DOI:
10.1039/B914774H
(Paper)
Green Chem., 2010,
12, 135-143
Ruthenium-catalyzed synthesis of β-oxo esters in aqueous medium: Scope and limitations
Received 21st July 2009, Accepted 30th September 2009
First published on 28th October 2009
Abstract
The ability of the hydrosoluble ruthenium(II) complexes [RuCl2(η6-arene)(PTA)] 3a–d, [RuCl2(η6-arene)(PTA-Bn)] 4a–d, [RuCl2(η6-arene)(DAPTA)] 5a–d, [RuCl2(η6-arene)(TPPMS)] 6a–d (arene = C6H6, p-cymene, 1,3,5-C6H3Me3, C6Me6) to promote the atom-economic formation of β-oxo esters, by addition of carboxylic acids to terminal propargylic alcohols in water has been explored. Scope, limitations and catalyst recycling have been evaluated using the most active catalyst [RuCl2(η6-C6H6)(TPPMS)], 6a.
Introduction
β-Oxo esters are important intermediates in organic synthesis since they can be easily transformed into the corresponding α-hydroxy ketones,1 which are structural units present in a large variety of biologically active natural products.2 Several procedures are presently available to prepare these types of compounds, such as the carbonylation of α-halo ketones,3 the anodic oxidation of enol acetates,4 the Cu catalyzed insertion of α-diazo ketones into the O–H bond of carboxylic acids,5 the two-step hydration/esterification of propargylic alcohols6 or the oxidation of ketones with metal–acetate complexes.7 However, the scope of most of these methods is rather low, and they also suffer from environmental problems associated with the use of harmful reagents.The catalytic addition of carboxylic acids to terminal propargylic alcohols, 1, represents an appealing and elegant alternative to β-oxo esters, 2 (Scheme 1); an atom-economic transformation that can be efficiently promoted by ruthenium complexes.8 Among the different catalysts employed, the best results in terms of activity and selectivity have been described using the mononuclear arene–Ru(II) derivatives [RuCl2(η6-arene)(PR3)] (arene = p-cymene, C6Me6; PR3 = PPh3, PMe3, phosphoramidite),9 the dimeric complex [{Ru(μ-O2CH)(CO)2(PPh3)}2]10 and the catalytic system composed of [Ru(η5-C8H11)2] (C8H11 = cyclooctadienyl), a trialkyl phosphine and maleic anhydride.11
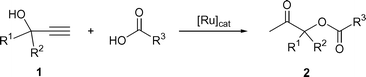 |
| Scheme 1 β-Oxo ester formation by addition of carboxylic acids to terminal propargylic alcohols | |
From a mechanistic point of view, these catalytic transformations are based on the well-known ability of ruthenium complexes to promote the addition of carboxylic acids to the C
C bond of terminal alkynes to afford enol esters.12,13 Thus, after the initial Markovnikov addition of the carboxylate anion to the π-alkyne intermediate A, intramolecular transesterification of the resulting enol ester complex B readily takes place, leading to the alkenyl derivative C. Final protonolysis of C liberates the β-oxo ester 2 and regenerates the catalytically active ruthenium species (Scheme 2).
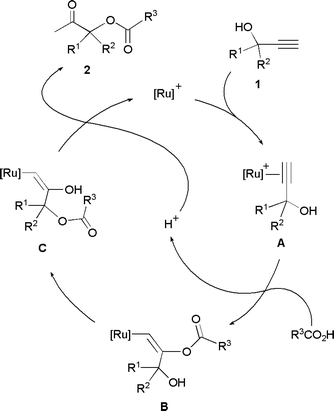 |
| Scheme 2 Proposed mechanism for the Ru-catalyzed β-oxo ester formation reactions | |
On the other hand, a crucial factor in realizing a “green chemical” process involves the choice of a safe, non-toxic and cheap solvent.14 Water is undoubtedly one of the most appealing candidates.15 Therefore, the development of organic transformations in aqueous media has become one of the major cornerstones in modern chemistry.16 Following this general trend, the design of novel transition metal catalysts for organic reactions in water has attracted a growing interest in recent years,17 disclosing a wide variety of highly efficient and selective synthetic approaches to date.16,17 In this context, in the course of our current studies directed toward the application of ruthenium catalysts in aqueous organic synthesis,18 we have recently reported the preparation of half-sandwich ruthenium(II) complexes [RuCl2(η6-arene)(PR3)] containing the hydrosoluble phosphine ligands PTA (3a–d), PTA-Bn (4a–d), DAPTA (5a–d) and TPPMS (6a–d) (see Fig. 1),19 also showing that some of them are excellent precatalysts for the selective hydration of organonitriles to amides in aqueous medium under neutral conditions.20 The availability of these compounds, along with the known effectiveness of mononuclear arene–Ru(II) derivatives [RuCl2(η6-arene)(PR3)] in β-oxo ester formation by addition of carboxylic acids to alkynols,9 prompted us to study this atom-economic transformation in water. To the best of our knowledge, no precedents on the use of environmentally benign aqueous media in these catalytic addition reactions have been described previously.
Results and discussion
Table 1 provides a summary of our catalyst screening. We used the addition of benzoic acid to the commercially available alkynol 1-phenyl-2-propyn-1-ol 1a as a model reaction. In a typical experiment, the alkynol (1 mmol) and the acid (1 mmol) were suspended in water (1 mL), under a nitrogen atmosphere, and treated with 2 mol% of the corresponding ruthenium complex 3a–6d at 60 °C for 24 h. Under these conditions, all the complexes checked were found to be active catalysts in the addition process providing the β-oxo ester 1-phenyl-2-oxopropyl benzoate 2a in moderate to good yields. Interestingly, the nature of the hydrosoluble phosphine played an important role in both the efficiency and selectivity of the process.21 Thus, the best results were obtained with complexes 6a–d, all bearing the sulfonated ligand TPPMS, which led to the selective formation of 2a in 76–87% GC yields (entries 13–16). In contrast, the use of the PTA, PTA-Bn and DAPTA-based catalysts 3a–5d (entries 1–12) resulted in lower yields of 2a (14–71%); in most of these reactions the formation of minor amounts of styrene as by-product was also observed (entries 1–5 and 9–11).
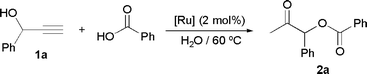
|
---|
Entry | Catalyst | Time/h | Yieldb/% |
---|
Reactions performed under N2 atmosphere at 60 °C using 1 mmol of 1-phenyl-2-propyn-1-ol (1 M in water) and 1 mmol of benzoic acid. Determined by GC. 5% of styrene is formed. 10% of styrene is formed. 7% of styrene is formed. 1% of styrene is formed. 2% of styrene is formed. 4% of styrene is formed. Reaction performed at 100 °C. |
---|
1 | [RuCl2(η6-C6H6)(PTA)] 3a | 24 | 64c |
2 | [RuCl2(η6-p-cymene)(PTA)] 3b | 24 | 39d |
3 | [RuCl2(η6-1,3,5-C6H3Me3)(PTA)] 3c | 24 | 54e |
4 | [RuCl2(η6-C6Me6)(PTA)] 3d | 24 | 71c |
5 | [RuCl2(η6-C6H6)(PTA-Bn)] 4a | 24 | 38f |
6 | [RuCl2(η6-p-cymene)(PTA-Bn)] 4b | 24 | 63 |
7 | [RuCl2(η6-1,3,5-C6H3Me3)(PTA-Bn)] 4c | 24 | 30 |
8 | [RuCl2(η6-C6Me6)(PTA-Bn)] 4d | 24 | 14 |
9 | [RuCl2(η6-C6H6)(DAPTA)] 5a | 24 | 62g |
10 | [RuCl2(η6-p-cymene)(DAPTA)] 5b | 24 | 50e |
11 | [RuCl2(η6-1,3,5-C6H3Me3)(DAPTA)] 5c | 24 | 61h |
12 | [RuCl2(η6-C6Me6)(DAPTA)] 5d | 24 | 56g |
13 | [RuCl2(η6-C6H6)(TPPMS)] 6a | 24 | 87 |
14 | [RuCl2(η6-p-cymene)(TPPMS)] 6b | 24 | 85 |
15 | [RuCl2(η6-1,3,5-C6H3Me3)(TPPMS)] 6c | 24 | 79 |
16 | [RuCl2(η6-C6Me6)(TPPMS)] 6d | 24 | 76 |
17i | [RuCl2(η6-C6H6)(TPPMS)] 6a | 3 | 95 |
Formation of alkene side products, arising from the cleavage of the C
C bond of the alkynol, has been recently observed in related addition reactions promoted by (η6-arene)ruthenium(II)-phosphoramidite catalysts in organic media.9b Such a competitive process involves the generation of a highly reactive allenylidene–ruthenium intermediate E (Scheme 3) as the result of an initial tautomerization of the π-alkyne complex A into the 3-hydroxy-vinylidene D, and subsequent dehydration of D. Then, the carbon–carbon cleavage process takes place via nucleophilic addition of water to the electrophilic α-carbon of the allenylidene chain, a well-known transformation in the chemistry of metal–allenylidenes.22–24
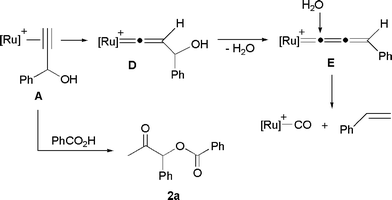 |
| Scheme 3 Reaction pathway explaining the formation of styrene | |
It is known that, due to its π-accepting properties, the allenylidene ligand formation is favored by electron-rich metal fragments.22 This fact could explain why the occurrence of styrene takes place using those catalysts bearing the aliphatic phosphines PTA, PTA-Bn and DAPTA, all of them more basic than the aromatic sulfonated one, TPPMS. From this general catalyst screening, the benzene derivative [RuCl2(η6-C6H6)(TPPMS)] 6a emerged as the top choice due to its selectivity and efficiency (87% GC yield of 2a after 24 h; entry 13). In addition, both the yield and the rate of the process could be significantly improved by increasing the working temperature from 60 to 100 °C. Under these new reaction conditions, using the same catalyst loading (2 mol% of 6a), the β-oxo ester 2a was formed in 95% GC yield after only 3 h (entry 17). No appreciable difference in activity was observed when an organic solvent (toluene) was used instead of water. Subsequent purification by column chromatography on silica gel provided an analytically pure sample of 2a in 88% isolated yield.
Under these optimized reaction conditions (1 M solution of the alkynol in water; [alkynol]
:
[6a]
:
[PhCO2H] ratio = 50
:
1
:
50; 100 °C) the ability of 6a to promote the addition of benzoic acid to a number of other terminal propargylic alcohols was explored. The results are summarized in Table 2. Thus, as observed for 1a (entry 1), other aromatic (1b–j; entries 2–10) and aliphatic (1k–n; entries 11–14) secondary alkynols could be converted into the corresponding β-oxo esters 2b–n in moderate to good yields (36–84% GC yields; 25–78% isolated yields) after 3–6 h of heating. Concerning the aromatic substrates, an influence of the electronic properties of the aryl rings on the efficiency of the process was observed. Thus, alkynols with electron-donating groups showed less reactivity (entries 8–10) compared to substrates with electron-withdrawing functionalities (entries 4–7), even when, in cases of the latter, the minor formation of olefinic by-products, via the competitive C
C bond scission process discussed above was observed. As expected,9–11 this addition process is not restricted to secondary alkynols. Thus, as shown in entry 15, propargylic alcohol itself, 1o, can also participate in this transformation leading to the known 2-oxopropyl benzoate (2o)9a in 60% isolated yield after only 3 h. Regarding tertiary alcohols (entries 16–22), the best results were obtained with 1-ethynyl-cycloalkanols (1q–s; entries 17–19) from which the corresponding β-oxo esters 2q–s could be synthesized in excellent yields (85–90% isolated yield; entries 17–19). The process is also operative starting from 2-phenyl-3-butyn-2-ol (1p; entry 15) and 2-ethynyl-2-adamantanol (1t; entry 20). However, its efficiency was found to be lower compared to the precedent cases (55–57% yield). Solvent removal and chromatographic work-up on silica gel provided analytically pure samples of all these β-oxo esters, which were fully characterized by means of standard analytical and spectroscopic techniques (see the Experimental section).
Table 2 Ruthenium-catalyzed synthesis of β-oxo esters in water: generality on the propargylic alcohola

|
---|
Entry | Propargylic alcohol 1 | Time/h | Yield of 2b/% |
---|
Reactions performed under N2 atmosphere at 100 °C using 1 mmol of the corresponding propargylic alcohol (1 M in water) and 1 mmol of benzoic acid. Determined by GC. Isolated yields are given in brackets. 15% of 1-vinyl-naphthalene is formed. 10% of 2-vinyl-naphthalene is formed. 4% of 2-chloro-styrene is formed. 6% of 3-chloro-styrene is formed. 6% of 4-chloro-styrene is formed. 14% of 2,3,4,5,6-pentafluoro-styrene is formed. 7% of allyl-benzene is formed. 4% of (1-methylallyl)-benzene is formed. 5% of 2-methylene-adamantane is formed. 80% of 1,1-diphenyl-ethene is formed. 63% of 1,1-bis(4-chlorophenyl)-ethene is formed. |
---|
1 | R1 = H, R2 = Ph 1a | 3 | 2a; 95 (88) |
2 | R1 = H, R2 = 1-naphthyl 1b | 3 | 2b; 76 (69)c |
3 | R1 = H, R2 = 2-naphthyl 1c | 3 | 2c; 72 (63)d |
4 | R1 = H, R2 = 2-C6H4Cl 1d | 6 | 2d; 84 (78)e |
5 | R1 = H, R2 = 3-C6H4Cl 1e | 6 | 2e; 75 (63)f |
6 | R1 = H, R2 = 4-C6H4Cl 1f | 6 | 2f; 75 (65)g |
7 | R1 = H, R2 = C6F51g | 6 | 2g; 71 (60)h |
8 | R1 = H, R2 = 2-C6H4OMe 1h | 6 | 2h; 57 (48) |
9 | R1 = H, R2 = 3-C6H4OMe 1i | 6 | 2i; 59 (51) |
10 | R1 = H, R2 = 4-C6H4OMe 1j | 6 | 2j; 36 (25) |
11 | R1 = H, R2 = Me 1k | 3 | 2k; 67 (59) |
12 | R1 = H, R2 = CH2Ph 1l | 3 | 2l; 82 (77)i |
13 | R1 = H, R2 = CH2CH2Ph 1m | 6 | 2m; 80 (72) |
14 | R1 = H, R2 = C(H)MePh 1n | 6 | 2n; 68 (63)j |
15 | R1 = R2 = H 1o | 3 | 2o; 71 (60) |
16 | R1 = Me, R2 = Ph 1p | 6 | 2p; 65 (55) |
17 | R1R2 = –(CH2)4–1q | 2 | 2q; 99 (90) |
18 | R1R2 = –(CH2)5–1r | 3 | 2r; 99 (88) |
19 | R1R2 = –(CH2)6–1s | 3 | 2s; 92 (85) |
20 | R1R2 = Adamantane-2,2-diyl 1t | 8 | 2t; 63 (57)k |
21 | R1 = R2 = Ph 1u | 3 | 2u; 3l |
22 | R1 = R2 = 4-C6H4Cl 1v | 3 | 2v; 8m |
Remarkably, when the addition of PhCO2H to tertiary diaromatic substrates, such as 1,1-diphenyl-2-propyn-1-ol (1u; entry 21) or 1,1-bis(4-chlorophenyl)-2-propyn-1-ol (1v; entry 22), was attempted, only trace amounts of the expected β-oxo esters could be detected by GC. In these cases, the reactions gave the major products 1,1-diphenyl-ethene (80%) and 1,1-bis(4-chlorophenyl)-ethene (63%), respectively.
We must also mention that, despite the ability of complex 6a to promote the addition of benzoic acid to 1-ethynyl-cycloalkanols 1q–s (entries 17–19), attempts to synthesize β-oxo esters derived from the hormonal steroids ethisterone, 1x, and mestranol, 1y, also failed.25 Thus, we found that treatment of 1x–y with 1 equivalent of PhCO2H, under the same catalytic conditions described above, led to the slow formation (48–60 h) of the known 17,17-dimethyl-18-norandrosta-4,13-dien-3-one 726 and 3-methoxy-17,17-dimethyl-gona-1,3,5(10),13-tetraene 8,27 respectively, isolated in moderate yields (50–68%) after appropriate chromatographic workup (Scheme 4). Compounds 7–8 most probably result from the initial ruthenium-catalyzed dehydration and cleavage of the 2-propyn-1-ol function, via the corresponding allenylidene intermediates,28 which affords the olefinic intermediates F. Then, a classical acid-promoted Wagner–Meerwein rearrangement can take place leading to the final steroids 7–8.26,27 All these results seem to indicate that the competitive π-alkynol to allenylidene rearrangement is governed not only by the electronic nature of the catalyst, but also by the steric requirements of the propargylic alcohol substituents, with bulky substrates clearly favoring this undesirable side reaction.
Once the generality of this aqueous transformation with respect to the propargylic alcohol had been evaluated, the scope regarding the nature of the carboxylic acid was explored. Results are collected in Fig. 2.
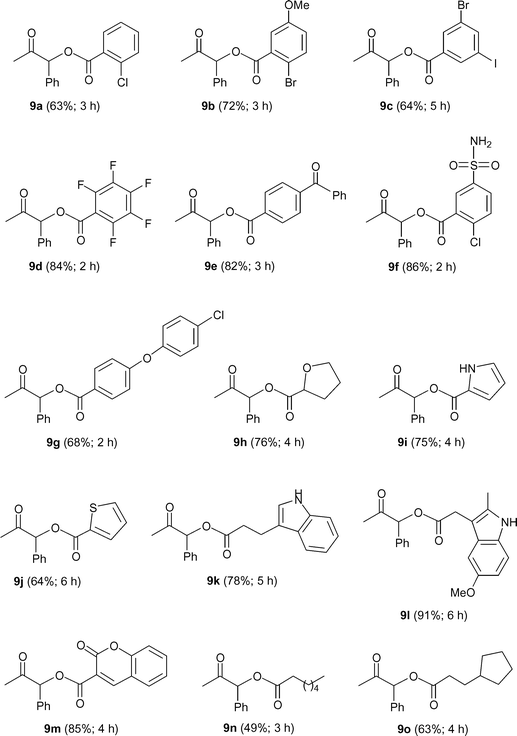 |
| Fig. 2 Structures of the β-oxo esters 9a–o. | |
Thus, we have found that using alkynol 1a as a model compound, several aromatic acids, bearing functional groups such as halide, alkoxy, ketone or sulfonamide, can be successfully employed in this addition process, affording the corresponding β-oxo esters 9a–g in good isolated yields (63–86%). Interestingly, the reaction is also compatible with heteroaromatic substrates. Thus, carboxylic acids derived from tetrahydrofuran, pyrrole, thiophene, indole or 2-oxo-2H-chromene were efficiently (64–91% yield) converted into the novel β-oxo esters 9h–m. In addition, the clean formation of 9n–o, starting from the aliphatic heptanoic and 3-cyclopentyl-propionic acid confirmed the wide scope of this catalytic transformation.
Finally, the catalyst recycling has also been investigated using the addition of benzoic acid to 1-phenyl-2-propyn-1-ol 1a as model reaction (entry 1 in Table 2). Thus, we have found that after a simple extraction of the final product 2a with hexane, the aqueous phase, containing [RuCl2(η6-C6H6)(TPPMS)] 6a, can be re-used in at least two consecutive runs. However, an important decrease of the activity was observed in the third cycle (91% GC yield after 24 h) due to the partial decomposition of the catalyst (release of the C6H6 ligand was observed by GC after prolonged periods in solution at 100 °C).
Conclusions
We have demonstrated that β-oxo ester formation, by catalytic addition of carboxylic acids to terminal propargylic alcohols, can be conveniently performed in environmentally benign aqueous medium using the water-soluble ruthenium(II) complex [RuCl2(η6-C6H6)(TPPMS)] 6a. The process showed a remarkably wide scope, tolerating the presence of several functional groups in the substrates. In fact, its only limitation concerns the use of bulky tertiary alkynols which, under the catalytic conditions employed, undergo hydrolytic cleavage of the C
C triple bond instead of the desired addition. In summary, the atom-economic nature of this catalytic transformation, which allows access to valuable synthetic intermediates from readily accessible or commercially available starting materials, along with the remarkable activity of 6a, confer a genuine potential for practical application in organic chemistry to the aqueous protocol reported herein.Experimental section
General methods
Infrared spectra were recorded on a Perkin-Elmer 1720-XFT spectrometer. NMR spectra were recorded on a Bruker DPX-300 instrument at 300 MHz (1H), 75.4 MHz (13C) or 282.4 (19F). The chemical shift values (δ) are given in parts per million and are referred to the residual peak of the deuterated solvent used (CDCl3). DEPT experiments have been carried out for all the compounds reported. GC/MS measurements were performed on a Agilent 6890 N equipment coupled to a 5973 mass detector (70eV electron impact ionization) using a HP-1MS column. ESI-TOF high-resolution mass spectra were provided by the mass spectrometry service of the University of Santiago de Compostela. Elemental analyses were performed with a Perkin-Elmer 2400 microanalyzer. Flash chromatography was performed using Merck silica gel 60 (230-400 mesh). Propargylic alcohols 1a–y were obtained from commercial suppliers or synthesized by following the classical Midland's procedure.29 Ruthenium(II) complexes 3a–6d were prepared by following the methods reported in the literature.20,30General procedure for the catalytic reactions. Under nitrogen atmosphere, water (1 cm3), the corresponding propargylic alcohol (1 mmol), carboxylic acid (1 mmol), and the ruthenium catalyst 6a (12 mg, 0.02 mmol) were introduced into a sealed tube and the resulting reaction mixture stirred at 100 °C for the time indicated (see Table 2, Schemes 3 and 4, or Fig. 2). The course of the reaction was monitored by regular sampling and analysis by GC. After elimination of the solvent under reduced pressure, the crude reaction mixture was purified by column chromatography over silica gel using an ethyl acetate–hexane mixture (1
:
10 v/v) as eluent. Compounds 2a,312k,322o,9a2p,9b2q,9b2r,9b726 and 827 have been previously described in the literature. Characterization data for the novel β-oxo esters synthesized in this work are as follows: 1-(1-Naphthyl)-2-oxopropyl benzoate 2b. Pale yellow solid; 1H NMR (300.1 MHz, CDCl3): δ 2.16 (s, 3H), 6.88 (s, 1H), 7.41–7.71 (m, 7H), 7.91–7.95 (m, 3H), 8.01–8.12 (m, 2H) ppm; 13C{1H} NMR (75.47 MHz, CDCl3): δ 26.3, 80.0, 123.9, 125.3, 126.2, 127.2, 128.3, 128.4, 128.9, 129.2, 129.5, 129.9, 130.3, 131.3, 133.4, 134.2, 165.7, 201.8 ppm; IR (nujol): ν 1716 and 1732 (C
O) cm−1; GC-MS (EI, 70eV): m/z 304 (30%, M+), 261 (30), 105 (100), 77 (25); Elemental analysis calcd (%) for C20H16O3: C 78.93, H 5.30; found: C 78.81, H 5.23. 1-(2-Naphthyl)-2-oxopropyl benzoate 2c. Pale yellow solid; 1H NMR (300.1 MHz, CDCl3): δ 2.24 (s, 3H), 6.38 (s, 1H), 7.45–7.63 (m, 6H), 7.89–7.94 (m, 3H), 8.02–8.17 (m, 3H) ppm; 13C{1H} NMR (75.47 MHz, CDCl3): δ 26.2, 81.4, 124.7, 126.7, 126.9, 127.7, 127.8, 128.1, 128.4, 129.1, 129.2, 129.9, 130.6, 131.1, 133.4, 133.5, 165.8, 201.8 ppm; IR (nujol): ν 1721 and 1738 (C
O) cm−1; GC-MS (EI, 70eV): m/z 304 (30%, M+), 261 (30), 105 (100), 77 (25); Elemental analysis calcd (%) for C20H16O3: C 78.93, H 5.30; found: C 78.77, H 5.36. 1-(2-Chlorophenyl)-2-oxopropyl benzoate 2d. Colourless oil; 1H NMR (300.1 MHz, CDCl3): δ 2.26 (s, 3H), 6.74 (s, 1H), 7.33–7.37 (m, 2H), 7.42–7.49 (m, 3H), 7.53–7.60 (m, 2H), 8.09–8.12 (m, 2H) ppm; 13C{1H} NMR (75.47 MHz, CDCl3): δ 26.8, 77.4, 127.5, 128.4, 129.1, 129.8, 129.9, 130.1, 130.6, 131.7, 133.5, 133.9, 165.5, 200.1 ppm; IR (neat): ν 1731 and 1785 (C
O) cm−1; GC-MS (EI, 70eV): m/z 253 (3%, M+− Cl), 245 (10), 211 (5), 105 (100), 77 (30); HRMS (ESI-TOF): m/z 289.063254 [M+ + H], C16H14O3Cl requires 289.063147. 1-(3-Chlorophenyl)-2-oxopropyl benzoate 2e. Colourless oil; 1H NMR (300.1 MHz, CDCl3): δ 2.22 (s, 3H), 6.16 (s, 1H), 7.30–7.43 (m, 4H), 7.45–7.61 (m, 3H), 8.10–8.12 (m, 2H) ppm; 13C{1H} NMR (75.47 MHz, CDCl3): δ 26.1, 80.4, 125.9, 127.7, 128.5, 128.9, 129.5, 129.9, 130.3, 133.6, 134.9, 135.2, 165.5, 201.4 ppm; IR (neat): ν 1706 and 1732 (C
O) cm−1; GC-MS (EI, 70eV): m/z 288 (1%, M+), 245 (10), 211 (2), 105 (100), 77 (30); HRMS (ESI-TOF): m/z 289.063007 [M+ + H], C16H14O3Cl requires 289.063147. 1-(4-Chlorophenyl)-2-oxopropyl benzoate 2f. Colourless oil; 1H NMR (300.1 MHz, CDCl3): δ 2.21 (s, 3H), 6.19 (s, 1H), 7.41–7.49 (m, 4H), 7.58–7.61 (m, 3H), 8.11–8.13 (m, 2H) ppm; 13C{1H} NMR (75.47 MHz, CDCl3): δ 26.1, 80.5, 128.6, 129.2, 129.4, 129.9, 132.0, 132.1, 135.2, 135.5, 135.9, 165.7, 201.6 ppm; IR (neat): ν 1716 and 1737 (C
O) cm−1; GC-MS (EI, 70eV): m/z 288 (1%, M+), 245 (10), 105 (100), 77 (30); HRMS (ESI-TOF): m/z 289.062991 [M+ + H], C16H14O3Cl requires 289.063147. 1-Pentafluorophenyl-2-oxopropyl benzoate 2g. Orange oil; 1H NMR (300.1 MHz, CDCl3): δ 2.43 (s, 3H), 6.68 (s, 1H), 7.48–7.53 (m, 2H), 7.63–7.68 (m, 1H), 8.10–8.13 (m, 2H) ppm; 13C{1H} NMR (75.47 MHz, CDCl3): δ 26.4, 70.3, 109.8 (m), 128.5, 128.7, 130.2, 134.1, 134.2, 135.8-147.3 (m), 164.9, 200.1 ppm; 19F NMR (282.4 MHz, CDCl3): δ−160.8 (m, 2F), −151.2 (t, J = 22.0 Hz, 1F), −136.6 (m, 2F) ppm; IR (neat): ν 1712 and 1731 (C
O) cm−1; GC-MS (EI, 70eV): m/z 344 (1%, M+), 301 (30), 105 (100), 77 (25); HRMS (ESI-TOF): m/z 345.054946 [M+ + H], C16H10F5O3 requires 345.055011. 1-(2-Methoxyphenyl)-2-oxopropyl benzoate 2h. Colourless oil; 1H NMR (300.1 MHz, CDCl3): δ 2.20 (s, 3H), 3.92 (s, 3H), 6.70 (s, 1H), 6.87–7.02 (m, 4H), 7.28–7.62 (m, 3H), 8.09–8.14 (m, 2H) ppm; 13C{1H} NMR (75.47 MHz, CDCl3): δ 26.3, 55.6, 75.2, 111.1, 120.6, 121.1, 128.3, 128.4, 129.4, 129.9, 133.1, 133.7, 156.9, 171.7, 202.1 ppm; IR (neat): ν 1683 and 1716 (C
O) cm−1; GC-MS (EI, 70eV): m/z 284 (1%, M+), 241 (10), 105 (100), 77 (30); HRMS (ESI-TOF): m/z 285.112721 [M+ + H], C17H17O4 requires 285.112684. 1-(3-Methoxyphenyl)-2-oxopropyl benzoate 2i. Colourless oil; 1H NMR (300.1 MHz, CDCl3): δ 2.21 (s, 3H), 3.84 (s, 3H), 6.18 (s, 1H), 6.94–7.12 (m, 4H), 7.35–7.61 (m, 3H), 8.11–8.14 (m, 2H) ppm; 13C{1H} NMR (75.47 MHz, CDCl3): δ 25.9, 55.3, 81.1, 113.4, 114.7, 120.2, 128.4, 129.2, 129.9, 130.1, 133.4, 133.7, 159.9, 165.7, 201.7 ppm; IR (neat): ν 1704 and 1732 (C
O) cm−1; GC-MS (EI, 70eV): m/z 284 (1%, M+), 241 (10), 105 (100), 77 (30); HRMS (ESI-TOF): m/z 285.112563 [M+ + H], C17H17O4 requires 285.112684. 1-(4-Methoxyphenyl)-2-oxopropyl benzoate 2j. Colourless oil; 1H NMR (300.1 MHz, CDCl3): δ 2.19 (s, 3H), 3.86 (s, 3H), 6.17 (s, 1H), 6.82–7.11 (m, 4H), 7.43–7.64 (m, 3H), 8.09–8.14 (m, 2H) ppm; 13C{1H} NMR (75.47 MHz, CDCl3): δ 25.6, 55.1, 80.7, 114.0, 127.9, 129.1, 129.6, 129.8, 133.0, 133.6, 159.9, 171.9, 201.7 ppm; IR (neat): ν 1709 and 1738 (C
O) cm−1; GC-MS (EI, 70eV): m/z 284 (1%, M+), 241 (10), 105 (100), 77 (30); HRMS (ESI-TOF): m/z 285.112687 [M+ + H], C17H17O4 requires 285.112684. 1-Benzyl-2-oxopropyl benzoate 2l. Colourless oil; 1H NMR (300.1 MHz, CDCl3): δ 2.14 (s, 3H), 3.22 (m, 2H), 5.45 (dd, J = 7.7 and 5.1 Hz, 1H), 7.23–7.35 (m, 5H), 7.43–7.61 (m, 3H), 8.04 (m, 2H) ppm; 13C{1H} NMR (75.47 MHz, CDCl3): δ 26.9, 36.9, 79.5, 127.1, 128.5, 128.6, 129.1, 129.4, 129.7, 133.5, 135.8, 165.9, 205.7 ppm; IR (neat): ν 1720 (br, C
O) cm−1; GC-MS (EI, 70eV): m/z 146 (40%, M+− PhCO2), 105 (100), 77 (40); HRMS (ESI-TOF): m/z 269.117855 [M+ + H], C17H17O3 requires 269.117770. 1-Phenethyl-2-oxopropyl benzoate 2m. Colourless oil; 1H NMR (300.1 MHz, CDCl3): δ 2.24 (s, 3H), 2.30 (m, 2H), 2.85 (m, 2H), 5.27 (dd, J = 6.0 and 6.0 Hz, 1H), 7.22–7.35 (m, 5H), 7.49–7.54 (m, 2H), 7.65 (m, 1H), 8.13 (m, 2H) ppm; 13C{1H} NMR (75.47 MHz, CDCl3): δ 26.2, 31.6, 32.1, 78.5, 126.4, 128.5, 128.6, 128.7, 129.3, 129.8, 133.5, 140.4, 166.0, 205.4 ppm; IR (neat): ν 1716 and 1734 (C
O) cm−1; GC-MS (EI, 70eV): m/z 282 (1%, M+), 105 (100), 77 (25); HRMS (ESI-TOF): m/z 283.133166 [M+ + H], C18H19O3 requires 283.133420. 1-(1-Phenylethyl)-2-oxopropyl benzoate 2n. Colourless oil; 1H NMR (300.1 MHz, CDCl3): δ 1.51 (d, J = 6.0 Hz, 3H), 2.00 (s, 3H), 3.45 (m, 1H), 5.31 (d, J = 5.7 Hz, 1H), 7.26–7.35 (m, 5H), 7.47–7.65 (m, 3H), 8.10 (m, 2H) ppm; 13C{1H} NMR (75.47 MHz, CDCl3): δ 16.1, 27.9, 41.2, 82.8, 127.7, 127.8, 128.4, 128.5, 128.7, 129.8, 133.5, 141.3, 166.0, 205.6 ppm; IR (neat): ν 1721 and 1743 (C
O) cm−1; GC-MS (EI, 70eV): m/z 239 (1%, M+− MeCO), 161 (15), 105 (100), 77 (25); HRMS (ESI-TOF): m/z 283.133276 [M+ + H], C18H19O3 requires 283.133420. 1-Acetyl-cycloheptyl benzoate 2s. Colourless oil; 1H NMR (300.1 MHz, CDCl3): δ 1.58–1.64 (m, 7H), 2.08–2.16 (m, 3H), 2.12 (s, 3H), 2.23–2.33 (m, 2H), 7.44–7.62 (m, 3H), 8.05 (m, 2H) ppm; 13C{1H} NMR (75.47 MHz, CDCl3): δ 21.3, 23.6, 24.8, 27.7, 29.3, 89.3, 128.4, 129.7, 129.8, 133.3, 165.5, 206.9 ppm; IR (neat): ν 1716 (br, C
O) cm−1; GC-MS (EI, 70eV): m/z 231 (5%, M+− 2CH2), 105 (100), 77 (20); HRMS (ESI-TOF): m/z 261.148865 [M+ + H], C16H21O3 requires 261.149070. 2-Acetyl-adamantan-2-yl benzoate 2t. Yellow oil; 1H NMR (300.1 MHz, CDCl3): δ 1.70–1.91 (m, 8H), 2.13 (s, 3H), 2.22 (m, 4H), 2.57 (br, 2H), 7.47–7.64 (m, 3H), 8.10 (d, J = 7.2 Hz, 2H) ppm; 13C{1H} NMR (75.47 MHz, CDCl3): δ 24.1, 26.8, 26.9, 32.6, 33.7, 33.9, 37.7, 88.8, 128.6, 129.8, 130.0, 133.4, 165.2, 205.9 ppm; IR (neat): ν 1673 and 1722 (C
O) cm−1; GC-MS (EI, 70eV): m/z 255 (20%, M+− MeCO), 105 (100), 77 (30); HRMS (ESI-TOF): m/z 299.164630 [M+ + H], C19H23O3 requires 299.164720. 1-Phenyl-2-oxopropyl 2-chlorobenzoate 9a. Pale yellow oil; 1H NMR (300.1 MHz, CDCl3): δ 2.21 (s, 3H), 6.22 (s, 1H), 7.31–7.54 (m, 8H), 8.00 (m, 1H) ppm; 13C{1H} NMR (75.47 MHz, CDCl3): δ 25.7, 81.3, 126.2, 127.6, 128.4, 128.7, 129.0, 130.7, 131.6, 132.5, 132.7, 133.8, 164.2, 200.9 ppm; IR (neat): ν 1738 (br, C
O) cm−1; GC-MS (EI, 70eV): m/z 245 (10%, M+− COMe), 139 (100), 111 (20), 75 (15); HRMS (ESI-TOF): m/z 289.063188 [M+ + H], C16H14O3Cl requires 289.063147. 1-Phenyl-2-oxopropyl 2-bromo-5-methoxybenzoate 9b. Orange oil; 1H NMR (300.1 MHz, CDCl3): δ 2.22 (s, 3H), 3.84 (s, 3H), 6.22 (s, 1H), 6.92 (dd, J = 8.7 and 3.1 Hz, 1H), 7.43–7.58 (m, 7H) ppm; 13C{1H} NMR (75.47 MHz, CDCl3): δ 26.2, 55.7, 81.9, 112.3, 117.2, 119.2, 128.1, 129.1, 129.5, 131.6, 132.8, 135.2, 158.6, 165.1, 201.2 ppm; IR (neat): ν 1730 (br, C
O) cm−1; GC-MS (EI, 70eV): m/z 364 (3%, M+), 321 (10), 213 (100), 187 (10), 170 (10); HRMS (ESI-TOF): m/z 363.023211 [M+ + H], C17H16O4Br requires 363.023202. 1-Phenyl-2-oxopropyl 3-bromo-5-iodobenzoate 9c. Orange oil; 1H NMR (300.1 MHz, CDCl3): δ 2.19 (s, 3H), 6.21 (s, 1H), 7.46–7.53 (m, 5H), 8.07 (dd, J = 1.7 and 1.7 Hz, 1H), 8.19 (dd, J = 1.7 and 1.7 Hz, 1H), 8.35 (dd, J = 1.7 and 1.7 Hz, 1H) ppm; 13C{1H} NMR (75.47 MHz, CDCl3): δ 26.2, 81.8, 94.0, 123.0, 128.2, 129.3, 129.7, 132.1, 132.4, 132.6, 137.3, 144.2, 163.1, 200.6 ppm; IR (neat): ν 1716 and 1738 (C
O) cm−1; GC-MS (EI, 70eV): m/z 415 (20%, M+− MeCO), 309 (100), 281 (15), 182 (10), 156 (15); HRMS (ESI-TOF): m/z 458.909398 [M+ + H], C16H13O3BrI requires 458.909284. 1-Phenyl-2-oxopropyl pentafluorobenzoate 9d. Orange oil; 1H NMR (300.1 MHz, CDCl3): δ 2.18 (s, 3H), 6.23 (s, 1H), 7.42-7.54 (m, 5H) ppm; 13C{1H} NMR (75.47 MHz, CDCl3): δ 28.0, 84.7, 130.0, 130.7-134.1 (m), 138.2-149.7 (m), 160.3, 201.9 ppm; 19F NMR (282.4 MHz, CDCl3): δ−160.1 (m, 2F), −147.2 (m, 1F), −136.6 (m, 2F) ppm; IR (neat): ν 1739 (br, C
O) cm−1; GC-MS (EI, 70eV): m/z 301 (20%, M+− MeCO), 195 (100), 167 (10), 117 (5), 105 (5); HRMS (ESI-TOF): m/z 345.055126 [M+ + H], C16H10F5O3 requires 345.055013. 1-Phenyl-2-oxopropyl 4-benzoylbenzoate 9e. Yellow oil; 1H NMR (300.1 MHz, CDCl3): δ 2.23 (s, 3H), 6.26 (s, 1H), 7.46–7.66 (m, 8H), 7.81–7.88 (m, 4H), 8.24 (d, J = 8.3 Hz, 2H) ppm; 13C{1H} NMR (75.47 MHz, CDCl3): δ 26.2, 81.6, 128.1, 128.5, 129.2, 129.6, 129.8, 130.1, 132.3, 133.0, 133.1, 136.9, 141.8, 165.1, 195.9, 201.2 ppm; IR (neat): ν 1667, 1717 and 1738 (C
O) cm−1; GC-MS (EI, 70eV): m/z 315 (10%, M+− MeCO), 252 (5), 209 (100), 152 (10), 105 (20); HRMS (ESI-TOF): m/z 359.128577 [M+ + H], C23H19O4 requires 359.128334. 1-Phenyl-2-oxopropyl 2-chloro-5-sulfamoylbenzoate 9f. Yellow solid; 1H NMR (300.1 MHz, CDCl3): δ 2.16 (s, 3H), 5.44 (br, 2H), 6.22 (s, 1H), 7.43–7.59 (m, 6H), 8.16 (dd, J = 8.3 and 2.1 Hz, 1H), 8.73 (d, J = 2.1 Hz, 1H) ppm; 13C{1H} NMR (75.47 MHz, CDCl3): δ 26.6, 82.4, 128.6, 129.1, 129.7, 130.2, 131.3, 132.4, 132.9, 134.9, 137.0, 140.5, 164.1, 201.4 ppm; IR (nujol): ν 1714 (br, C
O), 3390 (N–H) cm−1; GC-MS (EI, 70eV): m/z 324 (15%, M+− MeCO), 218 (100), 138 (10), 105 (15), 77 (20); Elemental analysis calcd (%) for C16H14O5ClNS: C 52.25, H 3.84, N 3.81; found: C 52.33, H 3.96, N 3.70. 1-Phenyl-2-oxopropyl 4-(4-chlorophenoxy)benzoate 9g. Yellow oil; 1H NMR (300.1 MHz, CDCl3): δ 2.21 (s, 3H), 6.22 (s, 1H), 7.01 (m, 4H), 7.34–7.55 (m, 7H), 8.11 (m, 2H) ppm; 13C{1H} NMR (75.47 MHz, CDCl3): δ 26.1, 81.3, 117.5, 121.3, 124.0, 128.0, 129.1, 129.4, 130.1, 132.2, 133.4, 154.2, 161.8, 165.2, 201.8 ppm; IR (neat): ν 1715 (br, C
O) cm−1; GC-MS (EI, 70eV): m/z 337 (5%, M+− MeCO), 231 (100), 168 (15), 139 (10), 105 (10); HRMS (ESI-TOF): m/z 381.089428 [M+ + H], C22H18O4Cl requires 381.089363. 1-Phenyl-2-oxopropyl tetrahydrofuran-2-carboxylate 9h. Orange oil; 1H NMR (300.1 MHz, CDCl3): δ 1.89–2.04 (m, 2H), 2.15 (s, 3H), 2.26–2.40 (m, 2H), 3.92–4.11 (m, 2H), 4.59–4.67 (m, 1H), 6.03 (s, 1H), 7.42 (br, 5H) ppm; 13C{1H} NMR (75.47 MHz, CDCl3): δ 25.1, 26.2, 30.3, 69.5, 76.3, 80.9, 128.2, 129.1, 129.4, 132.9, 172.8, 201.1 ppm; IR (neat): ν 1731 and 1755 (C
O) cm−1; GC-MS (EI, 70eV): m/z 205 (5%, M+− MeCO), 177 (10), 105 (10), 71 (100), 43 (50); HRMS (ESI-TOF): m/z 249.112745 [M+ + H], C14H17O4 requires 249.112692. 1-Phenyl-2-oxopropyl 1H-pyrrole-2-carboxylate 9i. Brown oil; 1H NMR (300.1 MHz, CDCl3): δ 2.20 (s, 3H), 6.16 (s, 1H), 6.31 (dd, J = 6.5 and 2.8 Hz, 1H), 7.00–7.09 (m, 2H), 7.42–7.53 (m, 5H), 9.24 (br, 1H) ppm; 13C{1H} NMR (75.47 MHz, CDCl3): δ 26.0, 80.6, 110.7, 116.5, 123.7, 127.9, 128.1, 128.7, 129.3, 133.5, 160.0, 202.3 ppm; IR (nujol): ν 1732 (br, C
O), 3315 (NH) cm−1; GC-MS (EI, 70eV): m/z 200 (20%, M+− MeCO), 94 (100), 66 (20), 39 (20); HRMS (ESI-TOF): m/z 244.097459 [M+ + H], C14H14O3N requires 244.097378. 1-Phenyl-2-oxopropyl thiophene-2-carboxylate 9j. Red oil; 1H NMR (300.1 MHz, CDCl3): δ 2.21 (s, 3H), 6.18 (s, 1H), 7.34 (dd, J = 5.1 and 3.1 Hz, 1H), 7.43-7.55 (m, 5H), 7.60 (dd, J = 5.1 and 1.1 Hz, 1H), 8.24 (dd, J = 3.1 and 1.1 Hz, 1H) ppm; 13C{1H} NMR (75.47 MHz, CDCl3): δ 26.1, 81.0, 126.3, 127.9, 128.0, 129.1, 129.4, 132.6, 133.4, 133.8, 161.8, 201.8 ppm; IR (nujol): ν 1719 (br, C
O) cm−1; GC-MS (EI, 70eV): m/z 217 (20%, M+− MeCO), 111 (100), 83 (15), 39 (20); HRMS (ESI-TOF): m/z 261.05846 [M+ + H], C14H13O3S requires 261.058541. 1-Phenyl-2-oxopropyl 3-(1H-indol-3-yl)propionate 9k. Orange oil; 1H NMR (300.1 MHz, CDCl3): δ 2.09 (s, 3H), 2.28 (m, 2H), 3.17 (t, J = 7.3 Hz, 2H), 5.99 (s, 1H), 7.09-7.39 (m, 9H), 7.60 (d, J = 7.9 Hz, 1H), 7.97 (br, 1H) ppm; 13C{1H} NMR (75.47 MHz, CDCl3): δ 20.9, 26.4, 35.0, 81.3, 111.5, 115.1, 119.0, 119.7, 121.9, 122.5, 127.8, 128.4, 129.5, 129.7, 133.7, 136.7, 173.0, 202.3 ppm; IR (nujol): ν 1716 and 1738 (C
O), 3412 (NH) cm−1; GC-MS (EI, 70eV): m/z 321 (20%, M+), 188 (10), 146 (10), 130 (100), 105 (10), 77 (10); HRMS (ESI-TOF): m/z 322.144280 [M+ + H], C20H20O3N requires 322.144319. 1-Phenyl-2-oxopropyl (5-methoxy-2-methyl-1H-indol-3-yl)acetate 9l. Yellow oil; 1H NMR (300.1 MHz, CDCl3): δ 2.04 (s, 3H), 2.38 (s, 3H), 3.80 (s, 3H), 3.83 (s, 2H), 5.97 (s, 1H), 6.77 (dd, J = 8.7 and 2.5 Hz, 1H), 7.01 (d, J = 2.5 Hz, 1H), 7.13 (d, J = 8.7 Hz, 1H), 7.39 (br, 5H), 7.82 (br, 1H) ppm; 13C{1H} NMR (75.47 MHz, CDCl3): δ 11.8, 25.9, 30.1, 55.8, 81.1, 100.2, 103.9, 111.0, 111.2, 127.9, 128.9, 129.0, 129.2, 130.1, 133.3, 133.7, 154.2, 171.2, 202.0 ppm; IR (nujol): ν 1719 (br, C
O), 3391 (NH) cm−1; GC-MS (EI, 70eV): m/z 351 (30%, M+), 174 (100), 158 (15), 131 (15), 105 (10), 77 (10); HRMS (ESI-TOF): m/z 352.154709 [M+ + H], C21H22O4N requires 352.154883. 1-Phenyl-2-oxopropyl 2-oxo-2H-chromene-3-carboxylate 9m. Orange oil; 1H NMR (300.1 MHz, CDCl3): δ 2.20 (s, 3H), 6.21 (s, 1H), 7.26–7.65 (m, 9H), 8.62 (s, 1H) ppm; 13C{1H} NMR (75.47 MHz, CDCl3): δ 26.0, 81.7, 116.7, 117.0, 117.7, 124.9, 127.9, 129.1, 129.4, 129.7, 132.8, 134.7, 149.5, 155.2, 156.3, 162.0, 201.4 ppm; IR (nujol): ν 1713 (br, C
O) cm−1; GC-MS (EI, 70eV): m/z 280 (10%, M+− MeCO), 216 (10), 173 (100), 146 (20), 105 (15), 89 (20); HRMS (ESI-TOF): m/z 323.092216 [M+ + H], C19H15O5 requires 323.091949. 1-Phenyl-2-oxopropyl heptanoate 9n. Yellow oil; 1H NMR (300.1 MHz, CDCl3): δ 0.87 (t, J = 6.9 Hz, 3H), 1.25–1.38 (m, 6H), 1.66 (m, 2H), 2.11 (s, 3H), 2.41 (m, 2H), 5.97 (s, 1H), 7.37–7.42 (m, 5H) ppm; 13C{1H} NMR (75.47 MHz, CDCl3): δ 14.0, 22.4, 24.7, 26.1, 28.6, 31.3, 33.9, 80.7, 127.9, 129.0, 129.3, 133.3, 173.1, 201.8 ppm; IR (neat): ν 1734 (br, C
O) cm−1; GC-MS (EI, 70eV): m/z 219 (20%, M+− MeCO), 134 (10), 113 (100), 105 (20), 85 (15), 77 (10); HRMS (ESI-TOF): m/z 263.164689 [M+ + H], C16H23O3 requires 263.164725. 1-Phenyl-2-oxopropyl 3-cyclopentylpropionate 9o. Yellow oil; 1H NMR (300.1 MHz, CDCl3): δ 1.10–1.89 (m, 11H), 2.11 (s, 3H), 2.47 (m, 2H), 5.97 (s, 1H), 7.40 (br, 5H) ppm; 13C{1H} NMR (75.47 MHz, CDCl3): δ 24.6, 25.7, 30.5, 31.9, 32.8, 39.1, 80.3, 127.6, 128.6, 128.8, 132.8, 172.7, 201.4 ppm; IR (neat): ν 1768 (br, C
O) cm−1; GC-MS (EI, 70eV): m/z 274 (2%, M+), 231 (40), 134 (10), 125 (100), 107 (60), 97 (10), 79 (50); HRMS (ESI-TOF): m/z 275.164641 [M+ + H], C17H23O3 requires 275.164724.
Acknowledgements
This work was supported by the Ministerio de Educación y Ciencia (MEC) of Spain (Projects CTQ2006-08485/BQU and Consolider Ingenio 2010 (CSD2007-00006)) and the Gobierno del Principado de Asturias (FICYT Project IB08-036). J.F. thanks MEC and the European Social Fund for the award of a PhD grant.Notes and references
- For references on the use of esters units as protecting groups of alcohols, see:
(a) P. J. Kocienski, Protecting Groups, Thieme Verlag, Stuttgart, 3rd edn, 2003 Search PubMed;
(b) T. W. Greene, P. G. M. Wuts, Protective Groups in Organic Synthesis, Wiley-Interscience, New York, 3rd edn, 1999 Search PubMed.
- See, for example:
(a) N. Kaila, K. Janz, S. DeBernardo, P. W. Bedard, R. T. Camphausen, S. Tam, D. H. H. Tsao, J. C. Keith, C. Nickerson-Nutter, A. Shilling, R. Young-Sciame and Qin Wang, J. Med. Chem., 2007, 50, 21 CrossRef CAS;
(b) J. F. Márquez Ruiz, G. Radics, H. Windle, H. O. Serra, A. L. Simplício, K. Kedziora, P. G. Fallon, D. P. Kelleher and J. F. Gilmer, J. Med. Chem., 2009, 52, 3205 CrossRef.
- See, for example:
(a) P. Ruggli and K. Knecht, Helv. Chim. Acta, 1944, 27, 1108 CrossRef CAS;
(b) M. A. Ashraf, M. A. Jones, N. E. Kelly, A. Mullaney, J. S. Snaith and I. Williams, Tetrahedron Lett., 2003, 44, 3151 CrossRef CAS.
- See, for example: T. Shono, Y. Matsumura and Y. Nakagawa, J. Am. Chem. Soc., 1975, 97, 6144 Search PubMed.
- See, for example: T. Shinada, T. Kawakami, H. Sakai, I. Takada and Y. Ohfune, Tetrahedron Lett., 1998, 39, 3757 Search PubMed.
- See, for example:
(a) D. W. Hansen, R. Pappo and R. B. Garland, J. Org. Chem., 1988, 53, 4244 CrossRef;
(b) P. Yates, R. S. Grewal and P. C. Hayes, Can. J. Chem., 1988, 66, 2805 CrossRef CAS.
- See, for example:
(a) G. W. K. Cavill and D. H. Solomon, J. Chem. Soc., 1955, 4426 RSC;
(b) A. S. Demir, M. Camkerten, H. Akgun, C. Tanyeli, A. S. Mahasneh and D. S. Watt, Synth. Commun., 1990, 20, 2279 CAS.
-
(a) Ruthenium complexes have emerged in recent years as powerful tools in catalytic organic synthesis, with significant applications in atom-economic transformations. See, for example: Ruthenium Catalysts and Fine Chemistry, ed. C. Bruneau and P. H. Dixneuf, Springer-Verlag, Berlin, 2004 Search PubMed;
(b) Ruthenium in Organic Synthesis, ed. S.-I. Murahashi, Wiley-VCH, Weinheim, 2004 Search PubMed;
(c) B. M. Trost, M. U. Fredericksen and M. T. Rudd, Angew. Chem., Int. Ed., 2005, 44, 6630 CrossRef CAS;
(d) C. Bruneau and P. H. Dixneuf, Angew. Chem., Int. Ed., 2006, 45, 2176 CrossRef CAS.
-
(a) D. Devanne, C. Ruppin and P. H. Dixneuf, J. Org. Chem., 1988, 53, 925 CrossRef CAS;
(b) S. Costin, N. P. Rath and E. B. Bauer, Adv. Synth. Catal., 2008, 350, 2414 CrossRef CAS.
-
(a) C. Bruneau, Z. Kabouche, M. Neveux, B. Seiller and P. H. Dixneuf, Inorg. Chim. Acta, 1994, 222, 155 CrossRef CAS;
(b) C. Darcel, C. Bruneau, P. H. Dixneuf and G. Neef, J. Chem. Soc., Chem. Commun., 1994, 333 RSC.
- T. Mitsudo, Y. Hori, Y. Yamakawa and Y. Watanabe, J. Org. Chem., 1987, 52, 2230 CrossRef CAS.
- For general reviews on metal-catalyzed addition of heteroatom-hydrogen bonds to alkynes, see:
(a) F. Alonso, I. P. Beletskaya and M. Yus, Chem. Rev., 2004, 104, 3079 CrossRef CAS;
(b) M. Beller, J. Seayad, A. Tillack and H. Jiao, Angew. Chem., Int. Ed., 2004, 43, 3368 CrossRef CAS.
- For specific accounts covering the use ruthenium catalysts in this type of transformations, see:
(a) C. Bruneau, M. Neveux, Z. Kabouche, C. Ruppin and P. H. Dixneuf, Synlett, 1991, 755 CrossRef;
(b) C. Fischmeister, C. Bruneau, and P. H. Dixneuf, in Ruthenium in Organic Synthesis, ed. S.-I. Murahashi, Wiley-VCH, Weinheim, 2004, pp.189-217 Search PubMed.
- See, for example:
(a) P. T. Anastas, and J. C. Warner, in Green Chemistry: Theory and Practice, Oxford University Press, Oxford, 1998 Search PubMed;
(b) A. S. Matlack, in Introduction to Green Chemistry, Marcel Dekker, New York, 2001 Search PubMed;
(c) M. Lancaster, in Handbook of Green Chemistry and Technology, eds. J. H. Clark, and D. J. Macquarrie, Blackwell Publishing, Abingdon, 2002 Search PubMed;
(d) M. Lancaster, in Green Chemistry: An Introductory Text, RSC Editions, London, 2002 Search PubMed.
- See, for example:
(a) W. M. Nelson, in Green Solvents for Chemistry: Perspectives and Practice, Oxford University Press, New York, 2003 Search PubMed;
(b) J. H. Clark and S. J. Taverner, Org. Process Res. Dev., 2007, 11, 149 Search PubMed;
(c) F. M. Kerton, in Alternative Solvents for Green Chemistry, RSC Publishing, Cambridge, 2009 Search PubMed.
- See, for example:
(a) C. J. Li, Chem. Rev., 1993, 93, 2023 CrossRef CAS;
(b) A. Lubineau, J. Auge and Y. Queneau, Synthesis, 1994, 741 CrossRef CAS;
(c) C. J. Li and T. H. Chan, in Organic Reactions in Aqueous Media, John Wiley & Sons, New York, 1997 Search PubMed;
(d) U. M. Lindström, Chem. Rev., 2002, 102, 2751 CrossRef;
(e) C. J. Li, Acc. Chem. Res., 2002, 35, 533 CrossRef CAS;
(f) C. J. Li, Chem. Rev., 2005, 105, 3095 CrossRef CAS;
(g) C. K. Z. Andrade and L. M. Alves, Curr. Org. Chem., 2005, 9, 195 CrossRef CAS;
(h) C. J. Li and L. Chen, Chem. Soc. Rev., 2006, 35, 68 RSC;
(i) L. Chen and C. J. Li, Adv. Synth. Catal., 2006, 348, 1459 CrossRef CAS;
(j) C. I. Herrerías, X. Yao, Z. Li and C. J. Li, Chem. Rev., 2007, 107, 2546 CrossRef CAS;
(k) C. J. Li and T. H. Chan, in Comprehensive Organic Reactions in Aqueous Media, John Wiley & Sons, New Jersey, 2007 Search PubMed;
(l) Organic Reactions in Water: Principles, Strategies and Applications, ed. U. M. Lindstrom, Blackwell Publishing Ltd., Oxford, 2007 Search PubMed.
- See, for example:
(a) P. Kalck and F. Monteil, Adv. Organomet. Chem., 1992, 34, 219 CAS;
(b) W. A. Herrmann and C. W. Kohlpaintner, Angew. Chem., Int. Ed. Engl., 1993, 32, 1524 CrossRef;
(c) Aqueous Organometallic Chemistry and Catalysis, eds. I. T. Horváth and F. Joó, Kluver, Dodrecht, 1995 Search PubMed;
(d) F. Joó and A. Kathó, J. Mol. Catal. A: Chem., 1997, 116, 3 CrossRef;
(e) Aqueous-Phase Organometallic Catalysis: Concepts and Applications, eds. B. Cornils and W. A. Herrmann, Wiley-VCH, Weinheim, 1998 Search PubMed;
(f) B. E. Hanson, Coord. Chem. Rev., 1999, 185–186, 795 CrossRef CAS;
(g) F. Joó, in Aqueous Organometallic Catalysis, Kluver, Dodrecht, 2001 Search PubMed;
(h) N. Pinault and D. W. Bruce, Coord. Chem. Rev., 2003, 241, 1 CrossRef CAS;
(i) C. A. M. Afonso, L. C. Branco, N. R. Candeias, P. M. P. Gois, N. M. T. Lourenço, N. M. M. Mateus and J. N. Rosa, Chem. Commun., 2007, 2669 RSC.
-
(a) V. Cadierno, S. E. García-Garrido and J. Gimeno, Chem. Commun., 2004, 232 RSC;
(b) V. Cadierno, P. Crochet, S. E. García-Garrido and J. Gimeno, Dalton Trans., 2004, 3635 RSC;
(c) V. Cadierno, S. E. García-Garrido, J. Gimeno and N. Nebra, Chem. Commun., 2005, 4086 RSC;
(d) V. Cadierno, S. E. García-Garrido, J. Gimeno, A. Varela-Álvarez and J. A. Sordo, J. Am. Chem. Soc., 2006, 128, 1360 CrossRef CAS;
(e) P. Crochet, J. Díez, M. A. Fernández-Zúmel and J. Gimeno, Adv. Synth. Catal., 2006, 348, 93 CrossRef CAS;
(f) A. E. Díaz-Álvarez, P. Crochet, M. Zablocka, C. Duhayon, V. Cadierno, J. Gimeno and J. P. Majoral, Adv. Synth. Catal., 2006, 348, 1671 CrossRef CAS;
(g) V. Cadierno, S. E. García-Garrido and J. Gimeno, J. Am. Chem. Soc., 2006, 128, 15094 CrossRef CAS;
(h) V. Cadierno, J. Francos, J. Gimeno and N. Nebra, Chem. Commun., 2007, 2536 RSC;
(i) V. Cadierno, J. Gimeno and N. Nebra, Chem.–Eur. J., 2007, 13, 6590 CrossRef CAS;
(j) A. E. Díaz-Álvarez, P. Crochet, M. Zablocka, C. Duhayon, V. Cadierno and J.-P. Majoral, Eur. J. Inorg. Chem., 2008, 786 CrossRef CAS;
(k) V. Cadierno, P. Crochet and J. Gimeno, Synlett, 2008, 1105 CAS.
- The abbreviations used for the hydrosoluble phosphines correspond to the following IUPAC names: PTA = 1,3,5-triaza-7-phosphatricyclo[3.3.1.13,7]decane; PTA-Bn = 1-benzyl-3,5-diaza-1-azonia-7-phosphatricyclo[3.3.1.13,7]decane chloride; DAPTA = 3,7-diacetyl-1,3,7-triaza-5-phosphabicyclo[3.3.1]nonane; TPPMS = 3-diphenylphosphanyl-benzenesulfonate sodium salt.
- V. Cadierno, J. Francos and J. Gimeno, Chem.–Eur. J., 2008, 14, 6601 CrossRef CAS.
- As observed in our previous work on nitrile hydrations using catalysts 3a–6d, no direct relationship between the solubility of these complexes in water and their catalytic activity exists. Detailed data about the solubility of 3a–6d in water at 20 °C can be found in ref. 20.
- For general reviews on the chemistry of transition-metal allenylidene complexes, see:
(a) M. I. Bruce, Chem. Rev., 1998, 98, 2797 CrossRef CAS;
(b) V. Cadierno, M. P. Gamasa and J. Gimeno, Eur. J. Inorg. Chem., 2001, 571 CrossRef CAS;
(c) V. Cadierno, P. Crochet and J. Gimeno, in Metal vinylidenes and allenylidenes in catalysis: From reactivity to applications in synthesis, ed. C. Bruneau and P. H. Dixneuf, Wiley-VCH, Weinheim, 2008, pp 61-98 Search PubMed;
(d) V. Cadierno and J. Gimeno, Chem. Rev., 2009, 109, 3512 CrossRef CAS.
- For specific examples of Ru-allenylidene Cα=Cβ bond cleavage by water, with alkene release, see:
(a) C. Bianchini, M. Peruzzini, F. Zanobini, C. López, I. de los Rios and A. Romerosa, Chem. Commun., 1999, 443 RSC;
(b) E. Bustelo and P. H. Dixneuf, Adv. Synth. Catal., 2005, 347, 393 CrossRef.
- Ruthenium-catalyzed cleavage of the C
C bond of propargylic alcohols to generate the corresponding alkenes and carbon monoxide has been described by R.-S. Liu, and co-workers:
(a) S. Datta, C.-L. Chang, K.-L. Yeh and R.-S. Liu, J. Am. Chem. Soc., 2003, 125, 9294 CrossRef CAS;
(b) R.-S. Liu, Synlett, 2008, 801 CrossRef CAS;
(c) For a general review on catalytic reactions involving carbon-carbon triple bond cleavage, see: M. Tobisu and N. Chatani, Chem. Soc. Rev., 2008, 37, 300 Search PubMed. - Addition of carboxylic acids to ethisterone and mestranol could be successfully achieved in organic media using [{Ru(μ-O2CH)(CO)2(PPh3)}2] as catalyst: see ref. 10b.
-
(a) A. Segaloff and R. B. Gabbard, Steroids, 1964, 4, 433 CrossRef CAS;
(b) V. I. De Brabandere, L. M. Thienpont, D. Stöckl and A. P. De Leenheer, J. Lipid Res., 1997, 38, 780 CAS.
-
(a) R. Kirdani, R. I. Dorfman and W. R. Nes, Steroids, 1963, 1, 219 CrossRef CAS;
(b) R. Kanojia, S. Rovinsky and I. Scheer, Chem. Commun., 1971, 1581 Search PubMed.
- Formation of allenylidene-ruthenium(II) complexes from ethisterone and mestranol has been described: V. Cadierno, S. Conejero, M. P. Gamasa, J. Gimeno and M. A. Rodríguez, Organometallics, 2002, 21, 203 Search PubMed.
-
(a) M. M. Midland, J. Org. Chem., 1975, 40, 2250 CrossRef CAS;
(b) The secondary propargylic alcohol 1-pentafluorophenyl-2-propyn-1-ol 1g, isolated as a yellow oil in 78% yield, has not been previously described. Characterization data for this new compound are as follows: 1H NMR (300.1 MHz, CDCl3):δ 2.66 (d, J = 2.4 Hz, 1H), 3.75 (br, 1H), 5.76 (d, J = 2.4 Hz, 1H) ppm; 13C{1H} NMR (75.47 MHz, CDCl3): δ 54.3, 74.8, 80.1, 114.1 (m), 135.7 (m), 139.6 (m), 143.3 (m) ppm; 19F NMR (282.4 MHz, CDCl3): δ−161.7 (m, 2F), −153.8 (m, 1F), −143.6 (m, 2F) ppm; IR (neat): ν 2127 (C
C), 3309 (br, OH and ≡C–H) cm−1; GC-MS (EI, 70eV): m/z 222 (30%, M+), 203 (70) 194 (100), 174 (50), 143 (20), 117 (20), 99 (15), 53 (20). -
(a) C. S. Allardyce, P. J. Dyson, D. J. Ellis and S. L. Heath, Chem. Commun., 2001, 1396 RSC;
(b) H. Horváth, G. Laurenczy and A. Kathó, J. Organomet. Chem., 2004, 689, 1036 CrossRef CAS;
(c) C. Scolaro, A. Bergamo, L. Brescacin, R. Delfino, M. Cocchietto, G. Laurenczy, T. J. Geldbach, G. Sava and P. J. Dyson, J. Med. Chem., 2005, 48, 4161 CrossRef CAS;
(d) J. Díez, M. P. Gamasa, E. Lastra, A. García-Fernández and M. P. Tarazona, Eur. J. Inorg. Chem., 2006, 2855 CrossRef CAS.
- C. L. Stevens, W. Malik and R. Pratt, J. Am. Chem. Soc., 1950, 72, 4758 CrossRef CAS.
- M. B. Rubin and S. Inbar, J. Org. Chem., 1988, 53, 3355 CrossRef CAS.
|
This journal is © The Royal Society of Chemistry 2010 |
Click here to see how this site uses Cookies. View our privacy policy here.