DOI:
10.1039/C003515G
(Communication)
Metallomics, 2010,
2, 530-534
Received
23rd February 2010
, Accepted 26th May 2010
First published on
8th June 2010
2-Aminophenoxazine-3-one is a naturally occurring heterocycle and a core part of the well-known polypeptide antibiotic Actinomycin D, the first antibiotic shown to have anticancer activity.1 Naturally occurring Actinomycin D inhibits DNA-directed RNA synthesis2 and is used in the treatment of a variety of cancers.3 The final step of the biosynthesis of Actinomycin D involves the oxidative coupling of o-aminophenol and is catalysed by Phenoxazinone synthase, a laccase member of the blue copper oxidases, Scheme 1.4
In addition to Actinomycin D many other natural products also incorporate the 2-aminophenoxazine-3-one structure including those found in insect pigments, fungal metabolites, antibiotics and various allelochemicals.5 It is therefore not surprising that many studies have demonstrated the good biological activity of 2-aminophenoxazine-3-one itself, which has a demonstrated ability to intercalate with DNA6 and has been shown to induce apoptosis in the human myeloma cell line U2667 and to inhibit proliferation of mouse malignant melanoma B16 cells in vitro.8 It has also been suggested that it can inhibit the expression of angiogenic factors such as myosin heavy chain (SMemb), endothelin-1 (ET-1) and plasminogen activator inhibitor-1 (PAI-1), thereby preventing vascular angiogenesis in diabetic patients.9 It also has anti-inflammatory properties due to its demonstrated ability to inhibit the pro-inflammatory mediators nitric oxide and prostaglandin E2.10
Reports of antimicrobial activity are conflicting: Shimazu clearly demonstrated the activity of 2-aminophenoxazine-3-one towards four strains of mycobacteria using the McFarland method but (using this method) concluded that it was inactive towards Gram(−) E. coli and Gram(+) S. aureus.11 Using a diffusion method Zeeck et al also concluded that 2-aminophenoxazine-3-one is inactive toward E. coli but in contrast to Shimazu found activity towards S. aureus.12
To date no metal complexes of 2-aminophenoxazine-3-one have been reported and given the good potential for coordination through the ring and/or terminal nitrogen donors we considered it an excellent candidate for coordination to soft metal ions such as the coinage metals. As silver(I) complexes are potent antimicrobial agents in their own right,13,14 and the ion is coordinatively and electronically non-demanding, silver was selected as the metal of choice. We now report the synthesis of the first metal complex of this phenoxazinone, its solid state structure and its activity towards Gram(+) S. aureus and Gram(−) E. coli. We also report a new high-yielding synthetic route to 2-aminophenoxazine-3-one using 2,5-dichloro-1,4-benzoquinone (p-chloranil) as oxidant.
The synthesis of 2-aminophenoxazine-3-one has been shown to proceed via a cascade of three consecutive two electron oxidations although only the first 2e- oxidation is laccase-catalysed.4 The o-aminophenol is initially oxidised to a quinone imine intermediate which undergoes a conjugate addition with a second o-aminophenol at the enzyme active site. The intermediate is then oxidised to a p-quinoneimine and a final 2e- oxidation completes the ring closure. Since this mechanism was elucidated new reports of synthetic routes to 2-aminophenoxazine-3-one by chemical means have continued to appear. Whilst some of these use non-metallic enzymatic or chemical routes,15 others use transition metal mimics of the natural enzyme such as cobalt(II),16 copper(II),17 manganese(II)18 or manganese(IV)19 or employ heavy metal catalysts20 such as lead(IV) or mercury(II), precious metal catalysts and dioxygen at elevated temperatures,21 or expensive radical initiators.22 In contrast to these we report here the high yielding and economical synthesis of 2-aminophenoxazine-3-one by stoichiometric oxidation with 2,5-dichloro-1,4-benzoquinone.
This work is part of a wider study of the biological activity of redox active 1,4-benzoquinone ligands, as many naturally occurring quinones possess antioxidant, anti-inflammatory, and antitumour properties.23 As part of this work we attempted to synthesise 2,5-bis(2-hydroxyphenylamino)-3,6-dichlorocyclohexa -2,5-diene-1,4-dione,241, by reacting o-aminophenol with p-chloranil under aerobic conditions in ethanol, Scheme 2a. Instead the reaction yielded 2-aminophenoxazine-3-one and 2,3,5,6-tetrachloro-1,4-hydroquinone, Scheme 2b, which co-crystallised in a 2
:
3 ratio and which were structurally characterised.
We investigated the possibility that the p-chloranil was catalysing the reaction using aerial oxygen as oxidant by carrying out the reaction in air but with only catalytic amounts of chloranil, but ruled this out when the reaction failed to proceed in the presence of only catalytic amounts of the chloranil (only starting materials were recovered). The action of chloranil as oxidant is confirmed by the high yield of the reduced hydroquinone form recovered in sufficient quantities to co-crystallise with the phenoxazinone product. However aerial oxygen is also implicated as an oxidant as, although the reaction proceeds in an inert atmosphere of nitrogen, the yield plummets from 80% in air to 30% in anaerobic conditions. Involvement of aerial oxygen is in line with the detailed mechanism proposed by Begley and is therefore not surprising.4
Structure of co-crystals of [2-aminophenoxazine-3-one].1.5[chloranil]:
Solution of the single crystal structure of the co-crystals of 2-aminophenoxazine-3-one and reduced 2,3,5,6-tetrachloro-1,4-hydroquinone in space group P-1, revealed a 2
:
3 ratio for the co-crystal components, Fig. 1.
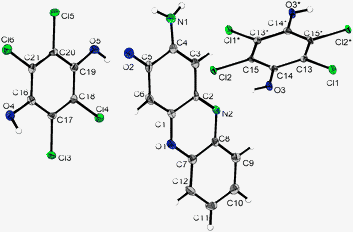 |
| Fig. 1 Crystal structure of 2-aminophenoxazine-3-one and reduced p-chloranil. Thermal ellipsoids are drawn on the 50% probability level; symmetry operations: *-x, 1-y, -z. | |
The repeat unit comprises one molecule of 2-aminophenoxazine-3-one, 2, and one and a half molecules of the reduced 2,3,5,6-tetrachloro-1,4-hydroquinone. The two halves of the 2,3,5,6-tetrachloro-1,4-hydroquinone on the special position are related by an inversion centre and the unit cell contains two repeat units, i.e. two phenoxazinone molecules and three hydroquinone molecules.
Given the enhanced potency of many metal complexes of known organic drugs over their metal free state we are interested in complexing 2-aminophenoxazine-3-one to a range of metal ions and studying the effect on the antimicrobial and anticancer activity. We now report the first structurally characterised metal complex of this important biological agent and its improved antibacterial activity towards Gram(+) bacteria over the free heterocycle.
Synthesis and characterisation of [Ag(2-aminophenoxazine-3-one)(NO3)], 3:
2-aminophenoxazine-3-one, 2, was reacted with silver(I) nitrate in 1
:
1 acetonitrile/ethanol to yield dark red coloured crystals of [Ag-2-aminophenoxazine-3-one(NO3)], 3. The 1H nmr spectrum of 3 is very similar to that of the free ligand, 2, suggesting that complexation does not alter the proton environment of the phenoxazine. The ESI-MS peak at 319 corresponds to [Ag-2-aminophenoxazine-3-one(NO3)]+ confirming the presence of 3 in solution. The shift in stretching frequency of the ring C
N from 1575 cm−1 in the free heterocycle to 1570 cm−1 in its silver complex (KBr disc) suggests that the ring nitrogen participates in coordination and this is confirmed by single crystal diffraction.
Crystal structure of [Ag(2-aminophenoxazine-3-one)(NO3)], 3:
[Ag(2-aminophenoxazine-3-one)(NO3)] crystallised in the triclinic space group P-1 and the unit cell contains two discrete repeat units of 3 which associate in a head-to-tail fashion via a pair of H-bonds through the terminal amine hydrogens and the ketonic oxygen on an adjacent complex, Fig. 2a. As indicated by the 1H nmr data, the binding is weak and the bond lengths around the ring are not significantly different from the free heterocycle, Table 1. The silver(I) is 6-coordinate with an unusual pentagonal pyramidal geometry, Fig. 2b and 2c, with a range of Ag(I)-oxygen bond lengths reflecting the coordinative plasticity of this ion.26 The silver cations and nitrate anions form double chains running along [0 0 1]. Each single chain consists of alternating cations and anions, each nitrate ion chelating two adjacent silver ions. The base of the pyramid around the [Ag]+ is completed by the phenoxazine nitrogen and the tip comes from the other single chain, combining the two single chains to a double chain, Fig. 2b. Each hydrogen-bond linked phenoxazine moiety connects two such double chains, thus forming sheets lying in the ac plane, Fig. 2c.
![(a) Hydrogen-bonded dimeric Ag(i) complex of 2-aminophenoxazine-3-one, 3; (b) View of 3 showing AgNO3 chain running along [0 0 1], phenoxazinones are represented by their coordinating nitrogen atoms only; c) View of 3 showing hydrogen-bonded phenoxazinone moieties linked to sheets by the AgNO3 chains, view side-on onto the sheets along the chains. Thermal ellipsoids are drawn on the 50% probability level in all cases.](/image/article/2010/MT/c003515g/c003515g-f2.gif) |
| Fig. 2 (a) Hydrogen-bonded dimeric Ag(I) complex of 2-aminophenoxazine-3-one, 3; (b) View of 3 showing AgNO3 chain running along [0 0 1], phenoxazinones are represented by their coordinating nitrogen atoms only; c) View of 3 showing hydrogen-bonded phenoxazinone moieties linked to sheets by the AgNO3 chains, view side-on onto the sheets along the chains. Thermal ellipsoids are drawn on the 50% probability level in all cases. | |
Bond length |
2a |
2b |
3 |
Data reported in this work at 100 K.
Data reported in ref. 25 at room temperature.
|
C(1)–C(2) |
1.471(3) |
1.467(7) |
1.468(3) |
C(2)–C(3) |
1.415(3) |
1.413 (7) |
1.413(3) |
C(3)–C(4) |
1.362(3) |
1.351(7) |
1.373(3) |
C(4)–C(5) |
1.499(3) |
1.494(6) |
1.497(3) |
C(5)–C(6) |
1.424(4) |
1.436(7) |
1.447(3) |
C(6)–C(1) |
1.399(4) |
1.343(7) |
1.347(3) |
C(7)–C(8) |
1.395(3) |
1.374(7) |
1.398(3) |
C(8)–C(9) |
1.393(3) |
1.399(7) |
1.402(3) |
C(9)–C(10) |
1.384(4) |
1.368(8) |
1.383(3) |
C(10)–C(11) |
1.396(4) |
1.377(9) |
1.405(4) |
C(11)–C(12) |
1.368(4) |
1.373(9) |
1.382(3) |
C(12)–C(7) |
1.390(4) |
1.389(7) |
1.392(3) |
C(4)–N(1) |
1.333(3) |
1.335(6) |
1.337(3) |
C(5)–O(2) |
1.239(3) |
1.238(6) |
1.230(3) |
C(2)–N(2) |
1.312(3) |
1.389 (7) |
1.325(3) |
N(2)–C(8) |
1.392(3) |
1.394(6) |
1.391(3) |
C(1)–O(1) |
1.359(3) |
1.365(7) |
1.355(3) |
O(1)–C(7) |
1.373(3) |
1.400(7) |
1.371(3) |
N(1)–H(1N1) |
0.911(18) |
0.83(4) |
0.84(4) |
N(1)–H(2N1) |
0.901(17) |
0.98(4) |
0.87(4) |
N2–Ag |
— |
— |
2.265(2) |
Ag–O(5) |
— |
— |
2.467(2) |
Ag–O(4)#1 |
— |
— |
2.496(2) |
Ag–O(3)#2 |
— |
— |
2.600(2) |
Ag–O(3) |
— |
— |
2.737(3) |
Ag–O(5)#1 |
— |
— |
2.875(2) |
N(3)–O(5) |
— |
— |
1.248(3) |
N(3)–O(3) |
— |
— |
1.249(3) |
N(3)–O(4) |
— |
— |
1.252(3) |
Biological evaluation
Comparative antibacterial activity of 2-aminophenoxazine-3-one towards Gram(+) S. aureus and Gram(−) E. coli was studied using the Kirby-Bauer disk diffusion method and the qualitative activity of 2, its silver(I) complex, 3 and a DMSO solution of silver(I) nitrate towards S. aureus and E. coli are shown in Fig. 3. 3-Aminophenoxazine-2-one has previously been shown to be inactive towards Gram(−) E. coli11,12 and we also observed the silver complex 3 to have no activity towards this microbe, Fig. 3a. In contrast the silver complex 3 shows enhanced activity against Gram(+) S. aureus compared with free 2-aminophenoxazine-3-one, Fig. 3b. We measured the activity of the DMSO solvent in this work and found it to be inactive towards both bacteria in line with previous studies.27 Despite their potency against many bacteria the mechanism for the biological activity of the aqueous silver(I) ion and silver(I) complexes is still poorly understood although their relative activities may vary widely.28
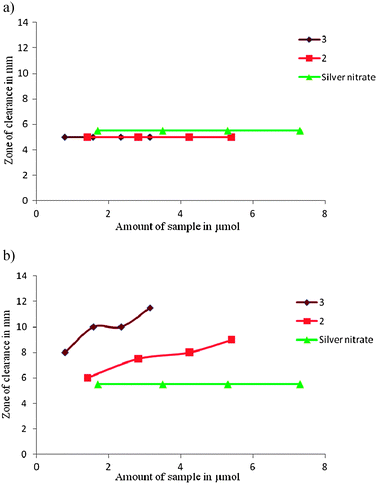 |
| Fig. 3 Activity of DMSO solutions of compounds 2, 3 and silver(I) nitrate towards a) Gram(−) E. coli and b) Gram(+) S. Aureus. Testing was conducted using individually cultured bacteria in Luria Bertoni (LB) medium and sample solutions (1.4 to 5.4 μmol for 2 and 0.78 to 3.1 μmol for 3) were placed on a 5 mm diameter paper disc and incubated at 37 °C overnight and activity was assessed by measuring the zone of clearance from the initial spot. | |
Nomiya has recently suggested that ligand exchangeability and silver coordination geometry play an important role in determining potency and has shown that complexes with labile Ag–N and Ag–O bonds are generally more active than those with stronger Ag–P and Ag–S bonds as biological donors can perhaps more effectively compete with the Ag–N/O sites thereby disrupting biochemical pathways.29 This may be the case for the S. aureus where the enhanced activity of the silver complex over the free 2-aminophenoxazine-3-one may be due to dissociation of the complex and release of silver ions in the cell. Given the demonstrated independent activity of both 2-aminophenoxazine-3-one12 and silver(I)30 towards S. aureus, it might be expected that dissociation of the complex, once transported inside the Gram(+) cell, should lead to enhanced activity compared with a solution containing only one component. This may be the reason for the enhanced activity of 3 over 2. The absence of activity of both 2 and 3 towards E. coli may be due to the greater challenges of penetrating the double layer cell wall in the Gram(−) bacterium and it may be that neither 2-aminophenoxazine-3-one or its silver(I) complex passes through in a DMSO solution.
Conclusions
In this work we have reported a new high-yielding route to 2-aminophenoxazine-3-one using p-chloranil as oxidant, and the synthesis and crystal structure of its first metal complex. This silver(I) complex shows enhanced activity towards Gram(+) S. aureus compared with the parent phenoxazinone and this may be due to the lability of the complex in cellular conditions which could provide a slow release of pathway-disrupting free silver(I) and phenoxazinone molecules. Work is currently underway to isolate and characterise additional metal complexes with this important antibacterial agent and to test their antibacterial properties.
Experimental details:
To a well stirred solution of o-aminophenol (500 mg, 4.56 mmol) in ethanol (50 ml) was added p-chloranil (1.68 g, 6.84 mmol) causing a red colour to develop. After stirring for 16 h at RT the reaction mixture was filtered. The product was purified by column chromatography using methanol/chloroform (1
:
5). 3-Aminophenoxazine-2-one was recovered as a red coloured powder (80%) and the reduced form of p-chloranil was recovered as pale white crystals. Co-crystals of the two products grew in one of the fractions and were X-rayed as described below, but clean samples of the products were isolated from the other fractions and were characterized as follows:
1H NMR: (DMSO-d6, 400 MHz): 7.69 (dd, 1H, J = 5.8 Hz), 7.48 (td, 1H, J = 8 Hz, J = 1.5 Hz), 7.43 (td, 1H, J = 8 Hz, J = 1.5 Hz), 7.36 (dd, 1H, J = 8 Hz, 1.5 Hz) 6.79 (br. s, 2H, NH2), 6.343 (s, 1H) and 6.340 (s, 1H). 13C NMR (DMSO-d6, 400 MHz): 98.80, 103.87, 116.37, 125.71, 128.40, 129.24, 134.16, 142.36, 147.82, 148.69, 149.31 and 180.65.: ESI-MS: m/z = 213 (M+). C12H8N2O4 calcd: C, 67.92, H, 3.80, N. 13.20; found C, 67.46, H, 3.80, N, 12.99. HRMS: calcd mass: 212.0586, found mass: 212.0590. M.P. = 256.5–258 °C.2,11 Selected FT-IR peaks (KBr pellet) ν 3409, 3305, 2352, 1575, 1176, 1094, 844 and 755 cm−1.
2,3,5,6-Tetrachloro-1,4-hydroquinone:
C6H2Cl4O4 calcd. C, 29.07, H, 0.81, found C, 28.82, H, 0.76. HRMS: calcd. mass for C6H2Cl4O4: 246.8701, found mass: 246.8694. Selected FT-IR peaks (KBr pellet) ν 3409, 3305, 2352, 1575, 1176 and 1094 cm−1.
Synthesis of [Ag(2-aminophenoxazine-3-one)(NO3)], 3:
To a well stirred solution of 2-aminophenoxazine (100 mg, 0.471 mmol) in 1
:
1 ethanol/acetonitrile solution (25 ml) was added AgNO3 (119 mg, 0.704 mmol) in 1
:
1 ethanol/acetonitrile (10 ml) at room temperature. The solution was filtered and dark brown crystals were recovered on standing overnight. (23%)
C12H8AgN3O5: calcd: C, 37.72; H, 2.11; N, 11.00; found C, 38.00; H, 2.11; N, 10.80. ESI-MS: m/z = 319 (M+ − NO3). Selected FT-IR peaks (KBr pellet) ν 3458, 3323, 2400, 1571, 1384, 1181, 1114 and 1035 cm−1.
Antibacterial studies
The antibacterial activity of 2-aminophenoxazine-3-one and its silver (I) complex was screened simultaneously with silver nitrate against two bacterial strains: Gram(+) Staphylococcus aureus (NCTC 7447) and Gram(+) Escherichia coli. To assess the biological activity of compounds 2-aminophenoxazine-3-one and its silver(I) complex and silver nitrate, the Kirby–Bauer disk-diffusion method was applied.31 All bacteria were individually cultured from a single colony in sterile LB medium32 overnight at 37 °C (orbital shaker incubator). All the work carried out was performed under sterile conditions.
For each strain, 70 μL of culture were spread evenly on an agar-LB medium. Four 5 mm diameter paper discs were placed evenly separated on each plate. Stock solutions (DMSO) of both compounds were prepared by dissolving 10 mg (47.2 μmol of 2, 26.2 μmol of 3 and 59.2 μmol of silver nitrate) in 100 μL to test the effect in different concentrations. Each plate was then tested with 3 μL, 6 μL, 9 μL and 12 μL of stock solution. The plates were covered and placed in an incubator at 37 °C for 24 h. The plates were then removed and the zone of clearance (defined as the diameter of inhibited bacterial growth around the filter paper) for each sample was measured in millimetres.
Crystal data for [2-aminophenoxazine-3-one and reduced form of p-Chloranil]:
C42H22N4O10Cl2(C6H2O2Cl4)3×(C12H8N2O2)2, M = 1168.04, T = 100(2) K, λ = 0.71073 Å, triclinic, space group P-1 (#2), a = 9.3384(13) Å, b = 10.8469(15) Å, c = 12.0839 (16) Å, α = 99.180°, β = 104.737 (3)°, γ = 109.772°, V = 1072.6 (3) Å3, Z = 1, Dc = 1.808 mg/m3, μ = 0.842 mm−1, F(000) = 586. Goodness of fit on F2 = 1.037. Crystal dimensions = 0.20 × 0.20 × 0.05 mm3, 9505 reflections collected, 4381 independent [R (int) = 0.0235], final R indices [I > 2σ (I)] R1 = 0.0384, wR2 = 0.0896, R indices (all data) R1 = 0.0506, wR2 = 0.0944.
Crystal data for [Ag(2-aminophenoxazine-3-one)(NO3)2]:
C12H8N3O5Ag, M = 382.08, T = 100(2) K, λ = 0.71073 Å, triclinic, space group P-1 (#2), a = 5.1653(13) Å, b = 10.3434(15) Å, c = 11.0730(16) Å, α = 99.015(2)°, β = 98.899(2)°, γ = 91.963(2)°, V = 576.26(11) Å3, Z = 2, Dc = 2.202 mg/m3, μ = 1.778 mm−1, F(000) = 376. Goodness of fit on F2 = 1.118. Crystal dimensions = 0.40 × 0.30 × 0.03 mm3, 13379 reflections collected, 3469 independent [R (int) = 0.0255], final R indices [I > 2σ (I)] R1 = 0.0317, wR2 = 0.0782, R indices (all data) R1 = 0.0341, wR2 = 0.0794.
Acknowledgements
The award of a Government of Ireland research scholarship from the Irish Research Council for Science, Engineering and Technology (IRCSET) to KP is gratefully acknowledged.
Notes and references
- E. L. Estlin and G. J. Veal, Cancer Treat. Rev., 2003, 29, 253 CrossRef CAS; U. Hollstein, Chem. Rev., 1974, 74, 625 CrossRef CAS.
- E. Reich, Cancer Res., 1963, 23(8), 1428 CAS; E. Reich, R. M. Franklin, A. J. Shatkin and E. L. Tatum, Proc. Natl. Acad. Sci. U. S. A., 1962, 48, 1238 CrossRef CAS.
- See for example J. Kleef, M. Kornmann, H. Sawhney and M. Korc, Int. J. Cancer, 2000, 86(3), 399 Search PubMed.
- C. E. Barry, P. G. Nayer and T. P. Begley, Biochemistry, 1989, 28, 6323 CrossRef.
- I. S. Fomsgaard, A. G. Mortensen and S. C. K. Carlsen, Chemosphere, 2004, 54, 1025 CrossRef CAS; M. B. Gents, S. T. Nielsen, A. G. Mortensen, C. Christophersen and I. S. Fomsgaard, Chemosphere, 2005, 61, 74 CrossRef CAS.
- X.-M. Li, H.-Q. Ju and S.-S. Zhang, Oligonucleotides, 2008, 18, 73 CrossRef CAS.
- K. Shirato, K. Imaizumi, K. Miyazawa, A. Takasaki, J. Mizuguchi, X.-F. Che, S. Akiyama and A. Tomoda, Biol. Pharm. Bull., 2008, 31, 62 CrossRef CAS.
- N. Miyano-Kurosaki, K. Kurosaki, M. Hayashi, H. Takaku, M. Hayafune, K. Shirato, T. Kasuga, T. Endo and A. Tomoda, Biol. Pharm. Bull., 2006, 29, 2197 CrossRef CAS.
- G. Fukuda, N. Yoshitake, Z. A. Khan, M. Kanazawa, Y. Notoya, X. -F. Che, S. Akiyama, A. Tomoda, S. Chakrabarti and M. Odawara, Biol. Pharm. Bull., 2005, 28, 797 CrossRef CAS.
- K. Kohno, M. Miyake, O. Sano, M. Tanaka-Kataoka, S. Yamamoto, S. Koya-Miyata, N. Arai, M. Fujii, H. Watanabe, S. Ushio, K. Iwaki and S. Fukuda, Biol. Pharm. Bull., 2008, 31, 1938 CrossRef CAS.
- S. Shimizu, M. Suzuki, A. Tomoda, S. Arai, H. Taguchi, T. Hanawa and S. Kamiya, Tohoku J. Exp. Med., 2004, 203, 47 CrossRef CAS.
- J. Bitzer, T. Groβe, L. Wang, S. Lang, W. Beil and A. Zeeck, J. Antibiot., 2006, 59(2), 86 CrossRef CAS.
- B. Ruan, Y. Tian, H. Zhou, J. Wu, Z. Liu, C. Zhu, J. Yang and H. Zhu, J. Organomet. Chem., 2009, 694(18), 2883 CrossRef CAS; K. M. Hindi, T. J. Siciliano, S. Durmus, M. J. Panzner, D. A. Medvetz, D. V. Reddy, L. A. Hogue, C. E. Hovis, J. K. Hilliard, R. J. Mallet, C. A. Tessier, C. L. Cannon and W. J. Youngs, J. Med. Chem., 2008, 51, 1577 CrossRef CAS; H. V. R. Dias, K. H. Batdorf, M. Fianchini, H. V. K. Diayabalanage, S. Carnahan, R. Mulcahy, A. Rabiee, K. Nelson and L. G. van Waasbergen, J. Inorg. Biochem., 2006, 100, 158 CrossRef.
- S. Pal, E. J. Yoon, Y. K. Tak, E. C. Choi and J. M. Song, J. Am.
Chem. Soc., 2009, 131, 16147 CrossRef CAS; A. Melaiye, Z. Sun, K. Hindi, A. Milsted, D. Ely, D. H. Reneker, C. A. Tessier and W. J. Youngs, J. Am. Chem. Soc., 2005, 127, 2285 CrossRef CAS; N. Poulter, X. Munoz-Berbel, A. L. Johnson, A. J. Dowling, N. Waterfield, A. Tobias and A. Jenkins, Chem. Commun., 2009, 7312 RSC.
- M. Giurg, K. Piekielska, M. Gębala, B. Ditkowski, M. Wolański, W. Peczyńska-Czoch and J. Młochowski, Synth. Commun., 2007, 37, 1779 CrossRef CAS.
- K. Maruyama, T. Moriguchi, T. Mashino and A. Nishinaga, Chem. Lett., 1996, 819 CAS; L. I. Simándi, T. M. Simándi, Z. May and G. Besenyei, Coord. Chem. Rev., 2003, 245, 85 CrossRef CAS.
- C. Mukherjee, T. Weyhermüller, E. Bothe, E. Rentschler and P. Chaudhuri, Inorg. Chem., 2007, 46, 9895 CrossRef CAS; M. R. Maurya, S. Sikarwar, T. Joseph and S. B. Halligudi, J. Mol. Catal. A: Chem., 2005, 236, 132 CrossRef CAS; T. Horváth, J. Kaizer and G. Speier, J. Mol. Catal. A: Chem., 2004, 215, 9 CrossRef CAS.
- J. Kaizer, G. Baráth, R. Csonka, G. Speier, L. Korecz, A. Rockenbauer and L. Parkányi, J. Inorg. Biochem., 2008, 102, 773 CrossRef CAS.
- C. Mukherjee, T. Weyhermüller, E. Bothe and P. Chaudhuri, C. R. Chimie, 2007, 10, 313 Search PubMed; M. Giurg, E. Wiech, K. Piekielska, M. Gębala, J. Młochowski, M. Wolański, B. Ditkowski and W. Peczyńska-Cozch, Polish J. Chem., 2006, 80, 297 Search PubMed.
- H. T. Nagasawa, H. R. Gutmann and M. A. Morgan, J. Biol. Chem., 1959, 234, 1600 CAS; G. W. K. Cavill, P. S. Clezy and F. B. Whitfield, Tetrahedron, 1961, 12, 139 CrossRef CAS.
- B. Gabriele, R. Mancuso, G. Salerno and M. Costa, J. Org. Chem., 2003, 68, 601 CrossRef CAS.
- J. Kaizer, R. Csonka and G. Speier, J. Mol. Catal. A: Chem., 2002, 180, 91 CrossRef CAS.
- P. H. Bernardo, C. L. L. Chai, M. Le Guen, G. D. Smith and P. Waring, Bioorg. Med. Chem. Lett., 2007, 17, 82 CrossRef CAS; S. Zhang, J. Earle, J. MacDiarmid and T. J. Bardos, J. Med. Chem., 1988, 31, 1240 CrossRef CAS; M. Yoshimoto, H. Miyazawa, H. Nakao, K. Shinkai and M. Arakawa, J. Med. Chem., 1979, 22, 491 CrossRef CAS.
- N. I. A. Sayed and K. E. Badry, Egypt. J. Chem., 2004, 47, 49 Search PubMed.
- J.-J. Nie and D.-J. Xu, (Jiegou Huaxue), Chinese Journal of Structural Chemistry, 2002, 21(2), 165 Search PubMed.
- D. Salazar-Mendoza, S. A. Baudron and M. W. Hosseini, Chem. Commun., 2007, 2252 RSC.
- B. Gleeson, J. Claffey, D. Ertler, M. Hogan, H. Müller-Bunz, F. Paradisi, D. Wallis and M. Tacke, Polyhedron, 2008, 27, 3619 CrossRef CAS.
-
N. P. Farrell, Uses of Inorganic Chemistry in Medicine, RSC, Cambridge, 1999, p. 1 Search PubMed.
- N. C. Kasuga, A. Sugie and K. Nomiya, Dalton Trans., 2004, 3732 RSC.
- Q. L. Feng, J. Wu, G. Q. Chen, F. Z. Cui, T. N. Kim and J. O. Kim, J. Biomed. Mater. Res., 2000, 52, 662 CrossRef CAS.
- A. Bondi, H. E. Spaulding, E. D. Smith and C. C. Dietz, Am. J. Med. Sci., 1947, 213, 221.
- S. E. Luria, Bacteriol Rev., 1947, 11.
|
This journal is © The Royal Society of Chemistry 2010 |