Nissinen, A. Dalla Cort, L. Mandolini and K. Rissanen, J. Am. Chem. Soc., 2007, 129, 3641–3648 CrossRef CAS;
(e) J. M. Mahoney, K. A. Stucker, H. Jiang, I. Carmichael, N. R. Brinkmann, A. M. Beatty, B. C. Noll and B. D. Smith, J. Am. Chem. Soc., 2005, 127, 2922–2928 CrossRef CAS;
(f) F. Oton, A. Tarraga, A. Espinosa, M. D. Velasco and P. Molina, Dalton Trans., 2006, 3685–3692 RSC;
(g)
J. L. Sessler, P. A. Gale and W. S. Cho, Synthetic Anion Receptor Chemistry, Royal Society of Chemistry: London, 2006, Chapter 6, pp 259–293. Search PubMed;
(h)
B. D. Smith, in Macrocyclic Chemistry: Current Trends and Future Perspectives, ed. K. Gloe, Springer: Dordrecht, 2005, pp 137–151 Search PubMed;
(i) G. J. Kirkovits, J. A. Shriver, P.A. Gale and J. L. Sessler, J. Inclusion Phenom. Macrocyclic Chem., 2001, 41, 69–75 CrossRef CAS.
- A. Baeyer, Ber. Dtsch. Chem. Ges., 1886, 19, 2184–2185 CrossRef.
- P. A. Gale, J. L. Sessler, V. Král and V. Lynch, J. Am. Chem. Soc., 1996, 118, 5140–5141 CrossRef CAS.
-
(a) J. L. Sessler, P. Anzenbacher Jr., H. Miyaji, K. Jursikova, E.R. Bleasdale and P. A. Gale, Ind. Eng. Chem. Res., 2000, 39, 3471–3478 CrossRef CAS;
(b) J. L. Sessler, A. Gebauer and P. A. Gale, Gazz. Chim. Ital., 1997, 127, 723–726 CAS;
(c) J. L. Sessler, P.A. Gale and J. W. Genge, Chem.–Eur. J., 1998, 4, 1095–1099 CrossRef CAS.
- R. Custelcean, L. H. Delmau, B. A. Moyer, J. L. Sessler, W.-S. Cho, D. E. Gross, G. W. Bates, S. J. Brooks, M. E. Light and P. A. Gale, Angew. Chem., Int. Ed., 2005, 44, 2537–2542 CrossRef CAS.
-
(a) J. L. Sessler, D. E. Gross, W.-S. Cho, V. M. Lynch, F. P. Schmidtchen, G. W. Bates, M. E. Light and P. A. Gale, J. Am. Chem. Soc., 2006, 128, 12281–12288 CrossRef CAS;
(b) G. W. Bates, P. A. Gale and M. E. Light, CrystEngComm, 2006, 8, 300–302 RSC;
(c) D. E. Gross, F. P. Schmidtchen, W. Antonius, P. A. Gale, V.M. Lynch and J. L. Sessler, Chem.–Eur. J., 2008, 14, 7822–7827 CrossRef CAS;
(d) G. W. Bates, P. A. Gale and M. E. Light, Supramol. Chem., 2008, 20, 23–28 CrossRef CAS;
(e) C.-H. Lee, J. S. Lee, H. K. Na, D. W. Yoon, H. Miyaji, W. S. Cho and J. L. Sessler, J. Org. Chem., 2005, 70, 2067–2074 CrossRef CAS;
(f) D. W. Yoon, D. E. Gross, V. M. Lynch, J. L. Sessler, B. P. Hay and C. H. Lee, Angew. Chem., Int. Ed., 2008, 47, 5038–5042 CrossRef CAS.
-
(a) M. P. Wintergerst, T. G. Levitskaia, B. A. Moyer, J. L. Sessler and L. H. Delmau, J. Am. Chem. Soc., 2008, 130, 4129–4139 CrossRef CAS; For an examples of calix[4]pyrrole decorated polymers see:
(b) A. Aydogan, D. J. Coady, V. M. Lynch, A. Akar, M. Marquez, C. W. Bielawski and J. L. Sessler, Chem. Commun., 2008, 1455–1457 RSC;
(c) A. Aydogan, D. J. Coady, S. K. Kim, A. Akar, C. W. Bielawski, M. Marquez and J. L. Sessler, Angew. Chem., Int. Ed., 2008, 47, 9648–9652 CrossRef CAS;
(d) G. V. Zyryanov, T.H. Kinstle and P. Anzenbacher Jr., Syn. Lett., 2008, 1171–1174 CAS.
-
(a) M. G. Fisher, P. A. Gale, J. R. Hiscock, M. B. Hursthouse, M. E. Light, F.P. Schmidtchen and C. C. Tong, Chem. Commun., 2009, 3017–3019 RSC;
(b) C. C. Tong, R. Quesada, J.L. Sessler and P. A. Gale, Chem. Commun., 2008, 6321–6323 RSC.
- R. Hazard, J. Cheymol, J. A. Gautier, E. Corteggiani and E. Leroi, Arch. Int. Pharm., 1952, 90, 271–275 Search PubMed ; See also R.E. Lyle and J. J. Gardikes, J. Am. Chem. Soc., 1955, 77, 1291–1292 Search PubMed; V.V. Namboodiri and R. S. Varna, Org. Lett., 2002, 4, 3161–3163 CrossRef CAS.
|
This journal is © The Royal Society of Chemistry 2010 |