DOI:
10.1039/B917342K
(Paper)
J. Mater. Chem., 2010,
20, 117-122
Effect of concentration of photoactive chromophores on photomechanical properties of crosslinked azobenzene liquid-crystalline polymers
Received
21st August 2009
, Accepted 6th October 2009
First published on
10th November 2009
Abstract
We studied the effects of concentration and location of azobenzene chromophores on the photoinduced deformation of crosslinked liquid-crystalline polymers (CLCPs). The concentration of azobenzene chromophores in CLCP affects the degree of isomerization of azobenzene moieties and the macroscopic deformation behaviour of the films while the location of azobenzene moieties determines the contraction force and length.
Introduction
Light-driven engines have attracted much attention as a next-generation power source because they work remotely, instantly and precisely. Compared to solar cell-driven motors, the engines would be simple, compact and effective in the future because they convert light energy directly into mechanical work. Therefore, many types of photomobile materials have been proposed.1,2 Recently, we fabricated light-driven engines composed of crosslinked liquid-crystalline polymers (CLCPs) containing azobenzene moieties.3 CLCPs are liquid-crystalline polymers whose mesogens are attached to the polymer backbones with uniform alignment. CLCPs show contraction along the alignment directions of mesogens when the molecular alignments of mesogens are disordered4 by external stimuli such as heat5 and electricity.6 By incorporating azobenzene chromophores into CLCPs, deformation can be induced upon irradiation with UV light due to the reduction of the order of mesogens caused by photoisomerization of azobenzene moieties.7 Azobenzenes stabilize liquid-crystalline states in a rod-like trans form, while a bent cis form works as an impurity, resulting in an isothermal reduction of molecular order.8 Furthermore, when CLCP films are irradiated with UV light from one side, deformation is induced at an irradiated part, which brings about a bending motion.9 In addition, the bending creates various movements because the direction of the movement depends on the alignment of azobenzene mesogens and the selectivity in absorption of chromophores.10
In previous work, we prepared a CLCP composite film: a plastic film coated with a CLCP layer that was prepared from monofunctional (monomer) and difunctional (crosslinker) azobenzene monomers. The films showed large and rapid-responsive behaviour by photoirradiation, exhibiting novel three-dimensional movements such as an inchworm walk and a flexible robotic arm motion.11 The deformation of CLCPs results mainly from the reduction in order of mesogens, and all the mesogens are not necessarily photochromic. CLCPs are prepared from monomers and crosslinkers, and the photoactive moieties could be incorporated in each part. By improving the penetration of incident actinic light in CLCP films, higher performance of the photomobile materials would be expected. In fact, it has been reported that the degree of contraction is enhanced as the feed ratio of the azobenzene moiety is reduced.7c However, the effects of the location of the azobenzene moiety, at crosslinks or at side chains, on the photomechanical properties are not clear. In this work, we prepared CLCP films with various azobenzene content at various sites and explored the effects of concentration and location of photoactive chromophores on the photomobile properties.
Experimental
Materials
Chemical structures of compounds used in this study are shown in Fig. 1. CLCP films were prepared by in situ photopolymerization from mixtures of the compounds in a cell with homogeneous alignment treatment. Abbreviations and feed ratios of the samples are shown in Table 1. The former number of the abbreviation of the samples describes the feed ratio of the azobenzene monomer, A9AB9, while the latter one denotes that of the azobenzene crosslinker, DA9AB. For example, a22 was prepared from a mixture of A9AB9/DA9AB/A9BZ9/C6A = 20/20/20/40 (mol%). Monomers and crosslinkers were synthesized according to the previously reported method.3,12 The CLCP films were prepared by in situ photopolymerization of a mixture of the compounds. The mixtures containing 2 mol% of a photoinitiator (Ciba Specialty, Irgacure 784) were melted and injected into a glass cell coated with rubbed polyimide (JSR, AL1254) at 110 °C. Then it was cooled down to an LC phase temperature (87 °C for the a46 films and 75.5 °C for the other samples) and photoirradiation was carried out at >540 nm (2.5 mW cm−2 at 547 nm) with a 500 W high-pressure mercury lamp (USHIO, UI-501HQ) through glass filters (AGC techno glass, Y-52, and IRA-25S) for 2.5 h. The cooling rate of the sample was 0.1 °C min−1 for the a46, a26 and a06 films and 0.5 °C min−1 for the other samples. The CLCP films were taken off from the cells in methanol after polymerization, and dried over 1 night under reduced pressure.
Table 1 Nomenclature and feed ratio of compounds
Sample |
A9AB9 mol% |
DA9AB mol% |
A9BZ9 mol% |
C6A mol% |
a46
|
40 |
60 |
0 |
0 |
a44
|
40 |
40 |
0 |
20 |
a42
|
40 |
20 |
0 |
40 |
a40
|
40 |
0 |
0 |
60 |
a26
|
20 |
60 |
20 |
0 |
a24
|
20 |
40 |
20 |
20 |
a22
|
20 |
20 |
20 |
40 |
a20
|
20 |
0 |
20 |
60 |
a06
|
0 |
60 |
40 |
0 |
a04
|
0 |
40 |
40 |
20 |
a02
|
0 |
20 |
40 |
40 |
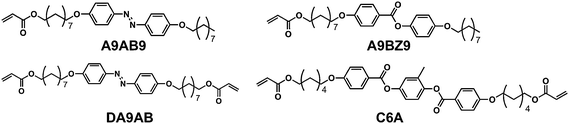 |
| Fig. 1 Chemical structures of compounds used in this study. | |
Characterization
The mesomorphic properties of the polymers were determined by differential scanning calorimetry (DSC; Seiko I&E, DSC6220G) at heating and cooling rates of 10 °C min−1. Absorption spectra of the films were recorded with a UV-vis absorption spectrometer (Jasco, V-650 with FLH-741). For evaluation of trans–cis photoisomerization of the azobenzene moieties, we used two photostationary states by two different excitation wavelengths: 366 nm from a UV-LED irradiator (Keyence, UV-400 with UV-50H and L-8) and 405 nm from a 500 W high-pressure mercury lamp through glass filters (AGC techno glass, MZ0405 and IRA-25S). Photoinduced bending of the films was brought about upon irradiation with UV light at 366 nm from the UV-LED. After measurement, the bent film was exposed to visible light from a halogen fiber lamp (Shimadzu, FLH-50) or a 530 nm LED irradiator (CCS, PJ-1505-2CA with HLV-24GR-3W) to revert to the initial shape. The change in length and stress of the CLCP films by photoirradiation was measured with a thermomechanical analyzer (Shimadzu, TMA-60).
Results and discussion
In our previous study, we used CLCP films prepared in the presence of 80 mol% crosslinker and found that the CLCP films showed a large contraction force.3 In this work, to explore the effects of the concentration and location of photoactive moieties on these properties in detail, we fixed the feed ratio of the crosslinkers (DA9AB + C6A) at 60 mol%. The results of the DSC on the CLCP films are shown in Fig. 2. It was difficult to identify clearly the glass transition temperature (Tg) in the DSC thermograms because of their high crosslinking density. However, an inflection point was observed at nearly the same temperature around 30 °C in all the thermograms. Polarized absorption spectra of the a04 film are shown in Fig. 3A. It is clear that the film showed dichroism. Order parameter (S) of the films was evaluated by the following equation, | S = (A// − A⊥)/(A// + 2A⊥) | (1) |
where A// and A⊥ are the absorbance measured with light polarized parallel and perpendicular to the rubbing direction of the alignment layers, respectively. Fig. 3B shows the values of S of the CLCP films prepared from mixtures containing various amounts of A9AB9 and DA9AB. The vertical axis indicates the order parameter, S, and the horizontal and the depth axes represent the feed ratio of crosslinker (DA9AB) and monomer (A9AB9), respectively. It was found that the order parameters of the films were almost the same among the CLCP films examined though the a06 film showed a slightly smaller value. In our previous work, we reported that the photomechanical properties of CLCP films were strongly affected by the order parameter, crosslinking density and temperature.13 It is necessary, therefore, to compare the photomechanical properties of CLCP films under the same experimental conditions with respect to these parameters. It can be assumed that all the samples prepared in this study show similar properties with respect to S, the crosslinking density and Tg.
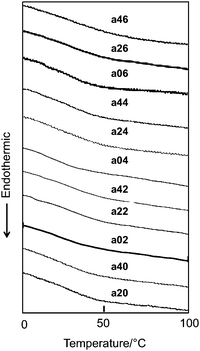 |
| Fig. 2 DSC thermograms of the CLCP films on the third heating at a rate of 10 °C min−1. | |
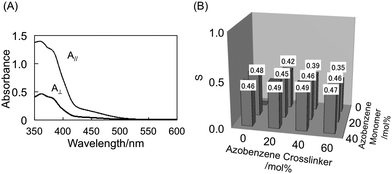 |
| Fig. 3 Polarized absorption spectra of the a04 film (A) and the order parameters of the CLCP films prepared from mixtures containing various feed ratios of the azobenzene monomer and the azobenzene crosslinker (B). A//, absorbance parallel to the rubbing direction; A⊥, absorbance perpendicular to the rubbing direction. Thickness of the film: 1 μm. | |
Next we measured the change in absorption spectra of the films. As shown in Fig. 4A the absorbance of the films around 366 nm decreased due to trans–cis photoisomerization of the azobenzene moieties upon photoirradiation at 366 nm. The spectrum of the film also changed upon exposure to 405 nm light. As photoisomerization of the azobenzene moieties proceeds, absorption of the actinic light decreases and azobenzenes in the deeper areas from the surface can photoisomerize. The degree of isomerization of the azobenzene moieties was calculated by the Fischer method14 and the ratio of the cis-form observed at the photostationary state upon irradiation at 366 nm (Cis366) in various CLCP films is shown in Fig. 4B. The horizontal and the depth axes of the figure are the same as those of Fig. 3B. It is clear that Cis366 increases with a decrease in the azobenzene content. Additionally, despite the same azobenzene content, the degree of isomerization of the a02 film was found to be lower than that of the a20 film. This is because photoisomerization of the azobenzene at the crosslinks is suppressed by its geometrical restriction. As the azobenzene crosslinkers are connected to the polymer backbones by two points, isomerization of azobenzene moieties at crosslinks is suppressed in comparison to those at the side chains.
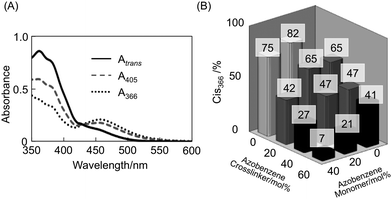 |
| Fig. 4 Change in absorption spectrum of the a04 film upon irradiation with actinic light (A) and degree of isomerization of the CLCP films containing various azobenzene monomers and azobenzene crosslinkers upon exposure to UV light (B). Thickness of the film: 1 μm. Atrans, absorption spectrum of a trans form; A405, absorption spectrum obtained at the photostationary state upon exposure to 405 nm light; A366, absorption spectrum obtained at the photostationary state upon exposure to 366 nm light. Colour of each column in (B) indicates the deformation type of the films with 10 μm thickness upon irradiation with UV light: black, bending alone; gray, partly unbending after bending by 90°; white, completely unbending (see Fig. 5). | |
Photoinduced motion of the CLCP films with 10 μm thicknesses was examined under an experimental setup shown in Fig. 5A. To irradiate the CLCP films under the same experimental condition, we covered part of the CLCP film with a glass substrate and irradiated them from above. By irradiation with UV light at 240 mW cm−2, CLCP films with a high azobenzene content (a46, a26, a06 and a44) bent toward the UV light source (Fig. 5(B-a)). On the other hand, CLCP films with low azobenzene content exhibited unbending behaviour after they bent to 90° by continuous irradiation with UV light (Fig. 5(B-b)). Especially, the a20 and the a40 films reverted completely to the initial flat shape (Fig. 5(B-c)). The irradiated film showed no further bending upon continuous UV light irradiation. However, when we used CLCP films with a 20 μm thickness or UV light with low intensity (25 mW cm−2), the films did not exhibit such a “reverting motion”, showing the same motion as indicated in Fig. 5(B-a). A plausible mechanism for the unbending behaviour is the relaxation of the gradient of the deformation along the depth direction as described in Fig. 5C. Since the incident light penetrates deeper areas from the surface of the film, photoinduced contraction occurs at the opposite side of the film as well as at the irradiated surface. Consequently, the bent films revert to the initial shape. We colour-coded Fig. 4B according to the photoinduced motion: black, bending; gray, incompletely unbending; white, unbending. It is clear that the degree of isomerization plays an important role on the macroscopic deformation of the films. In addition, the bent films reverted to the initial shape when they were left in the dark over one night or irradiated with visible light at room temperature. This observation supports the result of DSC that the films showed Tg’s around room temperature.
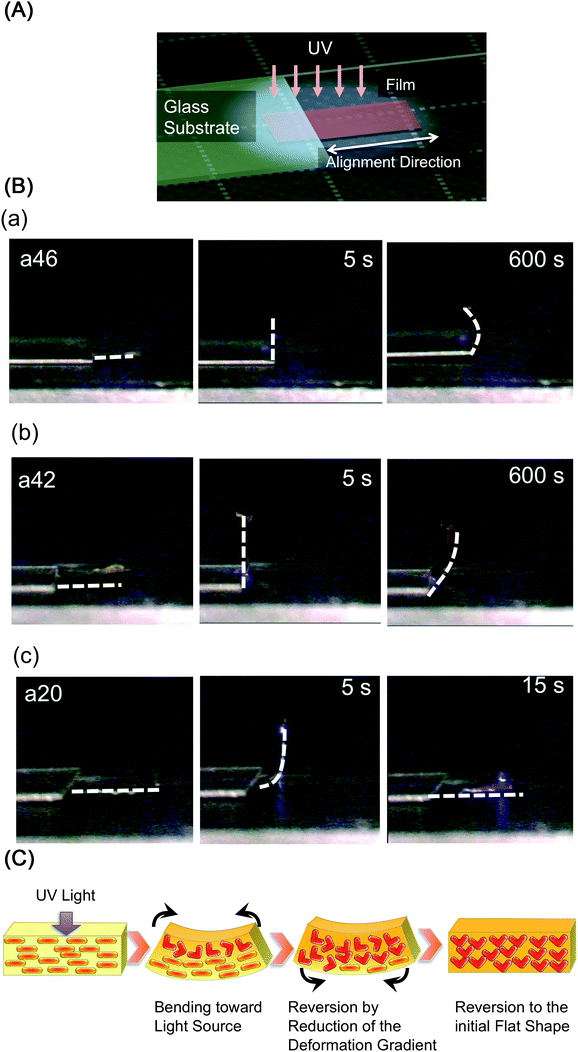 |
| Fig. 5 Schematic illustration of the experimental setup (A), photographs of CLCP films exhibiting photoinduced deformation (B) and schematic illustrations showing a plausible mechanism of the photoinduced deformation of CLCP films (C). The series of photographs in (B) show the motion of CLCP films by irradiation with UV light (366 nm, 240 mW cm−2) at room temperature: the first frame, before irradiation; the second frame, after irradiation for 5 s; the third frame, after continuous irradiation with UV light. The films showed different photoinduced deformation: (a) bending alone; (b) partly unbending after bending by 90°; (c) completely unbending. Size of the film: 2 mm × 3 mm × 10 μm. The white dash line in the photographs describes the edges of the film. | |
Photomechanical properties of the CLCP films were evaluated with an experimental setup shown in Fig. 6A. Both ends of a film were clamped and 10 mN was added as an initial load. The direction of the initial load was parallel to the alignment direction. Herein, a change in length of the CLCP films upon exposure to UV light, Δl, is defined by the following equation,
where l
0 and l denote the length of the film before and after photoirradiation, respectively. Upon exposure to UV light, the CLCP films contracted along the alignment direction (
Fig. 6B). We evaluated this photomechanical property by the change in length upon irradiation with UV light at 25 mW cm
−2 for 100 s (Δl
100). The values of Δl
100 of the various CLCP films are shown in
Fig. 6C. As the
azobenzene content decreased, the film showed a large contraction. Especially, Δl
100 of the
a02 film is about 4 times larger than that of the
a46 film, which contains
azobenzene mesogens alone. On the other hand, both
a20 and
a40 that were prepared without the
azobenzene crosslinker exhibited a small contraction though they showed a high degree of
photoisomerization. These results explain why the
a20 and
a02 films exhibited different photoinduced motions. The photoinduced change in length of the
a20 film is so small that the gradient deformation along the depth direction is also small enough for the film to revert to the initial flat-state, even the gradient remains in the depth direction. On the other hand, a change in length of the
a02 film is large, resulting in the generation of a large gradient and the film remains in the bent shape.
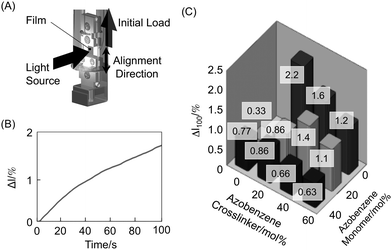 |
| Fig. 6 Schematic illustration of an experimental setup to evaluate photomechanical properties (A), change in length along the alignment direction of the a04 film by irradiation with UV light (366 nm, 25 mW cm−2) at 30 °C (B) and the value of contraction length after irradiation with UV light for 100 s (Δl100) of the CLCP films containing various azobenzene contents (C). Size of the film: 5 mm × 7 mm × 20 μm. | |
Finally, we evaluated the mechanical force of the films that was generated in the films upon irradiation with UV light. As the length of the film was kept unchanged, the increase in the load indicates the generation of the mechanical force. The mechanical force generated by irradiation with UV light, ΔF, is defined as follows,
where F
0 and F denote the force before and after irradiation with UV light. Upon irradiation with UV light, the load of the films increased as shown in
Fig. 7A. The generated force was evaluated by the value of the force after irradiation for 100 s (ΔF
100). The values of ΔF
100 of the samples are plotted in
Fig. 7B. The ΔF
100 showed the same tendency as Δl
100, but the difference is more significant: ΔF
100 of the
a02 and
a04 film is over 2.5 MPa, 7 times larger than that of
a46 film, which is larger than the previously reported films.
3 On the other hand, ΔF
100 of the
a40 and
a20 film was found to be low, indicating that the effect of
photoisomerization of
azobenzene chromophores at side chains is insignificant on photomechanical properties.
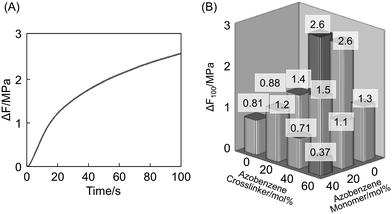 |
| Fig. 7 Mechanical force generated in the a04 film by UV light irradiation (366 nm, 25 mW cm−2) at 30 °C (A) and value of mechanical force generated by irradiation with UV light for 100 s (ΔF100) of the CLCP films containing various azobenzene content (B). Size of the film: 5 mm × 7 mm × 20 μm. | |
Conclusion
In summary, homogeneously aligned CLCP films with various azobenzene contents at various sites were prepared. The effects of concentration and location of azobenzene chromophores on photomobile and photomechanical properties were evaluated under the same conditions with respect to Tg, S and the crosslinking density. Upon exposure to UV light, the films exhibited photoinduced bending and unbending behaviour at room temperature. As the feed ratio of azobenzene moieties in the CLCP films decreases, the degree of isomerization and the penetration of incident light are enhanced, which leads to an effective photoinduced movement. It has been found that the photoisomerization of azobenzene chromophores at crosslinks affects more significantly than that at the side chains. The a02 film showed the highest photomechanical properties that were about 4 times larger in contraction length and 7 times larger in mechanical force than the CLCP film prepared from azobenzene moieties alone. It can be assumed that a way of further improvement of photomechanical properties is increment of azobenzene concentration without reduction of the degree of photoisomerization on crosslinking part. One solution to satisfy these problems is a block copolymer that separates photoactive part and crosslinking part.15
References
- S. Masiero, S. Lena, S. Pieraccini and G. P. Spade, Angew. Chem., Int. Ed., 2008, 47, 3184 CrossRef CAS.
-
(a) T. Ikeda, J. Mamiya and Y. Yu, Angew. Chem., Int. Ed., 2007, 46, 506 CrossRef CAS;
(b) C. J. Barrett, J. Mamiya, K. G. Yager and T. Ikeda, Soft Matter, 2007, 3, 1249 RSC.
- M. Yamada, M. Kondo, J. Mamiya, Y. Yu, M. Kinoshita, C. J. Barrett and T. Ikeda, Angew. Chem., Int. Ed., 2008, 47, 4986 CrossRef CAS.
- P. G. de Gennes, C.R. Acad. Sci. B, 1975, 281, 101.
-
(a) J. Küpfer and H. Finkelmann, Macromol. Chem. Phys., 1994, 195, 1353 CrossRef;
(b) H. Wermter and H. Finkelmann, e-polymers, 2001,(no. 13) Search PubMed;
(c) D. L. Thomsen III, P. Keller, J. Naciri, R. Pink, H. Jeon, D. Shenoy and B. R. Ratna, Macromolecules, 2001, 34, 5868 CrossRef.
-
(a) W. Lehmann, H. Skupin, C. Tolksdorf, E. Gebhard, R. Zentel, P. Krüger, M. Lösche and F. Kremer, Nature, 2001, 410, 447 CrossRef CAS;
(b) C. M. Spillmann, B. R. Ratna and J. Naciri, Appl. Phys. Lett., 2007, 90, 021911 CrossRef.
-
(a) H. Finkelmann, E. Nishikawa, G. G. Pereira and M. Warner, Phys. Rev. Lett., 2001, 87, 015501 CrossRef CAS;
(b) P. M. Hogan, A. R. Tajbakhsh and E. M. Terentjev, Phys. Rev. E: Stat., Nonlinear, Soft Matter Phys., 2002, 65, 041720 CrossRef CAS;
(c) M. H. Li, P. Keller, B. Li, X. Wang and M. Brunet, Adv. Mater., 2003, 15, 569 CrossRef CAS.
-
(a) S. Tazuke, S. Kurihara and T. Ikeda, Chem. Lett., 1987, 911 CAS;
(b) T. Ikeda and O. Tsutsumi, Science, 1995, 268, 1873 CrossRef CAS.
- T. Ikeda, M. Nakano, Y. Yu, O. Tsutsumi and A. Kanazawa, Adv. Mater., 2003, 15, 201 CrossRef CAS.
-
(a) Y. Yu, M. Nakano and T. Ikeda, Nature, 2003, 425, 145 CrossRef CAS;
(b) N. Tabiryan, S. Serak and X. M. Dai, Opt. Express, 2005, 13, 7442 CrossRef CAS;
(c) M. Kondo, Y. Yu and T. Ikeda, Angew. Chem., Int. Ed., 2006, 45, 1378 CrossRef CAS;
(d) K. D. Harris, R. Cuypers, P. Scheibe, C. L. van Oosten, C. W. M. Bastiaansen, J. Lub and D. J. Broer, J. Mater. Chem., 2005, 15, 5043 RSC;
(e) T. J. White, N. V. Tabiryan, S. V. Serak, U. A. Hrozhyk, V. P. Tondiglia, H. Koerner, R. A. Vaia and T. J. Bunning, Soft Matter, 2008, 4, 1796 RSC.
- M. Yamada, M. Kondo, R. Miyasato, Y. Naka, J. Mamiya, M. Kinoshita, A. Shishido, Y. Yu, C. J. Barrett and T. Ikeda, J. Mater. Chem., 2009, 19, 60 RSC.
-
(a) L. Andruzzi, F. D. Apollo, G. Galli and B. Gallot, Macromolecules, 2001, 34, 7707 CrossRef CAS;
(b) B. Donnio, H. Wermter and H. Finkelmann, Macromolecules, 2000, 33, 7724 CrossRef CAS.
-
(a) Y. Yu, M. Nakano and T. Ikeda, Pure Appl. Chem., 2004, 76, 1467 CrossRef CAS;
(b) Y. Yu, M. Nakano, A. Shishido, T. Shiono and T. Ikeda, Chem. Mater., 2004, 16, 1637 CrossRef CAS;
(c) Y. Yu, T. Maeda, J. Mamiya and T. Ikeda, Angew. Chem., Int. Ed., 2007, 46, 881 CrossRef CAS.
- E. Fischer, J. Phys. Chem., 1967, 71, 3704 CrossRef.
- M. H. Li, P. Keller, J. Yang and P. A. Albouy, Adv. Mater., 2004, 16, 1922 CrossRef CAS.
|
This journal is © The Royal Society of Chemistry 2010 |
Click here to see how this site uses Cookies. View our privacy policy here.