DOI:
10.1039/B913398D
(Paper)
Green Chem., 2010,
12, 94-98
Catalyst-free silylation of alcohols and phenols by promoting HMDS in CH3NO2 as solvent†
Received 8th July 2009, Accepted 17th September 2009
First published on 26th October 2009
Abstract
An uncatalyzed method for the silylation of alcohols and phenols with HMDS in CH3NO2 at rt is developed. A diverse range of aromatic and aliphatic alcohols as well as phenols undergo the silylation in very short reaction time with excellent yield. The uncatalyzed reaction requires neither elevated temperature nor high pressure for the silylation.
Introduction
Functional group protection is at the heart of multifunctional and multistep target molecules. Alcohol and phenol moieties represent one of the most ubiquitous functional groups in nature.1 The formation of a silyl ether from a hydroxyl group is one of the most popular and widely used methods. It is frequently used as a protection method in multistep reactions due to its stability under a variety of conditions, solubility in non-polar solvents and its ease of removal. Silylation of hydroxyl groups is also utilized in analytical chemistry to prepare volatile derivatives of alcohols and phenols for GC and GC-MS analysis.2 Many silylating agents, such as chlorotrimethylsilane,3 hexamethyldisiloxane,4 and allylsilane5 have been used to introduce silyl groups into a variety of alcohols. Hexamethyldisilazane (HMDS) is a stable, commercially available reagent which gives ammonia as the only by-product. Even though the handling of this reagent is convenient, the main drawback is its poor silylating power which demands the use of catalysts and high temperature.6 A variety of catalysts have been reported for the activation of HMDS, such as sulfonic acid-functionalized nanoporus silica,7 trichloroisocyanuric acid (TCCA),8 InBr3,9 zirconyl triflate,10 K-10 montmorillonite,11 H-β zeolite,12 silica-supported perchloric acid,13 barbutric acid,14 iodine,15 MgBr2,16N-bromosuccinimide (NBS)17 and iron(III) trifluoroacetate.18Hence, considering the importance of the silylation reaction, continuous efforts have been made to develop new methodologies involving the application of various catalysts. However, various limitations exist with the reported methodologies, such as long reaction time,14 high temperature,12 halogenated solvents,15 costly catalysts,9 toxic metal catalysts10 and use of excess reagent.25 According to the principle of green chemistry, the synthetic method should be designed to minimize the energy input by running the reaction at ambient temperature and reducing the amount of reagents or catalyst.19 Thus, with increasing environmental concern, there is a demand for efficient, metal- or catalyst-free and environmentally benign methods for the silylation of alcohols.
The appropriation of several solvents without intervention of catalyst has been reported for various organic transformations. Watahiki et al. have reported DMSO for cyanobenzoylation of aldehydes with benzyl cyanide.20 The combination of DMSO with hexane was employed for the cyanosilylation of aldehyde21 as well as synthesis of silyl ether from alcohol and tert-butyldimethylsilyl chloride.22 Similarly, Iwanami et al. describe the synthesis of cyanohydrin carbonates in DMSO in the presence of molecular sieves.23 Kumamoto et al. show the cyanation of acetal with TMSCN in CH3NO2 at 60 °C and high pressure.24 Considering the activity of different silyl reagents in polar solvents, HMDS could be activated in the proper solvent without using catalyst.
Result and discussion
We herein report a novel and simple catalyst-free silylation method of alcohols and phenols under mild conditions (Scheme 1). The effect of various solvents for the silylation of benzyl alcohol is explored (Table 1). The polar aprotic solvents DMF and DMSO produce 20 and 48% of yield, respectively (entries 1 and 2). THF shows a lesser yield (10%) of silylated product (entry 4). Methanol, CH2Cl2, dioxane, CH3CN and hexane give no product (entries 3 and 5–8). Surprisingly, the reaction in CH3NO2 results in the desired product with excellent yield in a very short reaction time (10 min). Accordingly, 1 mL of CH3NO2 and 1 mmol of HMDS is sufficient for the completion of silylation reaction with alcohols at rt. This could be the first example of uncatalyzed silylation of alcohols with HMDS in CH3NO2. |
| Scheme 1 Silylation of alcohols with HMDS in CH3NO2. | |
A variety of aromatic and aliphatic alcohols have been examined for the silylation in CH3NO2 without intervention of any catalyst or promoter (Table 2). Benzyl alcohol and most of its various derivatives undergo the silylation with excellent yields within 10 min of reaction time (entries 1–8). Only m-nitrobenzyl alcohol reacts rather slowly (30 min) with reduced yield (80%) (entry 8). This could be due to the electron-withdrawing power of the nitro substituent. Anilinoethanol gives the o-silylation product with excellent yield (entry 9). This method is also applicable to trans-2-methyl-3-phenyl-2-propen-l-ol with relatively lower yield (86%) and longer reaction time (30 min) (entry 10). Methoxyphenylethanol results in the silylation product with very good yield (entry 11). Silylation of heterocyclic furfuryl alcohol (entry 12) and aliphatic primary lauryl alcohol (entry 13) proceed efficiently with good yield in relatively longer reaction time (30 min). Acid-sensitive geraniol is able to produce the silylation product with considerably good yield (entry 14). This uncatalyzed protocol also tolerates presence of acetyl ester, benzoyl ester and TBDMS ether in order to produce excellent yields (entries 15–17).
Entry | Products | Yield/%b |
---|
Reagents and conditions: alcohols (1 mmol), HMDS (1.0 mmol), CH3NO2 (1 mL) at rt with 10 min reaction time, unless otherwise mentioned. Isolated yield. 30 min reaction time. |
---|
1 | 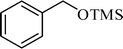 | 99 |
2 | 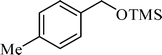 | 98 |
3 | 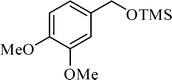 | 99 |
4 | 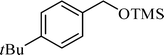 | 94 |
5 | 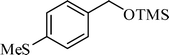 | 97 |
6 | 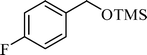 | 96 |
7 | 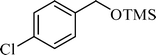 | 95 |
8 | 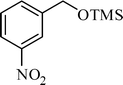 | 80c |
9 | 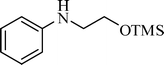 | 98 |
10 | 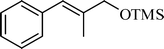 | 86c |
11 | 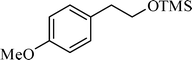 | 94 |
12 | 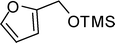 | 87c |
13 |  | 79c |
14 | 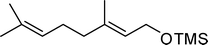 | 90 |
15 | 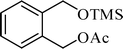 | 99 |
16 | 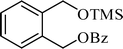 | 99 |
17 | 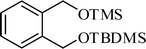 | 99 |
The silylation reaction is extended to various secondary alcohols and phenols (Table 3). Benzhydrol and 4-4′-dichlorobenzhydrol react smoothly with HMDS, giving excellent yields (entries 1 and 2). Phenylethanol and p-tolylethanol deliver the corresponding silylated products with 84 and 99% yield, respectively (entries 3 and 4). p-Bromomethylbenzyl alcohol requires a slightly longer reaction time for the production of silylated product (entry 5). 1-Phenyl-1-propanol requires 10 min for the completion of reaction (entry 6).
Entry | Products | Time | Yield/%b |
---|
Reagents and conditions: alcohols or phenols (1 mmol), HMDS (1.0 mmol), CH3NO2 (1 mL) at rt. Isolated yield. |
---|
1 | 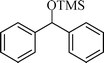 | 10 min | 99 |
2 | 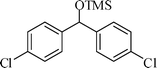 | 10 min | 99 |
3 |  | 30 min | 84 |
4 | 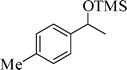 | 10 min | 99 |
5 | 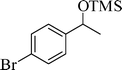 | 30 min | 91 |
6 |  | 10 min | 93 |
7 | 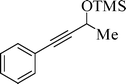 | 30 min | 87 |
8 | 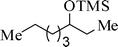 | 30 min | 89 |
9 | 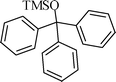 | 6 h | NR |
10 |  | 30 min | 80 |
11 |  | 15 min | 95 |
12 | 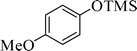 | 15 min | 84 |
13 | 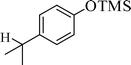 | 15 min | 92 |
14 | 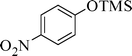 | 6 h | NR |
15 | 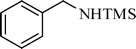 | 6 h | NR |
Propargyl alcohol and secondary aliphatic alcohol undergo the silylation reaction smoothly with slightly lower yield within 30 min reaction time (entries 7 and 8). The present method is not applicable to tertiary alcohol, even after 6 h reaction time (entry 9). This may indicate that the steric effect is serious enough to prevent the reaction taking place. Interestingly, phenols also undergo the silylation to produce silyl ether in considerably high yields (entries 10–13). Unsubstituted phenol requires a longer reaction time (30 min) relative to electron-donating p- and o-methoxy and p-isopropyl phenol (15 min) (entries 11–13). However, p-nitro phenol fails to produce silylation product (entry 14). This result may indicate that the electron-withdrawing ability of the nitro group could reduce the electron density on the oxygen atom that prohibits the silylation. It is very interesting to note that m-nitrobenzyl alcohol (entry 8, Table 2) gives a pretty good yield, because no such conjugation could occur here. The reaction of benzyl amine did not produce the corresponding silyl ether, even after 6 h reaction time (entry 15). This may be due to the high affinity of silicon for oxygen atoms compared to the nitrogen of benzyl amine.30 These data demonstrate that HMDS does not interact with amines under the present conditions.
A possible mechanism and the role of CH3NO2 are shown in Scheme 2. Several reports have indicated that the oxygen atom of heteroatom oxide can activate the silylating reagent through coordination with the silicon atom.24 Alcohols react with the activated HMDS–CH3NO2 (I) complex to produce the O-silyl ether with the liberation of ammonia. The evolution of ammonia is confirmed by its strong and pungent odor and red litmus paper, which turns from red to blue. The cyanation of acetal in CH3NO2 as solvent demands high pressure and elevated temperature,24 but the present silylation reaction involves quite mild reaction conditions.
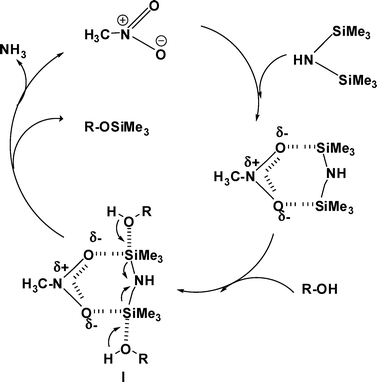 |
| Scheme 2 Plausible mechanism for the silylation of alcohols with HMDS in CH3NO2. | |
Conclusion
A novel and efficient uncatalyzed method for the silylation of alcohols and phenols is developed. Alcohols (phenols) and HMDS react to give silylated products in CH3NO2 as solvent without the aid of a catalyst. The major advantages of the method are mild reaction conditions, easy work up and the absence of catalyst. This could be the most economically convenient method for the silylation of alcohol, compared to other reported catalytic methods. Under the non-metallic and catalyst-free conditions, the reaction becomes “environmentally friendly”.Experimental section
General
In all cases, the 1H NMR (400/200 MHz) spectra were recorded with a Varian Gemini 2000 spectrometer. Chemical shifts are reported in ppm in CDCl3 with tetramethylsilane as an internal standard. 13C NMR data were collected on a Varian Gemini 2000 spectrometer (50 MHz). GC-MS data were recorded with 1200 L Single Quadrupole GC/MS System with 3800GC/Varian.General procedure for the silylation of alcohols or phenols
To a mixture of alcohol (phenol) (1 mmol) and CH3NO2 (1 mL), HMDS (1 mmol) was added at rt. The completion of the reaction was monitored with TLC. After the completion of the reaction, the reaction mixture was concentrated in vacuo. The viscous mass was subjected to silica gel flash column chromatography to obtain the pure compound.1H, 13C NMR and GC-MS data for new products are given below (Table 2, entries 9, 15, 16, 17 and Table 3, entries 7 and 8). 1H, 13C NMR and HRMS data for known products are the same as literature values.10,13,26-29
N-(2-Trimethylsilyloxy)ethyl)aniline (Table 2, entry 9). δH (200 MHz; CDCl3; Me4Si): 0.00 (s, 9H), 3.09 (t, J = 7.6 Hz, 2H), 3.64 (t, J = 7.6 Hz, 2H), 3.88 (s, 1H), 6.48–6.61 (m, 3H), 7.00–7.08 (m, 2H). δC (50 MHz; CDCl3, Me4Si): 0.5, 45.9, 61.0, 113.2, 117.5, 129.2, 148.3. GCMS: m/z = 209 [M+]. 2-((Trimethylsilyloxy)methyl)benzyl acetate (Table 2, entry 15). δH (400 MHz; CDCl3; Me4Si): 0.15 (s, 9H), 2.09 (s, 3H), 4.75 (s, 2H), 5.18 (s, 2H), 7.25–7.37 (m, 3H), 7.4 (d, J = 6.8 Hz, 1H). δC (CDCl3, 400 MHz): −0.4, 20.9, 62.3, 63.8, 127.5, 127.7, 128.47, 129.2, 133.3, 139.2. GCMS: m/z = 252 [M+]. 2-((Trimethylsilyloxy)methyl)benzyl benzoate (Table 2, entry 16). δH (400 MHz; CDCl3; Me4Si): 0.13 (s, 9H), 4.83 (s, 2H), 5.45 (s, 2H), 7.30–7.35 (m, 5 H), 7.43–7.56 (m, 2H), 8.06–8.08 (m, 2H); δC (50 MHz; CDCl3, Me4Si): −0.41, 62.49, 64.35, 127.57, 128.43, 128.53, 129.17, 129.71, 130.15, 133.07, 133.44, 139.33, 166.33. GCMS: m/z = 314 [M+]. tert-Butyldimethyl(2((trimethylsilyloxy)methyl)benzyloxy)silane (Table 2, entry 17). δH (400 MHz; CDCl3; Me4Si): 0.13 (s, 9H), 0.92 (s, 9H), 4.70 (s, 2H), 4.75 (s, 2H), 7.22–7.24 (m, 2H), 7.36–7.42 (m, 2H); δC (50 MHz; CDCl3, Me4Si): −5.25, −0.41, 18.40, 25.98, 62.35, 62.77, 126.64, 126.91, 127.03, 127.20, 137.78, 138.46. GCMS: m/z = 324 [M+]. Trimethyl(4-phenylbut-3-yn-2-yloxy)silane (Table 3. entry 7). δH (200 MHz; CDCl3; Me4Si): 0.02 (s, 3H), 1.59 (d, J = 8.2 Hz, 3H), 4.84 (q, J = 6.4 Hz, 1H), 7.33–7.45 (m, 3H), 7.48–7.53 (m, 2H). δC (50 MHz; CDCl3, Me4Si): 0.1, 25.4, 59.1, 83.6, 91.5, 123.0, 128.8, 128.3, 128.6, 131.7, 131.7. GCMS: m/z = 218 [M+]. (Hexan-3-yloxy)trimethylsilane (Table 3, entry 8). δH (400 MHz; CDCl3; Me4Si): 0.14 (s, 9H), 0.79–0.94 (m, 6 H), 1.25–1.51 (m, 8H), 3.52 (qt, J = 12 Hz, 1H), δC (50 MHz; CDCl3, Me4Si): 0.34, 9.93, 13.99, 22.63, 25.35, 30.02, 31.99, 36.89, 73.88. GCMS: m/z = 174 [M+].
Acknowledgements
The authors thank the Centre for Biological Modulators of the KRICT for financial support. Thanks are also due to BK 21 administrated by the Korea Research Council.References
-
(a) T. W. Green and P. G. M. Wuts, in protective Groups in Organic Syntheis, 3rd edn, John Wiley & Sons, NewYork, 1999, p150 Search PubMed;
(b) S. Otera, Chem. Rev., 1993, 93, 1449 CrossRef CAS;
(c) S. A. Fraklin, J. Chem. Soc., Perkin Trans. 1, 1998, 2451 RSC.
- G. V. Look, G. Smchen and J. Heberle, Silylation agents, Fluka, Buchs, Switzerland, 1195 Search PubMed.
- B. P. Bandgar, S. N. Chavare and S. S. Pandit, J. Chin. Chem. Soc., 2005, 52, 125 CAS.
- B. E. Cooper, Chem. Ind., 1978, 107, 794 Search PubMed.
- T. Morita, Y. Okamoto and H. Sakurai, Tetrahedron Lett., 1980, 21, 835 CrossRef CAS.
- C. A. Bruynes and T. K. Jurriens, J. Org. Chem., 1982, 47, 3966 CrossRef CAS.
- D. Zareyeea and B. Karimi, Tetrahedron Lett., 2007, 48, 1277 CrossRef.
- A. Khazaei, M. A. Zolfigol, A. Rostami and A. G. Choghamarani, Catal. Commun., 2007, 8, 543 CrossRef CAS.
- J. S. Yadav, B. V. S. Reddy, A. K. Basak, G. Baishya and A. V. Narsaiah, Synthesis, 2006, 3831 CrossRef CAS.
- M. Moghadam, S. Tangestaninejad, V. Mirkhani, I. Mohammadpoor-Baltork, S. Chahardahcheric and Z. Tavakoli, J. Organomet. Chem., 2008, 693, 2041 CrossRef CAS.
- Z. H. Zang, T. S. Li, F. Yang and C. G. Fu, Synth. Commun., 1998, 28, 3105 CAS.
- V. H. Tillu, V. H. Jadhav, H. B. Borate and R. D. Wakharkar, Arkivoc, 2004, 83 CAS.
- H. R. Shaterian, F. Shahrekipoor and M. Ghashang, J. Mol. Catal. A: Chem., 2007, 272, 142 CrossRef CAS and references cited therein.
- K. Khazaei, M. A. Zolfigol, Z. Tanbakouchian, M. Shiri, K. Niknam and J. Saien, Catal. Commun., 2007, 8, 917 CrossRef.
- B. Karimi and B. Golshani, J. Org. Chem., 2000, 65, 7228 CrossRef CAS.
- M. M. Mojtahedi, H. Abbasi and M. S. Abaee, J. Mol. Catal. A: Chem., 2006, 250, 6 CrossRef CAS.
- H. R. Shaterian, R. Doostmohammadi and M. Ghashang, Chin. J. Chem., 2008, 26, 1709 CrossRef CAS.
- H. Firouzabadi, N. Iranpoor, A. A. Jafari and M. R. Jafari, J. Organomet. Chem., 2008, 693, 2711 CrossRef CAS.
- P. Anastas and J. C. Warner, in Green Chemistry: Theory and Practice, Oxford University Press, Oxford, 1998 Search PubMed.
- T. Watahiki, S. Ohba and T. Oriyama, Org. Lett., 2003, 5, 2679 CrossRef CAS.
- F. L. Cabirol, A. E. C. Lim, U. Hanefeld, R. A. Sheldon and I. M. Lyapkalo, J. Org. Chem., 2008, 73, 2446 CrossRef CAS.
- T. Watahiki, M. Matsuzaki and T. Oriyama, Green Chem., 2003, 5, 82 RSC.
- T. Watahiki, Y. Hinakubo and T. Oriyama, Tetrahedron Lett., 2005, 46, 5881 CrossRef CAS.
- K. Kumamoto, K. Nakano, Y. Ichikawa and H. Kotsuki, Synlett, 2006, 12, 1968 and references cited therein.
- A. V. Narsaiah, J. Organomet. Chem., 2007, 692, 3614 CrossRef CAS.
- M. R. Saidi and N. Azizi, Organometallics, 2004, 23, 1457 CrossRef CAS.
- S. T. Kadam and S. S. Kim, J. Organomet. Chem., 2009, 694, 2562 CrossRef CAS.
- G. Rajagopal, Hanbin Lee and S. S. Kim, Tetrahedron, 2009, 65, 4735 CrossRef CAS.
- J. M. Midgley, S. J. Millership and B. W. Whalley, J. Chem. Soc., Perkin Trans. 1, 1976, 1384 RSC.
-
(a) H. Vorbruggen and K. krolikiewicz, Justus Liebigs Ann. Chem., 1976, 746;
(b) H. Vorbruggen, K. Krolikiewicz and U. Niedballa;, Justus Liebigs Ann. Chem., 1975, 988 CrossRef;
(c) M. J. Cook, A. R. Katritzky and P. Linda, Adv. Heterocycl. Chem., 1974, 17, 255 CAS;
(d) R. A. Singer and M. Dore, Org. Process Res. Dev., 2008, 12, 1261 Search PubMed.
Footnote |
† Electronic supplementary information (ESI) available: Experimental details and 1H and 13C NMR data. See DOI: 10.1039/b913398d |
|
This journal is © The Royal Society of Chemistry 2010 |
Click here to see how this site uses Cookies. View our privacy policy here.