DOI:
10.1039/C0FO00130A
(Paper)
Food Funct., 2010,
1, 294-300
Non-digestible fraction of cooked bean (Phaseolus vulgaris L.) cultivar Bayo Madero suppresses colonic aberrant crypt foci in azoxymethane-induced rats
Received
31st August 2010
, Accepted 25th October 2010
First published on
8th November 2010
Abstract
The non-digestible fraction (NDF) of common bean (Phaseolus vulgaris L.) cultivar Bayo Madero was evaluated for its chemopreventive effect on azoxymethane (AOM) induced aberrant crypt foci (ACF) in rats. Diets containing cooked beans (CB) or its non-digestible fraction (NDF) were fed to 72 male rats after 2 azoxymethane injections (15 mg kg−1 of body weight once a week for 2 weeks). ACF number, short chain fatty acids (SCFA) and β-glucuronidase activity were measured in colon sections from rats sacrificed 7 weeks after the last AOM injection. Food intake and weight gain of rats were unaffected by CB and NDF. CB and NDF suppressed the AOM-induced formation of ACF (0.8 and 1.5 ACF/distal zone, respectively vs. 6.6 ACF/distal zone based on methylene blue stain) and lowered β-glucuronidase activity in cecal, colonic and fecal content compared to AOM group. SCFA production was not significantly different among fecal, cecal and colonic content. These results indicate that CB and NDF from Bayo Madero provide direct chemoprotection against early stage of azoxymethane (AOM)-induced colon cancer in rats.
Introduction
In 2006, malignant tumors were the third highest cause of death in Mexico with colon cancer contributing 5 and 4.6% for men and women, respectively to this mortality.1 Several studies associate the importance of diet, particularly containing high fiber foods, with prevention and/or development of cancer.2,3 The common bean (Phaseolus vulgaris L.), an important food in the Mexican diet, is rich in several phytochemicals such as polyphenols, carbohydrates, fiber and polysaccharides with potential health benefits.4 Recently, the consumption of common beans has been associated with reduced mortality from colon cancer.5 For example, the phenolic extracts, residue and whole seed of navy beans significantly reduced development of chemically induced colon lesions in obese rats.6 The non-digestible carbohydrates such as fiber (soluble and insoluble), resistant starch and oligosaccharides can be fermented in the large intestine by colonic bacteria to produce short chain fatty acids (SCFA).7
Butyric acid, a major SCFA, plays an important role in suppressing growth in human colon cancer cells.8In vitro and in vivo studies have demonstrated that butyrate inhibits cell proliferation, induces differentiation and apoptosis, and modulates gene expression involved in colorectal carcinogenesis, with subsequent reduction in tumor development.9-11 Our previous studies12,13 showed that common bean, cultivars Negro 8025 and Bayo Madero, contained fermentable substrates with a non-digestible fraction that prevented the development of early and late stages of colon cancer by modulating genes and proteins involved in apoptosis, proliferation, cell cycle arrest and inflammation. Cultivar Negro 8025 prevented the development of early stage colon cancer in AOM-induced rats,12 whereas cultivar Bayo Madero elicited chemopreventive effect through several molecular mechanisms in human colon adenocarcinoma HT-29 cells.13 Furthermore, cooked beans of cultivar Bayo Madero showed the highest yield of polysaccharides (55%) and resistant starch (37%) than other bean types (Azufrado, Negro and Pinto) commonly consumed in Mexico based on regional preference.4 This investigation extends our previous study with cultivar Bayo Madero13 and describes the chemopreventive effect of its non-digestible fraction (NDF) on early stage of AOM-induced colon cancer in rats.
Experimental
Materials
Seeds of cultivar Bayo Madero were harvested in 2007 at the Bajio Experimental Station of the National Research Institute for Forestry, Agriculture and Livestock (INIFAP), Celaya, Guanajuato, Mexico. This cultivar was selected for its agronomic quality, such as yield and adaptation to the growing conditions in semi-arid regions. Male Sprague Dawley rats (28 days old) were obtained from Harlan, Inc. (Mexico City, Mexico). The care and use of these animals were in compliance with policies and regulations of the Institutional Animal Care and Use Committee of the University of Queretaro, Mexico.
Azoxymethane (AOM), (+)-catechin, α-amylase, protease, amyloglucosidase, tannic acid, D-(+)-raffinose, D-(+)-stachyose and D-(+)-verbascose standards were purchased from Sigma Chemical Co. (St. Louis, MO). Butyric acid was purchased from Sigma-Aldrich Canada Ltd. (Oakville, ON, Canada). Acetic, propionic, and formic acids and other chemicals were purchased from J. T. Baker (Mexico City, Mexico).
Thermal treatment
Seeds were cooked using a “traditional” cooking process according to the method of Aparicio-Fernández et al.14 Briefly, beans (50 g) were placed in a beaker with 250 mL of HPLC-grade water and boiled (90 °C) covered until they were suitable for consumption (approximately 2.5 h). Cooked seeds and broth were ground in a domestic grinder (Osterizer, Estado de Mexico, Mexico), lyophilized and stored in polyethylene bags at 4 °C until use.
Proximate composition
Nitrogen, lipid, and ash contents of ground raw and cooked bean samples were determined by procedures of the Association of Official Analytical Chemists (AOAC),15 (methods 920.87, 920.39, and 923.03, respectively). Protein content was calculated as nitrogen × 5.85.
Non-digestible fraction (NDF)
The extraction of non-digestible fraction was performed following the method of Kurtzman and Halbrook.16 Water (1.5 L) was added to cooked bean samples (300 g) and the mixture was shaken for 1 min and centrifuged (Hermle Z323K, Hermle Labortechnik GMBH, Wehingen, Germany) at 9000 g for 10 min. The pellet from the first centrifugation was dissolved in 100 mL of 10% tannic acid, adjusted to pH 4 and centrifuged again (9000 g, 10 min), and the pellet was washed three times with 100 mL acetone and centrifuged for 10 min after each washing to obtain the non-digestible fraction (NDF). NDF samples were lyophilized and stored in amber flasks at 4 °C until further analyses.
Soluble and insoluble fiber extraction and quantification
Soluble and insoluble fiber fractions were determined following the AOAC method 991.43.15 Sample (1 g of raw, cooked bean or NDF) was incubated with 0.1 mL of α-amylase in 50 mL of 0.08 M sodium phosphate buffer, pH 6.0 at 95 °C for 30 min in a water bath at 100 °C with manual stirring every 5 min. After cooling, the samples were adjusted to pH 7.5 and treated with 0.1 mL of protease (5 mg mL−1, in a water bath at 60 °C for 30 min), and finally with 0.3 mL of amyloglucosidase at pH 4.3 with stirring in a water bath at 60 °C for 30 min. The suspensions were cooled, vacuum filtered, and washed 3 times with 10 mL of distilled water. The residues corresponding to the insoluble fraction were dried and weighed. The supernatants were precipitated with 80% (v/v) ethanol at a 1
:
4 sample/ethanol ratio for 24 h at room temperature. The precipitates corresponding to the soluble fraction were filtered and the residues were washed 3 times with 10 mL of distilled water, dried and weighed.
Oligosaccharides were extracted according to Díaz-Batalla et al.,17 with some modifications. Raw, cooked bean or NDF samples (500 mg) and 10 mL of HPLC-grade water were placed in a water bath at 80 °C for 1 h, with stirring every 5 min. After cooling, the samples were filtered (45 μm, Millipore) and subjected to HPLC for quantification of oligosaccharides as described by Muzquiz et al.18 The sample (25 μL) was injected into an Agilent HPLC system model HP-1100 (Agilent Technologies, Inc., Santa Clara, CA) with a refractive index detector (IDR, 61362A) and fitted with a μBondapack/carbohydrate (3.9 mm × 30 cm) column, with acetonitrile-HPLC-grade water (80
:
20, v/v) as mobile phase at 1 mL min−1. Column and detector temperatures were maintained at 25 °C. Standard curves were determined using raffinose, stachyose and verbascose standards.
Quantification of phenolics
Total phenolic compounds and condensed tannin extractions were performed in raw and cooked beans, and NDF as described previously by Cardador-Martínez et al.19 with some modifications. Dry sample (1 g) was mixed with 10 mL methanol and the mixture was shaken for 24 h at 25 °C protected from light. After incubation, the samples were centrifuged (Hermle Z323K, 8000 g, 10 min) and the supernatants were analyzed for phenolic compounds and condensed tannins. Total phenolic compounds, expressed as mg equivalents of gallic acid per g sample (mg g−1), were determined according to the method of Nurmi et al.20 Methanolic extract (40 μL) was mixed with 460 μL of distilled water and 250 μL of 1 N Folin-Ciocalteu reagent. The mixture was vortexed for 5 min and 1250 μL of 20% sodium carbonate was added and shaken during 2 h in the dark. The total phenolic compounds were quantified by spectrophotometry (Perkin-Elmer Lambda 2S, Waltham, MA) at 760 nm, using gallic acid (up to 16 μg mL−1) as a reference standard.
Condensed tannins, expressed as mg of (+)-catechin equivalents per g sample (mg g−1), were analyzed according to the procedure of Deshpande and Cheryan21 modified for use in a microplate. Briefly, 200 μL of vanillin reagent (0.5% vanillin, 4% HCl in methanol; 1
:
1) were added to 50 μl of methanolic extract and placed in triplicate in a 96-well plate. Condensed tannins were quantified at 495 and 540 nm in a microplate reader (Thermo Electron Corporation, Multiskan Ascent®) using (+)-catechin (up to 0.8 mg mL−1) as a reference standard.
Quantification of resistant starch
The resistant starch was measured according to the gravimetric method of Saura-Calixto et al.22 in the NDF sample. The sample (0.1 g) was homogenized with 6 mL of 2 M KOH and placed in a shaker (Maxi Mix II, Thermolyne type 37600 mixer, San Francisco, CA) for 30 min at 25 °C. Acetate buffer (3 mL, 0.4 M, pH 4.75) was added and the pH adjusted to 4.75 using 2 N HCL or 0.275 M NaOH. Subsequently, 60 μl of amyloglucosidase were added and the tube placed in a shaking bath at 60 °C for 30 min. Samples were centrifuged (Hermle Z323K, 15 min, 3000 g) after incubation. The pellet was resuspended in 10 mL distilled water and centrifuged twice, freeze dried, and weighed. The fraction obtained corresponds to resistant starch.
In vitro protein digestibility
The protein digestibility was determined according to the method of Hsu et al.23 in the NDF sample. Sample (354.4 mg) containing exactly 63.8 mg of protein was dissolved in 10 ml of distilled water and adjusted to pH 8. The enzymatic mix (1.58 mg of trypsin, 3.65 mg of chymotrypsin, 0.45 mg of peptidase dissolved in 1 mL of distilled water) was added and the sample was incubated for 10 min at 37 °C. Bacterial protease was added and stirred for 10 min at 55 °C and change in pH was determined at room temperature.
Animal studies
Male Sprague Dawley rats (28 days old) were obtained from Harlan, Inc. The rats were maintained at 22–24 °C and 12 h light-dark cycle with ad libitum access to commercially prepared basal diet (2018S Harlan Tekland) and water throught the experimental period. After one week of acclimatization, the rats (n = 12) were randomly placed into six groups: (1) Control, subcutaneous injection and intragastric intubation of saline solution and basal diet; (2) cooked beans (CB), CB from cultivar Bayo Madero (5.7 g kg−1 of body weight) daily and basal diet; (3) non-digestible fraction (NDF) from cultivar Bayo Madero (2.5 g kg−1 of body weight) and basal diet; (4) azoxymethane (AOM), subcutaneous injection of AOM (15 mg kg−1 of body weight, dissolved in 1 mL of physiological saline solution) once a week for 2 weeks starting on 3rd week of supplementation and basal diet; (5) CB + AOM and basal diet; (6) NDF + AOM and basal. Both CB and NDF, dissolved in distilled water, were administered intragastrically once a day during the experimental period and the dose was selected according to the rural per capita intake of beans in the Lagunera Region of Mexico.24 All animals were sacrificed 7 weeks after the last injection (Fig. 1) and the colons were removed from caecum to the anus. The distal colon was fixed in phosphate-buffered formalin prior to histological analyses. Cecal and colonic contents (midcolon and distal colon) and feces samples were collected when the animals were sacrificed, immediately frozen and stored at −70 °C until analysis. Additionally, feces samples were collected during the experimental period and stored at −70 °C for SCFA and pH analyses and β-glucuronidase activity.
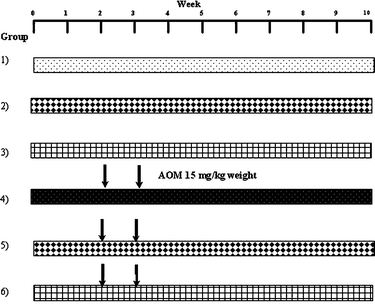 |
| Fig. 1 Experimental protocol for animal treatment. The animals were sacrificed 7 weeks after the last injection with AOM. (1) Control, basal diet; (2) CB, basal diet plus CB (5.7 g kg−1 of body weight) daily; (3) non-digestible fraction (NDF), basal diet plus NDF (2.5 g kg−1 of body weight); (4) azoxymethane (AOM), basal diet plus subcutaneous injection of AOM; (5) CB + AOM, basal diet plus cooked bean and AOM; (6) NDF + AOM, basal diet plus non-digestible fraction and AOM. | |
The consumption of food and water was registered during the experimental period and weight gain was registered once per week
SCFA analysis
Short chain fatty acids were determined by the method of Campos-Vega et al.4 Cecal, colonic or fecal samples (1 g) were suspended in 10 mL of HPLC-grade water, homogenized for about 3 min and centrifuged (Hermle Z323K, Hermle Labortechnik GMBH, Wehingen, Germany) at 8000 g for 10 min at 4 °C. An aliquot (1.5 μL) of the supernatant was adjusted to pH 2.9–3.1 by adding 1M HCl; 750 μL of the sample were transferred to a flask and 120 μL of formic acid was added. An aliquot (1.5 μL) of the acidified supernatant was loaded into the injection port of an Agilent 6890 Plus coupled to an FID detector (Agilent Technologies Wilmington, DE) and separated on a Carbowax 20 M column (25 m × 200 μm × 0.10 μm nominal). Helium was supplied as the carrier gas at 0.5 mL min−1. The initial oven temperature was 95 °C, maintained for 2 min and ramped at 20 °C min−1 to 180 °C. The temperature of the flame ionization detector (FID) and the injection port was 240 and 200 °C, respectively. The flow rates of hydrogen, air, and nitrogen as makeup gas were 30, 300 and 20 mL min−1, respectively. The concentration of acetic, propionic, and butyric acids were calculated by using standard curves of acetic, propionic, and butyric acid standards, respectively.
Aberrant crypt foci (ACF) analysis
Distal colon was removed, washed with physiological saline solution, opened longitudinally, cut in two parts (approximately 1 cm each) and fixed in 10% buffered formalin for at least 24 h. After fixation, one part of the colon was stained with 0.2% methylene blue and examined under a stereomicroscope. ACF were distinguished from adjacent normal crypts by their larger sizes, greater staining intensity, elongated pericryptal area, thickened layer of epithelial cells according to the method of Bird.25 The other part of the distal colon was dissected, embedded in paraffin, cut in parallel to the mucosal surface (5μm) and Hematoxylin and Eosin (HE) stained for histology examination. ACF was reported as the mean of distal zone.
β-Glucuronidase activity
β-Glucuronidase activity was assayed as described by Fishman et al.26 Cecal, colonic or fecal samples (5 g) kept on ice were placed in a conical tube and 10 mL 0.02M phosphate buffered saline (PBS) were added, homogenized in a polytron, then sonicated for 6 min and centrifuged (Hermle Z323K) at 500 g for 15 min at 4 °C. The supernatant (100 μL) was incubated at 37 °C for 1 h in the presence of an equal volume of β-D-glucuronide/phenolphthalein acid conjugate reagent in 800 μL of 0.02M PBS. The reaction was stopped by addition of 2.5 mL alkaline glycine, 1 mL of 5% trichloroacetic acid and 1.5 mL of HPLC-grade water and the mixture was allowed to rest for 10 min. Absorbance of liberated phenolphthalein was measured with a spectrophotometer at 540 nm and interpolated to phenolphthalein standard curve (up to 13 mg mL−1).
Statistical analysis
Results are expressed as mean ± standard error. Statistical significance was determined using Tukey's procedure (α = 0.05) for partial characterization of common bean (Phaseolus vulgaris L.) and Dunnett test (α = 0.05) for in vivo tests.
Results and discussion
Composition of raw, cooked and NDF beans
Table 1 shows the protein, lipid, ash, fiber in raw and cooked beans (Phaseolus vulgaris L.), yield of non-digestible fraction from cooked beans, resistant starch and protein digestibility in NDF of cv. Bayo Madero. Protein content improved from 18.7 to 19.7% with the cooking process. Similar protein contents were reported previously (20 to 29%) for different bean varieties grown in Mexico.27 The protein content in the NDF sample of Bayo Madero (Table 1) was lower (17.1%) than the raw (18.7%) and cooked bean (19.7%) and with high digestibility (81.4%) suggesting that most of the protein is digested and therefore highly bioavailable in the upper gastrointestinal tract and only a small amount reaches the colon, indicating its relatively low contribution to the colonic fermentation. Lipid content was 1.92 and 1.13% in raw and cooked Bayo Madero beans, respectively (Table 1), similar to a previous report28 for Mesoamerican and Andean bean varieties. Ash content, an indicator of iron, potassium and other mineral contents, in raw and cooked beans was higher compared to NDF (Table 1) and within the range reported for four beans cultivars.29 Common beans are an important source of dietary fiber,4 with the soluble fiber increasing significantly with the cooking process (Table 1) similar to those observed for black, navy and red bean cultivars.30 This increase may be due to the thermal dissociation of complex fiber structures that releases the soluble fiber, thereby increasing their availability for quantification. The total, soluble and insoluble fiber values were similar to those of four bean varieties reported by Bednar et al.31 The yield and the total, soluble and insoluble fiber contents in NDF of Bayo Madero (Table 1) were higher than those reported by Feregrino-Pérez et al.12 for cultivar Negro 8025. This difference could be attributed to the variability in bean cultivars, but most importantly to strong environmental effects on bean composition,32 so it was interesting to evaluate the chemopreventive effect of cv Bayo Madero on ACF development. Our results for resistant starch were lower than those reported by Campos-Vega et al.4 for the same Bayo Madero cultivar grown at the same location but different harvest year, suggesting the strong environmental effect on the variability of this component. The resistant starch and the different types of fiber are susceptible fermentation substrates for colonic bacteria, hence the importance of quantification.32
Table 1 Chemical composition of raw, cooked and NDF from cultivar Bayo Madero common beans (Phaseolus vulgaris L.)a
|
Raw bean |
Cooked bean |
NDF |
NDF: non-digestible fraction. Results, expressed as percentage per gram of dry sample, are the average of two independents experiments with two repetitions ± standard error. Different letters by row express significant difference (α = 0.05) by Tukey test.
|
Protein |
18.7 ± 0.1 b |
19.7 ± 0.1 a |
17.1 ± 0.1 c |
Lipid |
1.9 ± 0.4 ab |
1.1 ± 0.1 b |
2.2 ± 0.1 a |
Ash |
4.4 ± 0.1 a |
4.2 ± 0.1 b |
2.3 ± 0.1 c |
Fiber total |
38.5 ± 0.6 b |
41.2 ± 1.0 b |
51.7 ± 1.3 a |
Fiber insoluble |
37.9 ± 0.6 b |
40.6 ± 1.0 b |
51.1 ± 1.3 a |
Fiber soluble |
0.6 ± 0.1 b |
0.7 ± 0.1 a |
0.6 ± 0.1 b |
Yield of non-digestible fraction |
|
43.8 ± 0.6 |
|
Resistant starch |
|
|
25.5 ± 0.2 |
Protein digestibility |
|
|
81.4 ± 1.3 |
Oligosaccharide can inhibit colon carcinogenesis by different mechanisms,7 its quantification is therefore critical. Table 2 shows oligosaccharide content in raw, cooked beans and NDF samples. Total oligosaccharide content decreased with cooking (59.6 to 55.8 mg g−1) (Table 2) and similar trends were observed for the individual oligosaccharide, raffinose, stachyose, and verbascose. The results are in agreement with those of Díaz-Batalla et al.17 for both raw and cooked beans of 10 different Mexican varieties. Total oligosaccharide content of NDF was significantly lower than those of raw and cooked samples, constituting only 28.3% of the total oligosaccharide content in cooked samples. The decrease in total oligosaccharide content in the NDF was due primarily to the extraction method, since substantial amount of oligosaccharide was lost in the supernatants after homogenization with water, tannic acid and acetone washes (data not shown).
Table 2 Oligosaccharide, total phenolic and condensed tannin contents of raw, cooked and NDF from common bean (Phaseolus vulgaris L.) Bayo Madero cultivara
Sample |
Raffinoseb (mg g−1 dry sample) |
Stachyoseb (mg g−1 dry sample) |
Verbascoseb (mg g−1 dry sample) |
Total oligosaccharidesb (mg g−1 dry sample) |
Total phenolicsc (mg eq gallic acid/g dry sample) |
Condensed tanninsc (mg eq (+)-catechin/g dry sample) |
NDF: non-digestible fraction. Different letters in each column express significant difference (α = 0.05) by Tukey test.
Results are the average of two independent experiments with duplicates ± standard error.
Results are the average of three independent experiments with duplicates ± standard error.
|
Raw |
12.3 ± 0.1 a |
42.9 ± 0.1 b |
4.6 ± 0.1 a |
59.6 ± 0.2 a |
0.7 ± 0.1 b |
7.4 ± 0.1 c |
Cooked |
10.4 ± 0.2 b |
43.3 ± 0.1 a |
2.1 ± 0.1 b |
55.8 ± 0.2 b |
1.0 ± 0.1 b |
8.9 ± 0.1 b |
NDF |
1.5 ± 0.1 c |
13.8 ± 0.1 c |
0.5 ± 0.1 c |
15.8 ± 0.1 c |
13.8 ± 0.7 a |
14.0 ± 0.1 a |
Total phenolics and condensed tannins (Table 2) were higher in cooked than raw beans. The increase in cooked bean could be attributed to thermal hydrolysis of phenolic complexes formed with other components such as protein and resistant starch in raw bean. Similar phenolic contents have been reported previously for black and pinto cultivars,33 different Mexican bean varieties,34 and for raw and cooked beans.12 NDF, as expected, had significantly higher amount of total phenolics and condensed tannins than raw and cooked samples, because these compounds are concentrated in the extract.
Food and water consumption and weight gain
Difference in weight gain of rats among groups was not significant (data not shown), suggesting that the induction of colon cancer with AOM had no influence on weight gain or food and water intake of the rats since evaluation was at the early cancer stage.
ACF analysis
Fig. 2 and 3 show the ACF in distal zone stained with methylene blue and hematoxylin and eosin, respectively. The dose of CB or NDF from cultivar Bayo Madero selected according to the per capita rural intake24 of a 70 kg body weight person in the Lagunera Region of Mexico did not induce ACF and showed no significant difference compared to the control group by both staining procedures. The results suggest that CB or NDF consumption do not contribute to generation of early lesions in the colon, which is a plus to promote the increase in bean consumption. The NDF + AOM treatment significantly decreased the ACF number in the distal colon section compared with AOM group (1.5 ± 0.72 vs. 6.6 ± 0.40 ACF/distal zone) by methylene blue stain. Hematoxylin and eosin stain showed the same tendency with a significant decrease in the NDF + AOM group compared to AOM group (4.6 ± 0.68 vs. 10.5 ± 1.89 ACF/distal zone) (Fig. 4). In turn, CB + AOM also decreased the ACF number in the distal colon section compared with AOM group by both stain (0.8 ± 0.20 and 4.4 ± 1.81 ACF/distal zone by methylene blue and hematoxylin and eosin stains, respectively). These data suggest that both CB and NDF can function as preventive treatment at an early or initiation stage of colon cancer. The results are consistent with our previous study,12 where similar chemoprotective effect of polysaccharide extract (PE) from Negro 8025 bean was observed in AOM-induced rats, with significant decrease in ACF in the PE + AOM group compared to AOM group (24.5 ± 8.2 vs. 35.0 ± 18.9 ACF/distal zone) using methylene blue stain. ACF reduction in the present study was 77.3 and 56.2% in the NDF + AOM treatment with methylene blue and HE, respectively, similar to the 70% observed with PE from Negro 8025 bean.12
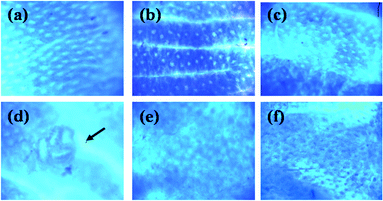 |
| Fig. 2 Distal colon tissue stained with methylene blue. (a) Control; (b) CB; (c) NDF; (d) AOM; (e) CB + AOM; (f) NDF + AOM. Magnification ×40. The ACF (indicated by an arrow), consisting of four large and elliptical crypts and a thicker epithelial lining compared to adjacent crypts were darkly stained. CB: cooked bean; NDF: non-digestible fraction; AOM: azoxymethane. | |
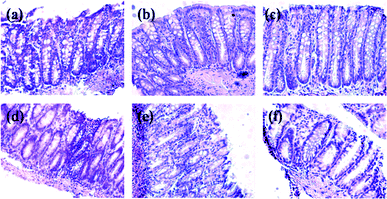 |
| Fig. 3 Distal colon tissue stained with hematoxylin and eosin. (a) Control; (b) CB; (c) NDF; (d) AOM; (e) CB + AOM; (f) NDF + AOM. Magnification ×20. The ACF (indicated by arrows) increased staining intensity of epithelial cytoplasm and presented irregular elongation of the ducts. Some ACF presented conical shape of the focus. CB: cooked bean; NDF: non-digestible fraction; AOM: azoxymethane. | |
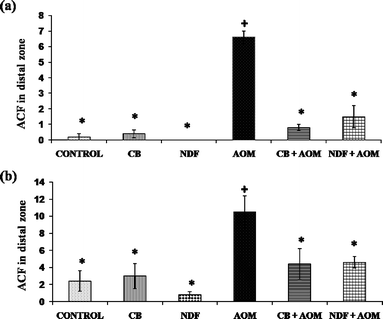 |
| Fig. 4 Analysis of aberrant crypt foci (ACF) in distal zone stained with (a) methylene blue; (b) hematoxylin and eosin. CB: cooked bean; NDF: non-digestible fraction; AOM: azoxymethane. Each value is the mean of ACF by distal zone ± standard error. * Mean statistically significant compared to the AOM treatment (Dunnett α = 0.05). + Mean statistically significant compared to the negative control (Dunnett α = 0.05). | |
SCFA production in cecal, colonic, and fecal contents
SCFA production was not significantly different among groups in cecal, colonic and fecal contents (Fig. 5).
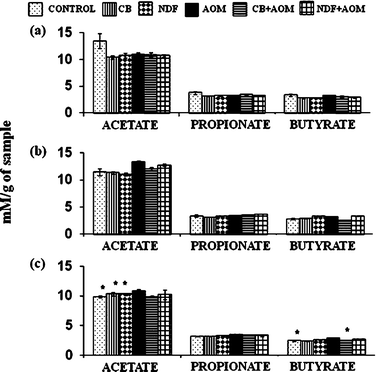 |
| Fig. 5 Concentration of SCFA in (a) cecal, (b) colonic and (c) fecal contents. CB: cooked bean; NDF: non-digestible fraction; AOM: azoxymethane. Results expressed as mM per g sample, are the average of 3 independent experiments with 3 repetitions ± standard error. * Mean statistical significant in comparison to the AOM treatment (Dunnett α = 0.05). | |
However, the fecal content of the NDF + AOM group showed a slight increase in propionic (3.71 ± 0.03 mM g−1 of sample) and butyric acid concentrations (3.34 ± 0.02 mM g−1 of sample) compared with those of AOM group (3.48 ± 0.01 and 3.21 ± 0.01 mM g−1 of sample of propionic and butyric acids, respectively). The highest SCFA concentration was present in fecal content. Our results differ from those reported by Feregrino-Perez et al.,12 where SCFA production was higher in cecal content relative to colonic and fecal contents in AOM-induced rats and those treated with Negro 8025 bean or its polysaccharide extract. These differences can be due to the bean used, Negro 8025, and the composition of its NDF or its fermentation capacity, thereby suggesting that Bayo Madero and its NDF could produce more SCFA in fecal content. In the present study, the butyric acid concentration in NDF + AOM group was 2.86 mM, 2.63 and 3.34 mM in cecal, colonic and fecal content, respectively, concentration sufficient to produce a protective effect in the colon according to the literature. For example, Hofmanová et al.35 demonstrated that 3 mM of butyric acid decreased proliferation and induced cell cycle arrest, apoptosis and differentiation on different cell lines of human colon cancer.
Furthermore, the presence and activity of other NDF components such as phenolic compounds and galactoligosaccharides, quantifed in this study, and others as bioactive peptides, may influence those preventing ACF development. These compounds have been shown to participate in modulation of genes and proteins involved in cell cycle arrest, apoptosis, and other cellular processes.36,37,38 Non-digestible galactooligosaccharides increase beneficial bacterial species and have been implicated in interactions between epithelial and bacterial cells, implying that these compounds also have the capacity to inhibit the binding of pathogens to cell surfaces by acting as competitive receptors, resulting in reduced activity of enzymes capable of reactivating the colon carcinogens.39 Both treatments, CB and NDF contain considerable amount of galactooligosaccharide, as described above for the protection mechanism against ACF development. CB and NDF also contain phenolic compounds, that may effectively induce programmed cell death, differentiation, and growth inhibition in transformed colonocytes by acting at the mRNA levels of genes involved in these processes; probably, the SCFA derived from the polyphenols during gut flora-mediated fermentation could also affect these processes.37,40 Finally, bioactive peptides with potential anticancer activity probably present in NDF may pass intact from the gastrointestinal tract and are released into the colon. Bioactive peptides from different legumes have high anti-cancer potential by inducing apoptosis through caspase 3 activation, topoisomerase 2 inhibition or reduction of NF-kappa-B and interleukin-6 activation.36
Effect of CB and NDF on β-glucuronidase activity
The β-glucuronidase enzymatic activity is relevant in colon carcinogenesis because it has the ability to hydrolyze many glucuronide conjugates and thus may release active carcinogenic metabolites in the intestinal lumen.41 Diets supplemented with different types of fiber have been suggested to contribute to colon cancer inhibition by increasing non-pathogenic bacteria, fiber fermentation and reduction of bacterial β-glucuronidase activity. CB and NDF consumption in AOM-induced groups significantly decreased the β-glucuronidase activity with respect to AOM (Fig. 6). This decrease was accompanied by protection against precancerous lesions induced by the chemical carcinogen (AOM), suggesting that reduction of ACF development may be associated with a decrease in cecal, colonic and fecal activity of this enzyme, because insufficient amount of the enzyme prevents carcinogen release in the colon and thus cannot induce the damage produced in the AOM group.
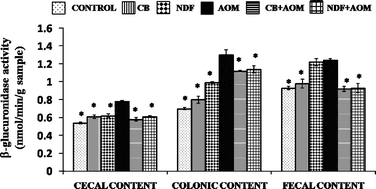 |
| Fig. 6 Effect of CB and NDF on β-glucuronidase activity in cecal, colonic and fecal contents. CB: cooked bean; NDF: non-digestible fraction; AOM: azoxymethane. Results are the average of 3 independent experiments with 3 repetitions ± standard error. Results are expressed as nmol phenolphthalein liberated/min g−1 sample. * Mean statistical difference in comparison to the AOM-treated group (Dunnett α = 0.05). | |
The inhibition of β-glucuronidase activity agrees with those observed by Nakanishi et al.,42 in colonic and cecal contents of AOM-induced rats with a diet high in resistant starch and probiotics C. butyricum. Similarly, Manoj et al.43 found a decrease of β-glucuronidase activity in colonic and fecal contents of DMH-induced rats on diets complemented with black gram and coconut kernel. The results suggest the reduction on β-glucuronidase activity and the presence of other bioactive compounds such as galactooligosaccharides, phenolic compounds and bioactive peptides in the CB and NDF mitigate ACF development.
Conclusions
Our results suggest that seeds of the cooked common bean cultivar Bayo Madero are excellent sources of protein, soluble and insoluble fiber and polysaccharide. Furthermore, cooked common bean Bayo Madero (CB) and its non-digestible fraction (NDF) have significant amount of oligosaccharide, resistant starch, total phenolics and condensed tannins, compounds that are all related to a lower risk of developing colon cancer. Bayo Madero and its NDF did not induce the ACF formation and demonstrated a chemoprotective effect against AOM treatment, suggesting that consumption of common beans prevent early stage colon cancer development in vivo. CB and NDF treatments significantly reduced β-glucuronidase activity thereby indicating a strong probiotic effect exerted by these compounds. These findings suggest that the suppression of aberrant crypt foci (ACF) formation is strongly influenced by the decrease in the β-glucuronidase activity in the colon, probably by altering the metabolic activities of the intestinal microflora that prevents AOM release in the colon. On the other hand, this investigation together with our previous similar study12 with Negro 8025 bean indicate that early stage of colon carcinogenesis, evaluated by ACF, in vivo is modulated by different mechanisms depending on the cultivar and composition of the common bean and its non-digestible fraction.
Notes and references
- Instituto Nacional de Estadísticas, Geografía e Informática, Información sobre Tumores Malignos, INEGI, México, 2008, http://www.inegi.org.mx Search PubMed.
- A. E. Millen, A. F. Subar, B. I. Graubard, U. Peteres, R. B. Hayes, J. L. Weissfeld, L. A. Yokochi and R. G. Ziegler, Am. J. Clin. Nutr., 2007, 86, 1754–1764 CAS.
- S. A. Bingham, T. Norat, A. Moskal, P. Ferrari, N. Slimani, F. Clavel-Chapelon, E. Kesse, A. Nieters, H. Boeing, A. Tjønneland, K. Overvad, C. Martinez, M. Dorronsoro, C. A. González, E. Ardanaz, C. Navarro, J. R. Quirós, T. J. Key, N. E. Day, A. Trichopoulou, A. Naska, V. Krogh, R. Tumino, D. Palli, S. Panico, P. Vineis, H. B. Bueno-de-Mesquita, M. C. Ocké, P. H. M. Peeters, G. Berglund, G. Hallmans, E. Lund, G. Skeie, R. Kaaks and E. Riboli, Cancer Epidemiol., Biomarkers Prev., 2005, 14, 1552–1556 CrossRef CAS.
- R. Campos-Vega, R. Reynoso-Camacho, G. Pedraza-Aboytes, J. A. Acosta-Gallegos, S. H. Guzman-Maldonado, O. Paredes-Lopez, B. D. Oomah and G. Loarca-Piña, J. Food Sci., 2009, 74, 59–65.
- E. Lanza, T. J. Hartman, P. S. Albert, R. Shields, M. Slattery, B. Caan, E. Paskett, F. Iber, J. W. Kikendall, P. Lance, C. Daston and A. Schatzkin, J. Nutr., 2006, 136, 1896–1903 CAS.
- G. Bobe, K. G. Barrett, R. A. Mentor-Marcel, U. Saffiotti, M. R. Young, N. H. Colburn, P. S. Albert, M. R. Bennink and E. Lanza, Nutr. Cancer, 2008, 60, 373–381 Search PubMed.
- K. Swennen, C. M. Courtin and J. A. Delcour, Crit. Rev. Food Sci. Nutr., 2006, 46, 459–471 CrossRef CAS.
- T. Kautenburger, G. Beyer-Sehlmeyer, G. Festag, N. Haag, S. Kühler, A. Küchler, A. Weise, B. Marian, W. H. M. Peters, T. Liehr, U. Claussen and B. L. Pool-Zobel, J. Cancer Res. Clin. Oncol., 2005, 131, 692–700 CrossRef CAS.
- L. Wang, H. S. Luo and H. Xia, J. Int. Med. Res., 2009, 37, 803–811 Search PubMed.
- M. Comalada, E. Bailón, O. de Haro, F. Lara-Villoslada, J. Xaus, A. Zarzuelo and J. Gálvez, J. Cancer Res. Clin. Oncol., 2006, 132, 487–497 CrossRef CAS.
- J. W. Finley, J. B. Burrell and P. G. Reeves, J. Nutr., 2007, 137, 2391–2398 CAS.
- A. A. Feregrino-Pérez, L. C. Berumen, G. García-Alcocer, R. G. Guevara-González, M. Ramos-Gómez, R. Reynoso-Camacho, J. A. Acosta-Gallegos and G. Loarca-Piña, J. Agric. Food Chem., 2008, 56, 8737–8744 CrossRef CAS.
- R. Campos-Vega, R. G. Guevara-Gonzalez, B. L. Guevara-Olvera, B. D. Oomah and M. G. F. Loarca-Piña, Food Res. Int., 2010, 43, 1057–1064 CrossRef CAS.
- X. O. Aparicio-Fernández, L. Manzo-Bonilla and G. F. Loarca-Piña, J. Food Sci., 2005, 70, 73–78.
- Association of Official Analytical Chemists, Official Methods of Analysis, 17th edn, AOAC, Arlington, VA, 2002, Methods 920.87, 920.39, 923.03, 991.43 Search PubMed.
- R. H. Kurtzman and W. U. Halbrook, Appl. Microbiol., 1970, 20, 715–719 Search PubMed.
- L. Díaz-Batalla, J. M. Widholm, G. C. Fahey, E. Castaño-Tostado and O. Paredes-López, J. Agric. Food Chem., 2006, 54, 2045–2052 CrossRef CAS.
- M. Muzquiz, C. Burbano, G. Ayet, M. M. Pedrosa and C. Cuadrado, Biotechnol. Agron. Soc., Environ., 1999, 3, 210–216 Search PubMed.
- A. Cardador-Martínez, E. Castaño-Tostado and G. Loarca-Piña, Food Addit. Contam., Part A, 2002, 19, 62–69 Search PubMed.
- K. Nurmi, V. Ossipov, E. Huakioja and K. Pihlaja, J. Chem. Ecol., 1996, 22, 2023–2040 CrossRef CAS.
- S. S. Deshpande and M. Cheryan, J. Food Sci., 1985, 50, 905–910 CrossRef CAS.
- F. Saura-Calixto, I. Goñi, L. Bravo and E. Mañas, J. Food Sci., 1993, 58, 642–643 CrossRef CAS.
- H. W. Hsu, D. L. Vavak, L. D. Satterlee and G. A. Miller, J. Food Sci., 1977, 42, 1269–1273 CrossRef CAS.
- L. M. Del Razo, G. G. Garcia-Vargas, J. Garcia-Salcedo, M. F. Sanmiguel, M. Rivera, M. C. Hernandez and M. E. Cebrian, Food Chem. Toxicol., 2002, 40, 1423–1431 CrossRef CAS.
- R. P. Bird, Cancer Lett., 1987, 37, 147–151 CrossRef CAS.
- W. H. Fishman, B. Springer and R. Brunetti, J. Biol. Chem., 1948, 173, 449 CAS.
- C. Reyes-Moreno and O. Paredes-López, Crit. Rev. Food Sci. Nutr., 1993, 33, 227–286 CrossRef CAS.
- F. L. Da Silva, V. Monteze Guimarães, E. Gonçalves de Barros, M. Alves Moreira, L. A. Dos Santos Dias, M. G. de Almeida Oliveira, I. Chamel José and S. Tavares de Rezende, Plant Foods Hum. Nutr., 2006, 61, 87–89.
- A. Vargas-Torres, P. Osorio-Díaz, J. Tovar, O. Paredes-López, J. Ruales and L. A. Bello-Pérez, Starch/Staerke, 2004, 56, 74–78 Search PubMed.
- M. Herrera, E. P. González and J. G. Romero, ALAN., 1998, 48, 179–182 Search PubMed.
- G. E. Bednar, A. R. Patil, S. M. Murray, C. M. Grieshop, N. R. Merchen and G. C. Fahey, J. Nutr., 2001, 131, 276–286 CAS.
- A. Florez, M. Pujolà, J. Valero, E. Centelles, A. Almirall and F. Casañas, Food Chem., 2009, 113, 950–956 CrossRef CAS.
- L. G. Espinosa-Alonso, A. Lygin, J. M. Wildholm, M. E. Valverde and O. Paredes-Lopez, J. Agric. Food Chem., 2006, 54, 4436–4444 CrossRef CAS.
- E. G. De Mejía, S. H. Guzmán-Maldonado, J. A. Acosta-Gallegos, R. Reynoso-Camacho, E. Ramírez-Rodríguez, J. L. Pons-Hernández, M. M. González-Chavira, J. Z. Castellanos and J. D. Nelly, J. Agric. Food Chem., 2003, 51, 5962–5966 CrossRef CAS.
- J. Hofmanová, A. Vaculová, Z. Koubková, M. Hyzd'alová and A. Kozubík, Mol. Nutr. Food Res., 2009, 53, S102–S113.
- E. G. De Mejía and V. I. Prisecaru, Crit. Rev. Food Sci. Nutr., 2005, 45, 425–445 CrossRef CAS.
- S. Veeriah, T. Hofmann, M. Glei, H. Dietrich, F. Will, P. Schreier, B. Knaup and B. L. Pool-Zobel, J. Agric. Food Chem., 2007, 55, 2892–900 CrossRef CAS.
- S. Kuntz, C. Kunz and S. Rudloff, Br. J. Nutr., 2009, 101, 1306–1315 CrossRef CAS.
- G. Tzortzis, A. K. Goulas, J. M. Gee and G. R. Gibson, J. Nutr., 2005, 135, 1726–1731 CAS.
- U. Wenzel, S. Kuntz, M. D. Brendel and H. Daniel, Cancer Res., 2000, 60, 3823–3831 CAS.
- J. Rafter, Br. J. Nutr., 2002, 88, S89–S94 CrossRef CAS.
- S. Nakanishi, K. Kataoka, T. Kuwahara and Y. Ohnishi, Microbiol. Immunol., 2003, 47, 951–958 Search PubMed.
- G. Manoj, B. S. H. Thampi, S. Leelamma and P. V. G. Menon, Plant Foods Hum. Nutr., 2001, 56, 13–21 CrossRef CAS.
|
This journal is © The Royal Society of Chemistry 2010 |
Click here to see how this site uses Cookies. View our privacy policy here.