DOI:
10.1039/B915490F
(Paper)
J. Mater. Chem., 2010,
20, 145-151
Stimuli-responsive europium-containing metallo-supramolecular polymers†
Received
29th July 2009
, Accepted 28th August 2009
First published on
24th September 2009
Abstract
A series of stimuli-responsive films based on metallo-supramolecular polymers have been prepared and studied. The metallo-supramolecular polymers are comprised of a 4-oxy-2,6-bis-(1′-methylbenzimidazolyl)pyridine ditopic endcapped poly(tetrahydrofuran) with different ratios of Zn2+ and Eu3+. A combination of the optical properties of the Eu3+ complex along with its more labile nature results in the formation of highly stimuli-responsive materials. The films of the Eu3+-containing metallo-supramolecular polymers show a pronounced optical response to an increase in temperature or upon exposure to chemicals such as triethyl phosphate, which was used as a mimic for organophosphate pesticides and nerve gas agents.
Introduction
The ability to utilize supramolecular interactions1 toward the design of new stimuli-responsive materials has gained a great amount of interest in recent years.2 For example, main-chain supramolecular polymers, whose polymer backbone consists of both covalent bonds and non-covalent interactions, can result in large (e.g. mechanical) responses to small changes in the environment.3 Reversible metal–ligand coordination is one class of non-covalent interaction that has been widely utilized to access main-chain supramolecular polymers.4 Most of these metallo-supramolecular polymers rely on ligand coordination with transition metal ions. Such systems have been targeted, at least in part, on account of the strength of the metal–ligand interaction and the fact that 2 : 1 ligand to metal complexes (required for linear chain extension) can be easily accessed with either terdentate ligands bound to hexacoordinate metal ions or bidentate ligands bound to tetracoordinate metal ions.5 A series of metal ions that have seen much less attention in this field are the lanthanide metals,6 which can be of special interest as their complexes have been shown to have many potential applications that include (bio-)sensing,7 optical fiber lasers and amplifiers,8 liquid crystals9 and light-emitting diodes.10 One of the most interesting and unique properties of certain lanthanide ion complexes is their excellent luminescence characteristics that include extremely sharp emission bands and high photoluminescence (PL) efficiency.11
Combining the distinctive properties of lanthanide complexes with the mechanical properties of polymers presents an interesting method of creating stimuli-responsive metal-containing materials. We have shown that 2,6-bis-(1′-methylbenzimidazolyl)pyridine (Mebip) ligands can be placed at the ends of ditopic macromonomers, which consist of either conjugated12 or polyether cores,13 and produce polymeric materials upon addition of an appropriate transition metal ion (e.g. Fe2+, Co2+, or Zn2+) in a 1 : 1 ratio of metal to ditopic macromonomer. Such transition metals bind the terdentate Mebip in a 2 : 1 ligand to metal ratio that allows for the formation of linear high molecular weight metallo-supramolecular polymers resulting in a significant enhancement in the solid-state mechanical properties of these materials compared to the unbound macromonomer.
Pioneering work by Piguet and Bünzli has shown that planar aromatic terdentate ligands, like Mebip, will coordinate with lanthanide ions to form metallo-supramolecular complexes.14 Some lanthanide ion complexes, e.g. Eu3+ complexes, can show an intense metal-centered luminescence in the presence of an appropriate UV absorbing ligand via the so-called “antenna effect”.15 This is in effect a light conversion process which occurs by absorption of the light by the ligand, followed by a ligand to metal energy transfer process finally resulting in the metal ion-based emission. The Mebip ligand has been shown16 to act as an effective “antenna” for Eu3+ ions and as such this “antenna effect” can be used to demonstrate whether the ligand is complexed to the Eu3+ ion. More recently, Piguet and co-workers have taken an in-depth look at the interaction between lanthanides and terdentate aza-ligands in both the solid-state and in solution.17 With their preference for high coordination numbers (8–10),18 lanthanides, such as Eu3+, can coordinate with Mebip in a 3 : 1 ligand to metal ratio. Piguet and co-workers demonstrated that the nature of the complexes formed between the lanthanides and the terdentate aza-ligands is very sensitive to the counterion present. For example, europium nitrates (Eu(NO3)3) were found to bind such ligands predominately in a 1 : 1 ligand to metal ratio because of competitive binding between the nitrate and the ligand. Conversely, europium perchlorate (Eu(ClO4)3) will bind in a 3 : 1 Mebip ligand to metal ratio as the Mebip ligands now have much stronger interactions with the metal ion than the weakly binding perchlorate counterions. This suggests that incorporating lanthanide perchlorates into a supramolecular polymer should result in the creation of dynamic branching or networking points19 that could lead to changes in mechanical properties.
Previously,20 we have prepared stimuli-responsive gels in which Mebip ligands were placed on the ends of a pentaethylene glycol core.21 The addition of Zn2+ ions with or without varying amounts of lanthanide ions produced gels in acetonitrile that displayed interesting stimuli-responsive behaviour. The lanthanide containing gels were found to show enhanced thermo-, mechanical- and chemo-responsiveness which in part was a consequence of the Mebip binding more weakly to the lanthanide ion as compared to the Zn2+ ions.
Along the same lines, complexes of low molecular weight Mebip derivatives have demonstrated the capability of sensing small amounts of certain organophosphates22 that were used as pesticides and nerve gas agent mimics.23 These ligands were complexed with La(NO3)3 and Eu(NO3)3 to form highly emissive 1 : 1 complexes. When exposed to the organophosphates, either in solution or vapour, these materials showed a change in colour from red to blue. In this case the organophosphate binds to the Eu3+ ion, displacing the Mebip ligand and thus converts the red emissive Eu3+–Mebip complex to the free Mebip ligand (blue emission) and a non-emissive Eu3+–organophosphate complex. It is interesting to note that stronger binding Zn2+ complexes of similar Mebip ligands did not show Mebip displacement in the presence of organophosphates.
We have already shown that ditopic macromonomer 1 (Fig. 1) derived from low molecular weight poly(tetrahydrofuran) (p(THF)) cores (Mn < 5000 g·mol−1) form thermoplastic elastomers once complexed with Zn2+ ions.13 It is believed that the elastomeric behaviour occurs as a consequence of phase segregation between the hard ionic metal–ligand complexes and the soft poly(tetrahydrofuran) segments. Thus with the goal of developing stimuli-responsive thermoplastic elastomers we report herein the effects of replacing a percentage of the Zn2+ ion complexes with the more responsive Eu3+ complexes in these metallo-supramolecular polymers. From a structural point-of-view this is also interesting as conceptually 3 : 1 complexes will result in the formation of dynamic-crosslinking points. Thus, as shown schematically in Fig. 1, the addition of both Eu3+ and Zn2+ ions to 1 should result in a crosslinked metallo-supramolecular polymer in solution and in the solid-state. Of course, the presence of physical crosslinking in the solid phase on account of the phase segregation between metal–ligand complexes and the polymer core adds an additional level of complexity to this system.
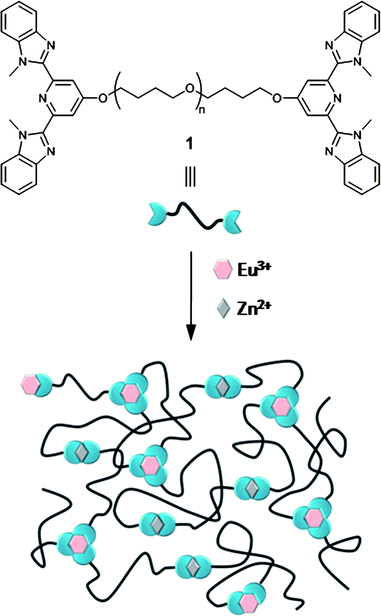 |
| Fig. 1 Ditopic ligand endcapped macromonomer 1 and schematic representation of the formation of a metallo-supramolecular polymer. | |
Experimental section
Materials
All reagents and solvents were purchased from Aldrich Chemical Co. Reagents were used without further purification. Solvents were distilled from suitable drying agents. Spectrophotometric grade chloroform and acetonitrile were used for all experiments.
Instruments
NMR spectra were recorded on a Varian 600 NMR spectrometer. Dynamic mechanical thermal analysis experiments were performed using a TA Instruments DMAQ800 under N2 with liquid N2 cooling and heated at a rate of 10 °C min−1. UV-vis spectra were obtained by a Perkin-Elmer Lambda 800 UV-vis spectrometer. Titration experiments were performed in quartz cuvettes with scanning in the range of 250–400 nm with an integration time of 0.24 s. Fluorescence spectra were obtained with a SPEX Fluorolog 3 (model FL3-12); corrections for the spectral dispersion of the Xe-lamp, the instrument throughput, and the detector response were applied. Temperature and chemical dependent photoluminescence spectra were acquired on an Ocean Optics ACD1000-USB spectrometer (λex = 377 nm) through the use of a Y-shaped optical fiber in conjunction with a Gel Instrumente AG hotstage with a TC2 temperature controller. The broad peak centering around 490 nm that is observed in some measurements is the product of a reflection from the slide the films were placed on. Molecular weights of the materials were measured by mass spectrometry on a Bruker AUTOFLEX III MALDI-TOF/TOF mass spectrometer using HABA [2-(4-hydroxypheylazo)benzoic acid] as the matrix with a sodium trifluoroacetate additive. X-Ray measurements were conducted using a Rigaku SA-HF3 X-ray generator for the D/MAX2000/PC series diffractometer. All samples for X-ray study were prepared as films and placed on a glass cover slide aligned in the path of the wide-angle diffractometer.
Preparation of 1
In flame dried glassware, a mixture of 4-hydroxy-2,6-bis(1′-methylbenzimidazoyl)pyridine (HOMebip, 5.03 g, 14.1 mmol) and N,N-diiospropylethylamine (DIEA, 5.00 mL, 28.7 mmol) was suspended in 350 mL of freshly distilled tetrahydrofuran (THF). In a separate flask, 350 mL of freshly distilled THF were cooled with stirring to 5 °C in an ice bath. Trifluoromethanesulfonic anhydride (1 mL, 5.9 mmol) was added to the THF and allowed to polymerize for 20 min. After 20 min, the suspension of HOMebip and DIEA was added to terminate the growing polymer chain and stirred overnight. The solvent was removed under vacuum to give a viscous purple oil which was then dissolved in CHCl3 and washed with 1 M NaOH (× 3). The precipitated unreacted HOMebip was filtered and the organic phase was stirred with decolourizing carbon to further remove excess HOMebip. After filtering off the carbon, the solvent was again removed under vacuum and the product was purified by column chromatography (CHCl3 : MeOH, 100 : 0, 99 : 1, 98 : 2) to give macromonomer 1 (7.9 g, 67%). δH(600 MHz; CDCl3) 7.92 (s, 4H, Ar), 7.86 (d, 4H, Ar), 7.46 (d, 4H, Ar), 7.36 (m, 8H, Ar), 4.26 (t, 4H, MebipOCH2), 4.23 (s, 12H, CH3), 3.40 (m, 256H, OCH2CH2), 1.61 (m, 260H, OCH2CH2); δC(100 MHz; CDCl3) 166.4, 150.9, 150.2, 142.3, 137.0, 123.3, 122.6, 119.9, 111.6, 109.8, 70.4, 32.4, 26.3. m/z (MALDI TOF-TOF) (matrix: HABA): Mn = 3500. PDI: 1.03.
Example procedure for 1 with Zn2+ : Eu3+ 90 : 10. A solution containing 180 mg (0.045 mmol) of 1 in 1 mL of chloroform was mixed with 0.3 mL (0.003 mmol) of Eu(ClO4)3 from a 10 mM stock solution in acetonitrile then with 2.025 mL (0.0405 mmol) of Zn(ClO4)2 from a 20 mM stock solution in acetonitrile. Once everything had dissolved, the solvent was removed under vacuum and the resulting polymer was redissolved in 1.5 mL of chloroform. This solution was then cast into an aluminium-walled casting dish with a Teflon sheet bottom. The complex was allowed to air-dry overnight and then was vacuum dried in an oven for 6 h at 40 °C. Films of other metal ratios were prepared by altering the amount of the Eu3+ and Zn2+ stock solutions appropriately. All films studied had a thickness of 200 ± 20 µm.
Temperature and chemical responsiveness experiments
A Zn2+ : Eu3+ 70 : 30 film was used for all experiments. The response to temperature was examined by placing the film on a glass slide at room temperature and heating to 120 °C before cooling to room temperature by simply removing the film from the heat source; this cycle of heating and cooling was then repeated. The PL spectra were recorded at different temperatures using an optical fiber setup (Ocean Optics).
Experiments for the detection of triethyl phosphate were carried out using a Zn2+ : Eu3+ 70 : 30 film. The solution detection was carried out by dipping the film into a 10 mM triethyl phosphate solution in hexanes. Colour changes were observed after short periods of time (10 s) with the full quenching of the red Eu3+-based emission occurring after 1 min. This was also seen in 1 mM solutions, although the time required for detection was greater. Vapour detection was accomplished by creating an analyte-rich environment by heating a beaker of triethyl phosphate at 60 °C for 2 h in a closed container. The temperature was then dropped back to room temperature (to avoid any thermal response) and the film was placed into the container for the desired amount of time.
Results and discussion
Macromonomer 1 was synthesized following a procedure similar to one we previously reported.13 The cationic, living ring-opening polymerization of tetrahydrofuran was carried out using trifluoromethanesulfonic anhydride as the initiator and 4-hydroxy-2,6-bis(1′-methylbenzimidazoyl)pyridine as the end-capping chain terminator. This versatile method not only allows for control of the polymer molecular weight by simply varying the reaction time but can also be carried out on relatively large scales (routinely run on a 10 g scale).
Optical properties of the metallosupramolecular polymer films
The coordination of Eu(ClO4)3 to 1 was initially studied using UV-vis spectroscopy (Fig. 2). Complexation of Mebip to Eu3+ results in an increase in the ligand absorbance around 340 nm and a decrease in the absorbance at 315 nm, which corresponds to the λmax of the free ligand. Plotting the absorbance at 340 nm vs. the ratio of metal to ligand (Fig. 2 inset) shows that Eu(ClO4)3 can bind three of the polymeric Mebip ligands, confirming that the presence of the polymer does not significantly alter the binding ratio of Mebip to Eu3+. Films of the metallo-supramolecular polymers with the metal perchlorate salts were obtained by solution casting. Six films were studied containing different ratios of Zn2+ to Eu3+ ranging from only Zn2+ and no Eu3+ (100 : 0) to 50% Zn2+ and 50% Eu3+ (50 : 50). The percentages refer to the percent of ligands in the system which are bound to that metal ion, assuming a 2 : 1 ratio for Zn2+ and 3 : 1 ratio for Eu3+ (although we cannot rule out the presence of a certain percentage of 2 : 1 and even 1 : 1 ligand : Eu3+ complexes). All of these ratios produced optically clear, mechanically stable, elastomeric films (Fig. 3). Attempts to cast films containing greater than 50% of the ligands complexed to Eu3+ result in mechanically unstable films, suggesting that there is a limit to the amount of the weaker binding Eu3+ that can be used in order to get films with good mechanical properties.
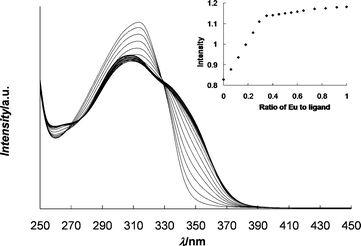 |
| Fig. 2 UV titration of Eu(ClO4)3 with 1 (25 μM); inset: plot of absorbance at λ = 340 nm vs. molar ratio of Eu3+ to ligand. | |
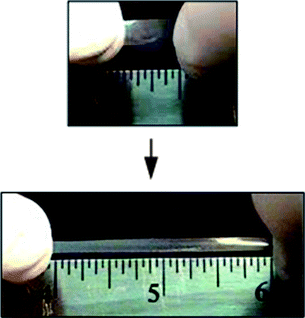 |
| Fig. 3 A Zn2+ : Eu3+ 80 : 20 film stretched by hand which shows the elastomeric nature of the films caused by the physical crosslinks formed from phase segregation of the metal–ligand complex from the polymeric core. | |
All six of the films studied were optically clear under visible light, but were fluorescent upon exposure to UV light. Fig. 4 shows the fluorescence of the six films under irradiation with 365 nm light. The film containing no Eu3+ (100 : 0) gives a blue fluorescence (λmax = 410 nm) on account of the Mebip : Zn2+ complex. As Eu3+ is introduced into the supramolecular polymer, more of the Eu3+ : Mebip complex's emission is observed and as a result the film's emission changes from blue to red/pink. For example, the film containing Zn2+ : Eu3+ 80 : 20 appears light pink/purple from the combination of the blue emission and the red emission. These effects are shown in the photoluminescence spectra (excited at 365 nm) of the films in Fig. 5 (solution data are given in the ESI†). The addition of Eu3+ results in a decrease in the emission band at 410 nm and the appearance of the distinctive Eu3+ emission bands at 575 nm, 590 nm, 615 nm and 645 nm. These bands have been identified as the 5D0 → 7F0–3 transitions.24 Upon increasing the concentration of Eu3+ within the films there is a decrease in the emission band at 410 nm and the distinctive Eu3+-based emission bands at 590 nm and 615 nm appear.
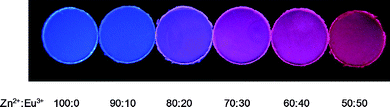 |
| Fig. 4 Pictures of solution cast films of 1 with varying amounts of Zn2+ and Eu3+ under UV light (365 nm) demonstrating the effect of metal content on the fluorescence colour. | |
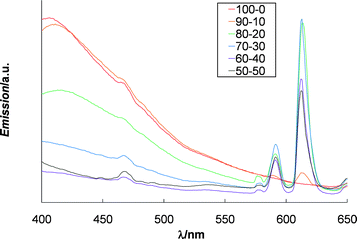 |
| Fig. 5 Photoluminescence spectra of films of 1 and varying ratios of Zn2+ and Eu3+ ranging from Zn2+ : Eu3+ 100 : 0 to 50 : 50 (excited at 365 nm). | |
Thermomechanical properties
The thermomechanical properties of the films were then investigated with the goal of elucidating the effect of varying the Zn2+ : Eu3+ ratio of the these metallo-supramolecular polymers. Thus dynamic mechanical thermal analysis (DMTA) studies were carried out on the series of films that range from Zn2+ : Eu3+ 100 : 0 to Zn2+ : Eu3+ 50 : 50. Fig. 6 shows the DMTA data for the six films that were annealed at −35 °C for 60 min prior to being cooled to −110 °C, at which point the run was started. If this annealing step is not carried out and the samples are cooled to −110 °C directly from room temperature then an increase in modulus is seen around −35 °C (see ESI†) during the experiment, which is attributed to the cold crystallization of the p(THF) core. Annealing at −35 °C for 60 min before the experiment removes most of this effect. Two other major thermal transitions are observed in all six of the films. The first transition corresponds to the glass transition temperature of poly(tetrahydrofuran) which is seen at ca. −80 °C, while the second transition is attributed to the Tm of the low molecular weight p(THF) segment that occurs around 0 °C. The presence of both the peaks is consistent with the p(THF) being phase segregated from the metal complexes. The major difference in the thermomechanical properties of these films comes at higher temperatures. As a general trend the lower the Zn2+ : Eu3+ ratio the weaker and more thermally sensitive the films become. For example, after the Tm transition the modulus of the 100% Zn2+ film stays relatively constant at ca. 30 MPa (the relative independence of modulus with temperature in this range is consistent with the presence of physical crosslinking) with increasing temperature, only dropping off significantly above 120 °C. The Zn2+ : Eu3+ 50 : 50 film on the other hand shows a gradual decrease in modulus, starting at a modulus of ca. 30 MPa after the melting point of p(THF), with increasing temperature and showing a significant drop in modulus above 40 °C.
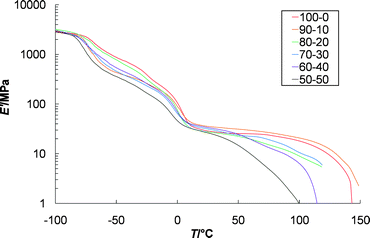 |
| Fig. 6 Dynamic mechanical thermal analysis of solution cast films made from 1 with different ratios of Zn2+ to Eu3+ ranging from Zn2+ : Eu3+ 100 : 0 to 50 : 50. All samples were annealed at −35 °C prior to being run. All samples display a glass transition of ca. −80 °C and a melting temperature of ca. 0 °C which are attributed to the p(THF) core. | |
The trend toward more thermally responsive films upon increasing Eu3+ content can be rationalized in a number of different ways. One aspect is the relative binding strength of Eu3+vs. Zn2+ to the ligand. It has already been shown that Eu3+ is a weaker binder to Mebip than Zn2+ ions.20 Thus, it is expected that these films will be more thermally responsive.
To see if the presence of Eu3+ has any effect on the mechanical properties of these films, a series of four films that ranged from 80 : 5 to 80 : 20 (Zn2+ : Eu3+) were prepared and investigated. Interestingly, all four supramolecular polymers formed mechanically stable stand alone films, even though for three of them a significant number of chain ends, in the form of free ligand, must be present. The presence of physical crosslinks caused by phase segregation explains why these materials are not as sensitive to metal–ligand stoichiometry as may be expected.25 DMTA of these films (Fig. 7) do show, however, that the presence of the Eu3+ ions does enhance their thermomechanical properties. There is a small improvement in the films' moduli as free ligands are bound to Eu3+, and an improvement in the temperature at which the sample yields (the slight increase in E′ right before the sample yields is presumably from slippage at the clamps).
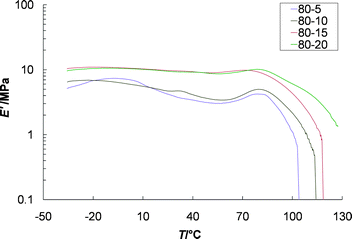 |
| Fig. 7 Dynamic mechanical thermal analysis of solution cast films with varying ratios of Zn2+ : Eu3+ ranging from Zn2+ : Eu3+ 80 : 5 to 80 : 20, showing the enhancement of mechanical properties with addition of Eu3+ ions. | |
It can be expected that in the solid-state a significant percentage of the Eu3+ ions are complexed to three Mebip ligands and thus can potentially act as dynamic-crosslinking sites. However, as mentioned before, the mechanical properties of these films seem to be related to the phase separation between the core and the metal complexes. Thus it is also possible that the incorporation of the (Eu : Mebip3)3+ complexes results in a disruption of the ordered (Zn : Mebip2)2+ crosslinking hard phase. To examine this aspect in more detail WAXS studies were carried out on selected films which contain the different ratios of Zn2+ to Eu3+. As we have seen before13 the Zn2+ only films show two main peaks, one at d = 9.8 Å (2θ = 9.0) and another at d = 60.2 Å (2θ = 1.5). We have previously assigned these peaks to correspond to the metal-to-metal distance within the hard phase (d = 9.8 Å) and the metal-to-metal distance between the hard phases (d = 60.2 Å).13 As the Eu3+ content is increased in these films the peak (at d = 9.8 Å) corresponding to the order within the hard phase starts to decrease (Fig. 8) suggesting some sort of disruption of this phase . However, the peak at d = 60.2 Å is still observed even in the Zn2+ : Eu3+ 50 : 50 material. Thus these results are consistent with the presence of some phase segregation in the Eu3+-containing films but with disorder occurring within the hard phase which could contribute to the weakening of the films. We are currently studying the rheology of the films in more detail to better understand the mechanisms at play here.
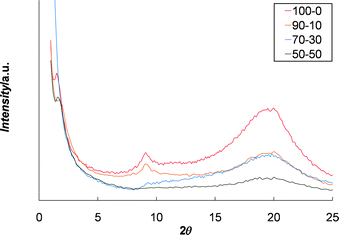 |
| Fig. 8 WAXS of selected films of 1 with varying ratios of Zn2+ : Eu3+ which demonstrate the loss of order in the hard phase (d = 9.8 Å, 2θ = 9) as the ratio of Zn2+ : Eu3+ decreases. | |
Stimuli-responsive behaviour
Having demonstrated the increased thermomechanical sensitivity of the Eu3+-containing polymers, an investigation of the optical response of these films was undertaken upon exposure to either temperature or chemical stimuli. For example, upon heating the 70 : 30 (Zn2+ : Eu3+) film to about 120 °C on a glass slide the fluorescence changes from pink to blue (Fig. 9a). The PL data (Fig. 9b) confirm that the increase in temperature results in the disappearance of the Eu3+-based emission peaks (at 575, 590 and 615 nm). This provides further back up evidence that, at least in part, the increase in the thermomechanical sensitivity of these films is on account of the more thermally sensitive Eu3+ complexes. As the temperature is returned to ambient (by simply removing the film from the heat source) the pink colour reappears, consistent with the recomplexation of the Eu3+ to Mebip ligands. It should be noted that while there is a slight difference in emission colour between the film that is solution cast and the one that has been exposed to high temperatures, a subsequent heating and cooling cycle did not show any significant change in the emission spectra suggesting that once annealed this is a reversible process. This phenomenon suggests that these materials may be used as temperature sensors with reversible characteristics.26
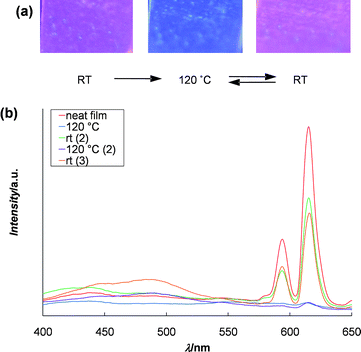 |
| Fig. 9 (a) Temperature sensing: sample heated to 120 °C resulting in quenching of the Eu3+ fluorescence and subsequent return upon cooling back to room temperature (Zn2+ : Eu3+ 70 : 30); (b) photoluminescence spectra (excited at 377 nm) of the above films showing the reversible disappearance of the Eu3+ metal-based emission at 590 and 615 nm upon heating and subsequent return with cooling. | |
Along with being temperature sensors, the materials' ability to act as chemical sensors was also investigated. As mentioned before, previous work has shown that small molecule analogues containing the Mebip ligand have the ability to detect nerve gas agent mimics.22 Using this knowledge, the 70 : 30 (Zn2+ : Eu3+) films were exposed to triethyl phosphate, the same nerve gas agent mimic used in the previous study. The first study simply dipped the film into a solution of triethyl phosphate in hexanes (10 mM). As can be seen in Fig. 10a, the Eu3+-based fluorescence is quenched only in the part of the film that was exposed to the solution. Similar results were also observed in the film that came into contact with liquid triethyl phosphate. This is consistent with the triethyl phosphate displacing the Eu3+ ion which results in the combined blue fluorescence from the Zn2+ : ligand complex and free ligand. We also wanted to explore the potential for these films to detect organophosphate vapours. To do this the film was exposed to a triethyl phosphate enriched environment. Fig. 10a shows the film after 24 h exposure which again shows the quenching of the Eu3+-based emission (Fig. 10b). The film after being exposed for 1 h showed similar changes, although the film's red fluorescence quickly returned upon removal from the triethyl phosphate-rich atmosphere, highlighting the reversibility of this process. Thus these studies show that the incorporation of Eu3+ into these metallo-supramolecular polymers also allows the films to be used as chemical sensors for detection of both liquid, solution and gaseous organophosphates.
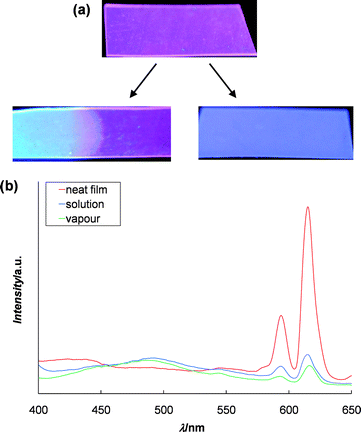 |
| Fig. 10 (a) Picture of a film prepared from 1 and Zn2+ : Eu3+ 70 : 30 fluorescing under UV light (λ = 365 nm) before and after dipping into a 10 mM solution of triethyl phosphate in hexane for 1 min (left) and exposure to triethyl phosphate vapour (24 h., rt) (right); (b) photoluminescence spectra (excited at 377 nm) of the above films. Both films show a decrease in the characteristic Eu3+ emission peaks at 590 and 615 nm. | |
Conclusions
The incorporation of Eu3+ metal ions into metallo-supramolecular polymers that consist of 4-oxy-2,6-bis-(1′-methylbenzimidazolyl)pyridine (Mebip) ditopic endcapped poly(tetrahydrofuran) with Zn2+ ions results in the formation of stimuli-responsive thermoplastic elastomer films. A series of these metallo-supramolecular polymer films were studied with differing ratios of Zn2+ to Eu3+ in order to determine the effect that an increase in Eu3+ has on the photoluminescent, thermomechanical and structural properties of these films. Increasing the films' Eu3+ content results in a change in fluorescence of the films from blue to red, which occurs as the blue ligand-based emission from the Mebip : Zn2+ complexes is replaced by the more intense red Eu3+-based emission from the Eu3+ : Mebip complexes. The films' mechanical properties show an enhanced sensitivity to temperature with increasing Eu3+ content and the Eu3+-based emission of these films is also sensitive to temperature as well as to chemicals known to displace the Eu3+ from the ligand. This stimuli-responsive behaviour combined with the relatively good mechanical properties of these films offer potential as flexible fluorescence-based temperature and chemical sensors. We are currently looking into this aspect in more detail.
Acknowledgements
This material is based upon work supported by the National Science Foundation under Grant no. CHE-0704026, DMR-0602869, DMR-0423914, and MRI-0821515 (for the purchase of the MALDI-TOF/TOF).
Notes and references
- J. -M. Lehn, Chem. Soc. Rev., 2007, 36, 151–160 RSC; M. J. Serpe and S. L. Craig, Langmuir, 2007, 23, 1626–1634 CrossRef CAS; A. Ciferri, Macromol. Rapid Commun., 2002, 23, 511–529 CrossRef CAS.
- G. ten Brinke, J. Ruokolainen and O. Ikkala, Adv. Polym. Sci., 2007, 207, 113–177 CAS; S. Rieth, C. Baddeley and J. D. Badjić, Soft Matter, 2007, 3, 137–154 RSC; P. M. Mendes, Chem. Soc. Rev., 2008, 37, 2512–2529 RSC; T. Gunnlaugsson and J. P. Leonard, Chem. Commun., 2005, 3114–3131 RSC.
- J. D. Fox and S. J. Rowan, Macromolecules, 2009, 42, 6823–6835 CrossRef CAS.
- V. A. Friese and D. G. Kurth, Coord. Chem. Rev., 2008, 252, 199–211 CAS; R. Dobrawa and F. Würthner, J. Polym. Sci., Part A: Polym. Chem., 2005, 43, 4981–4995 CrossRef CAS; H. Hofmeier and U. S. Schubert, Chem. Soc. Rev., 2004, 33, 373–399 RSC;
B. M. McKenzie and S. J. Rowan, Molecular Recognition and Polymers, ed. V. M. Rotello and S. Thayumanavan, John Wiley and Sons, Hoboken, NJ, 2008, ch. 7, pp. 157–178 Search PubMed; V. A. Friese and D. G. Kurth, Curr. Opin. Colloid Interface Sci., 2009, 14, 81–93 Search PubMed; S. Schmatloch, A. M. J. va den Berg, A. S. Alexeev, H. Hofmeier and U. S. Schubert, Macromolecules, 2003, 36, 9943–9949 CrossRef CAS.
- D. G. Kurth and M. Higuchi, Soft Matter, 2006, 2, 915–927 RSC.
- T. Vermonden, M. J. van Steenbergen, N. A. M. Besseling, A. T. M. Marcelis, W. E. Hennink, E. J. R. Sudhölter and M. A. Cohen Stuart, J. Am. Chem. Soc., 2004, 126, 15802–15808 CrossRef CAS; J. L. Bender, P. S. Corbin, C. L. Fraser, D. H. Metcalf, F. S. Richardson, E. L. Thomas and A. M. Urbas, J. Am. Chem. Soc., 2002, 124, 8526–8527 CrossRef CAS.
- H. L. Handl and R. J. Gillies, Life Sci., 2005, 77, 361–371 CrossRef CAS.
- K. Kuriki, Y. Koike and Y. Okamoto, Chem. Rev., 2002, 102, 2347–2356 CrossRef CAS.
-
K. Binnemans, Liquid-crystalline lanthanide complexes in Inorganic Chemistry in Focus II, ed. G. Meyer, D. Naumann and L. Wesemann, Wiley-VCH, Weinheim, 2005, ch. 15, pp. 267–291 Search PubMed; K. Binnemans and C. Goerller-Walrand, Chem. Rev., 2002, 102, 2303–2345 Search PubMed.
- J. Kido and Y. Okamoto, Chem. Rev., 2002, 102, 2357–2368 CrossRef CAS; R. J. Curry and W. P. Gillin, Curr. Opin. Solid State Mater. Sci., 2001, 5, 481–486 CrossRef CAS.
- J. -C. G. Bünzli and C. Piguet, Chem. Soc. Rev., 2005, 34, 1048–1077 RSC.
- P. Iyer, J. B. Beck, S. J. Rowan and C. Weder, Chem. Commun., 2005, 3, 319–321 Search PubMed; D. Knapton, S. J. Rowan and C. Weder, Macromolecules, 2006, 39, 651–657 CrossRef CAS.
- J. B. Beck, J. M. Ineman and S. J. Rowan, Macromolecules, 2005, 38, 5060–5068 CrossRef CAS.
- C. Piguet, A. F. Williams, G. Bernardinelli and J. -C. G. Bünzli, Inorg. Chem., 1993, 32, 4139–4149 CrossRef CAS.
- S. Lis, J. Alloys Compd., 2002, 341, 45–50 CrossRef CAS; N. Sabbatini, M. Guardigli and J. -M. Lehn, Coord. Chem. Rev., 1993, 123, 201–228 CrossRef CAS.
- S. Petoud, J. -C. G. Bünzli, T. Glanzman, C. Piguet, Q. Xiang and P. Thummel, J. Lumin., 1999, 82, 69–79 CrossRef CAS.
- A. Escande, L. Guénée, K. -L. Buchwalder and C. Piguet, Inorg. Chem., 2009, 48, 1132–1147 CrossRef CAS.
- C. Piguet, J.- C. G Bünzli, G. Bernardinelli, G. Hopfgartner and A. F. Williams, J. Alloys Compd., 1995, 225, 324–330 CrossRef CAS.
- C. -C. Chen and E. E. Dormidontova, J. Am. Chem. Soc., 2004, 126, 14972–14978 CrossRef CAS; S. H. Wang, C.- C. Chen and E. E. Dormidontova, Soft Matter, 2008, 4, 2039–2053 RSC.
- J. B. Beck and S. J. Rowan, J. Am. Chem. Soc., 2003, 125, 13922–13923 CrossRef CAS; Y. Zhao, J. B. Beck, S. J. Rowan and A. M. Jamieson, Macromolecules, 2004, 37, 3529–3531 CrossRef CAS; J. B. Beck and S. J. Rowan, Faraday Discuss., 2005, 128, 43–53 RSC; W. Weng, J. B. Beck, A. M. Jamieson and S. J. Rowan, J. Am. Chem. Soc., 2006, 128, 11663–11672 CrossRef CAS.
- For other examples of stimuli-responsive metallo-gels see: K. Kuroiwa, T. Shibata, A. Takada, N. Nemoto and N. Kimizuka, J. Am. Chem. Soc., 2004, 126, 2016–2021 Search PubMed; H. -J. Kim, W. -C. Zin and M. Lee, J. Am. Chem. Soc., 2004, 126, 7009–7014 CrossRef CAS; S. Kawano, N. Fujita and S. Shinkai, J. Am. Chem. Soc., 2004, 126, 8592–8593 CrossRef CAS; A. Kishimura, T. Yamishita and T. Aida, J. Am. Chem. Soc., 2005, 126, 179–183 CrossRef CAS.
- D. Knapton, M. Burnworth, S. J. Rowan and C. Weder, Angew. Chem., Int. Ed., 2006, 45, 5825–5829 CrossRef CAS.
- M. Burnworth, S. J. Rowan and C. Weder, Chem.–Eur. J., 2007, 13, 7828–7836 CrossRef CAS.
- P. Godlewska, L. Macalik and J. Hanuza, J. Alloys Compd., 2008, 451, 236–239 CrossRef CAS.
- T. Vermonden, J. van der Gucht, P. de Waard, A. T. M. Marcelis, N. A. M. Besseling, E. J. R. Sudhölter, G. J. Fleer and M. A. Cohen Stuart, Macromolecules, 2003, 36, 7035–7044 CrossRef CAS.
- J. Kunzelman, T. Chung, P. T. Mather and C. Weder, J. Mater. Chem., 2008, 18, 1082–1086 RSC.
Footnote |
† Electronic supplementary information (ESI) available: 1H NMR and 13C NMR spectra of 1, MALDI(MS–MS) of 1, PL spectra (solution), non-annealed DMTA, TGA and WAXS d-spacings of the metallo-supramolecular polymers. Photograph of a 70 : 30 (Zn2+ : Eu3+) film dipped into neat triethyl phosphate. Movie showing the elastic nature of the 80 : 20 (Zn2+ : Eu3+) films. See DOI: 10.1039/b915490f |
|
This journal is © The Royal Society of Chemistry 2010 |
Click here to see how this site uses Cookies. View our privacy policy here.