DOI:
10.1039/C0FO00048E
(Paper)
Food Funct., 2010,
1, 278-283
Protective role of air potato (Dioscorea bulbifera) of yam family in myocardial ischemic reperfusion injury
Received
21st June 2010
, Accepted 19th October 2010
First published on
15th November 2010
Abstract
Hydroalcoholic extract of Dioscorea bulbifera (DB), a yam variety called air potato, was tested for its protective effect on myocardial ischemic/reperfusion (I/R) injury in rats due to apoptosis and necrosis. Myocardial I/R injury was induced by 30 min ischemia followed by 2 h reperfusion by perfusing isolated rat hearts with Krebs Henseilet bicarbonate (KHB) buffer in a Langendorff set up. Pretreatment of DB (150 mg kg−1 body weight) for 30 days significantly reduced myocardial infarct size and improved the ventricular function (aortic flow and coronary flow, LVDP, LVmax dp/dt). Role of DB on apoptosis was also evaluated by determining caspase 3 as well as by examining pro-apoptotic and anti-apoptotic proteins Bax and Bcl2 by Western blot analysis followed by TUNEL assay. DB also prevented I/R-mediated down regulation of survival protein Akt and HO-1. Our results indicated that Dioscorea bulbifera could ameliorate myocardial ischemia and reperfusion injury by improving ventricular function and inhibition of cardiomyocyte necrosis and apoptosis.
Introduction
Ischemia and reperfusion (I/R) causes myocardial infarction potentiated by both necrosis and apoptosis.1 Moreover, accumulating evidence indicates that, apart from necrosis, apoptosis contributes significantly to post ischemic cardiomyocyte death, suggesting that therapeutic intervention that inhibits apoptotic cell death may attenuate I/R-induced cardiomyocyte injury.2,3 Recently, there have been many scientific claims that botanicals provide cardioprotection against (I/R) injury in a variety of experimental models via multiple mechanisms including inhibition of apoptotic cell death.4–6
Dioscorea bulbifera (DB), commonly known as aerial yam or air potato, belonging to the Dioscoreacae family, is widely distributed in India, Ceylon, the Malay Peninsula, Australia, East Africa and Brazil.7 It is one of the important medicinal plants used in indigenous systems of medicine in Asia.8Dioscorea species are most noted for the abundance of diosgenin, a steroidal saponin used as a precursor for the synthesis of corticosteroids, estrogen, contraceptives, and spiranolactones.9 Recently, diosgenin has been found to ameliorate myocardial infarction by its anti-lipoperoxidative activity.10 Hence, this study aimed to investigate whether the extract of Dioscorea bulbifera could reduce myocardial I/R injury-induced apoptosis in the rat heart.
Materials and methods
The solvents used for plant extraction were of analytical grade and obtained from Sisco Research Laboratory (SRL), India. All other chemicals used were of analytical grade and were obtained from Sigma-Aldrich Chemical Company (St. Louis, MO), unless otherwise specified. Primary antibodies such as Akt, pAkt, Bax, Bcl2, HO-1, pro and cleaved caspase - 3 and glyceraldehyde-6-phosphate dehydrogenase (GAPDH) were obtained from Santa Cruz Biotechnology, Santa Cruz, CA.
Preparation of plant extract
Fresh Dioscorea bulbifera (DB) tubers were purchased from Ayurmed Biotech Pvt. Ltd, Mumbai, India, and it was taxonomically identified and authenticated by a taxonomist and a voucher specimen has been preserved for further reference. The tubers were cleaned, dried under shade at room temperature and coarsely pulverized using a mechanical grinder. The dried powder (1 kg) was extracted using a Soxhlet-extractor in 70% ethanol. Hydroalcoholic extracts were evaporated (free of solvents) using a rotary evaporator under reduced pressure at 40 °C. A brown concentrated hydroalcoholic extract was obtained (yield 8.52% w/w with respect to the dried starting material). The final product was then stored at room temperature in a dessicator for further use.
Animals
All animals used in this study received humane care in compliance with the Animal Welfare Act and other federal statutes and regulations relating to animals and experiments involving animals; the care adhered to principles stated in the Guide for the Care and Use of Laboratory Animals, NRC (Publication Number NIH 85-23, revised 1996). Sprague–Dawley male rats weighing between 250 and 300 g were used for the experiment. The rats were randomly assigned to two groups: control and hydroalcoholic extract of DB treated. The rats were fed ad libitum regular rat chow with free access to water. The experimental rats were gavaged with 1 ml of DB extract dissolved in water (150 mg kg−1 of body weight) for 30 days, whereas the control group of rats were gavaged 1 ml of the vehicle for the same period of time.
Isolated working heart preparation
At the end of 30 days, the rats were anesthetized with sodium pentobarbital (80 mg kg−1 of BW, ip) (Abbott Laboratories, North Chicago, IL) and anticoagulated with heparin sodium (500 IU/kg of BW, I.V) (Elkins-Sinn Inc., Cherry Hill, NJ) injection. After euthanising the rats, a thoracotomy was performed, and the heart was perfused in the retrograde Langendorff mode at 37 °C at a constant perfusion pressure of 100 cm of water (10 kPa) for a 5 min washout period.11 The perfusion buffer used in this study consisted of a modified Krebs–Henseleit bicarbonate buffer (KHB): sodium chloride, 118 mM; potassium chloride, 4.7 mM; calcium chloride, 1.7 mM; sodium bicarbonate, 25 mM; potassium biphosphate, 0.36 mM; magnesium sulfate, 1.2 mM; and glucose, 10 mM. The Langendorff set up was switched to the working mode following the washout period.
At the end of 10 min, after the attainment of steady state cardiac function, baseline functional parameters were recorded. The circuit was then switched back to the retrograde mode, and the hearts were perfused for 15 min with KHB for stabilization.12 The hearts were then subjected to 30 min of global ischemia followed by 2 h of reperfusion. The first 10 min of reperfusion was in the retrograde mode to allow for post-ischemic stabilization and thereafter, in the antegrade working mode to allow for assessment of functional parameters, which were recorded at 30, 60, and 120 min of reperfusion.
Assessment of ventricular function
Aortic pressure was measured using a Gould P23XL pressure transducer (Gould Instrument Systems Inc., Valley View, OH) connected to a side arm of the aortic cannula; the signal was amplified using a Gould 6600 series signal conditioner and monitored on a CORDAT II real-time data acquisition and analysis system (Triton Technologies, San Diego, CA).12 Heart rate (HR), left ventricular developed pressure (LVDP) (defined as the difference of the maximum systolic and diastolic aortic pressures), and the first derivative of developed pressure (dp/dt) were all derived or calculated from the continuously obtained pressure signal. Aortic flow (AF) was measured using a calibrated flow meter (Gilmont Instrument Inc., Barrington, IL), and coronary flow (CF) was measured by timed collection of the coronary effluent dripping from the heart.
Determination of infarct size
At the end of reperfusion, a 1% (w/v) solution of triphenyl tetrazolium (TTC) in phosphate buffer was infused into the aortic cannula for 20 min at 37 °C.13 The hearts were excised, and the sections (1 mm) of the heart were fixed in 2% paraformaldehyde, placed between two coverslips, and digitally imaged using a Microtek ScanMaker 600z. To quantify the areas of infarct in pixels, a NIH image 5.1 (a public domain software package) was used. The infarct size was quantified and expressed in pixels.
Immunohistochemical detection of apoptotic cells was carried out using the terminal dUTP nick-end labeling (TUNEL) method (Promega, Madison, WI).14 The heart tissues were immediately put in 10% formalin and fixed in an automatic tissue-fixing machine. The tissues were carefully embedded in molten paraffin in metallic blocks, covered with flexible plastic molds and kept under freezing plates to allow the paraffin to solidify. The metallic containers were removed, and tissues became embedded in paraffin on the plastic molds. Prior to analyzing tissues for apoptosis, tissue sections were deparaffinized with xylene and washed in succession with different concentrations of ethanol (absolute, 95%, 85%, 70%, 50%). Then the TUNEL staining was performed according to the manufacturer's instructions. The fluorescence staining was viewed with a fluorescence microscope (AXIOPLAN2 IMAGING) (Carl Zeiss Microimaging, Inc., NY) at 520 nm for green fluorescence of fluorescein and at 620 nm for red fluorescence of propidium iodide. The number of apoptotic cells was counted throughout the slides and expressed as a percent of total myocyte population.
Preparation of subcellular fractions
Cardiac tissues were homogenized in 1 mL of buffer A (25 mM Tris-HCl, pH 8, 25 mM NaCl, 1 mM sodium orthovanadate, 10 mM NaF, 10 mM sodium pyrophosphate, 10 nM okadaic acid, 0.5 mM EDTA, 1 mM PMSF, and 1× protease inhibitor cocktail) in a Polytron homogenizer. Homogenates were centrifuged at 3000 rpm at 4 °C for 10 min, and the nuclear pellet was resuspended in 500 μL of buffer A with 0.1% Triton X-100. Supernatant from the above centrifugation was further centrifuged at 10,
000 rpm at 4 °C for 20 min, and the resultant supernatant was used as cytosolic extract. The mitochondrial pellet was resuspended in 200–300 μL of buffer A with 0.1% Triton X-100. The nuclei pellet and mitochondrial pellet were lysed by incubation for 1 h on ice with intermittent tapping. Homogenates were then centrifuged at 14
000 rpm at 4 °C for 10 min, and the supernatant was used as nuclear lysate and mitochondrial lysate, respectively. Cytosolic and nuclear extracts were aliquoted, snap frozen, and stored at −80 °C until use. Total protein concentrations in cytosolic, and mitochondrial extracts were determined using a BCA Protein Assay Kit (Pierce, Rockford, IL).
Western blot analysis
Western blot was performed to measure apoptosis-related proteins such as Bax, Bcl2, p-Akt, pro and cleaved caspase - 3 and HO-1. Proteins were separated in SDS-PAGE after estimating the total protein content in each sample. Following electrophoresis, proteins were transferred to nitrocellulose filters. Filters were blocked with TBS-T (20 mM Tris HCl pH 7.5, Tween 20) containing 5% (w/v) nonfat dry milk and probed with the respective primary antibodies overnight.14 All primary antibodies were used at the dilution of 1
:
1000. Protein bands were identified with horseradish peroxidase conjugated secondary antibody (1
:
2000 dilutions) and Western blotting luminol reagent (Santa Cruz Biotechnology). GAPDH was used as loading control. The resulting blots were digitized, subjected to densitometric scanning using a standard NIH image program, and normalized against loading control.
Statistical analysis
The values of myocardial functional parameters, total and infarct volumes and infarct sizes are all expressed as the mean ± standard error of the mean (SEM). Analysis of variance test followed by Bonferroni's correction was first carried out to test for any differences between the mean values of all groups. If differences between groups were established, the values of the treated groups were compared with those of the control group by a modified t-test. The results were considered to be significant if p < 0.05.
Results
Effects of DB extract on left ventricular function
We first determined if the hearts of DB-treated animals displayed improved ventricular performance compared to those of control animals. As shown in Fig. 1, aortic flow, LVDP, and LVmax dp/dt of DB treated hearts consistently displayed improved performance compared to the control group during post ischemic reperfusion. The heart rate did not vary significantly between the two groups. Coronary flow of the DB group was higher compared to the control group only at the end of 60 min and 2 h of reperfusion.
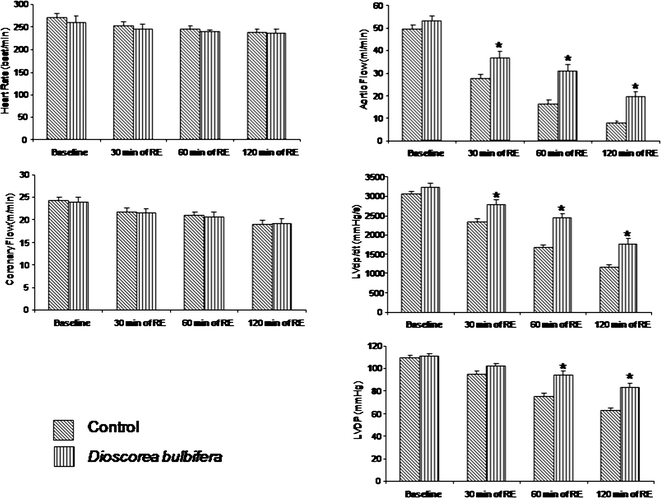 |
| Fig. 1 Effect of ischemia/reperfusion on heart rate, aortic flow, LVDP, Maximum first derivative of developed pressure, coronary flow of control rat heart and DB treated rat heart. Results are shown as mean ± SEM. * p < 0.05 vs. control. | |
Effects of DB on myocardial infarct size
Myocardial infarct size at the end of 30 min of ischemia and 2 h of reperfusion as determined by TTC staining method was about 35 ± 1.17% normalized to area of risk (Fig. 2). There was no infarction when the hearts were perfused with the KHB buffer without subjection to ischemia and reperfusion (control) (data not shown). DB significantly reduced myocardial infarct size to about 20 ± 2.64% as compared to the control group.
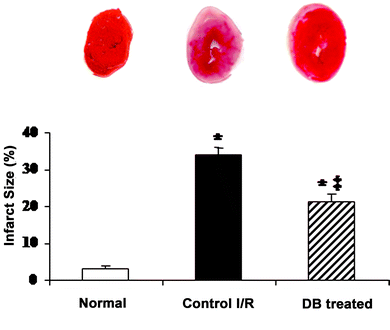 |
| Fig. 2 Effect of DB on myocardial infarct size. Results are shown as mean ± SEM. * p < 0.05 vs. normal, ‡ p < 0.05 vs. ischemic control, n = 3 in each group. | |
Cardiomyocyte apoptosis was determined by TUNEL assay. As shown in Fig. 3, it was about 39.42 ± 3.5% at the end of reperfusion in case of control group. There were reduced number of apoptotic cells (4.96 ± 0.75%) in the non-treated control hearts perfused with the Krebs–Henseleit bicarbonate buffer without subjection to ischemia and reperfusion. DB significantly reduced the number of apoptotic cardiomyocytes to 16.89 ± 1.7% revealing that DB exhibits anti-apoptotic activity.
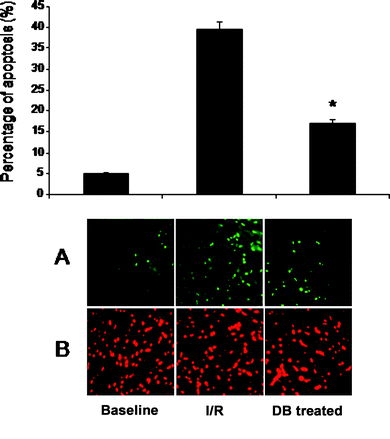 |
| Fig. 3 Effects of DB on cardiomyocyte apoptosis. Panel A is total number of apoptotic cells (green channels). Panel B is total number of cells (red channel). Values are Mean ± SEM. * p < 0.05 vs. ischemic control. Representative photomicrographs are shown below the bar graphs. Results are shown as mean ± SEM. * p < 0.05 vs. ischemic control. | |
Modulation of pro- and anti-apoptotic proteins by DB
We examined the modulation of the pro- and anti-apoptotic protein signals triggered by DB extract. Fig. 4 shows the results. I/R, regardless of reperfusion time, significantly reduced the expression of the anti-apoptotic protein Bcl2, but increased the expression of pro-apoptotic protein Bax compared to the control value. DB extract significantly increased Bcl2 expression and decreased Bax expression. Taken together, DB treatment reduced the ratio of Bax/Bcl2 as compared to the control hearts. I/R reduced the phosphorylation of Akt, which was prevented with DB (Fig. 5). Similarly there was an upregulation of procaspase - 3 in the DB treated group whereas a significant downregulation was observed in the Cleaved caspase - 3 when compared to that of the ischemic/reperfusion group. To determine the effect of the DB extract on phase II enzyme HO-1 with proven cardioprotective ability, we studied the expression of the HO-1 protein. As shown in Fig. 5, HO-1 was significantly reduced in the ischemic reperfused myocardium, and DB extract prevented the loss of HO-1.
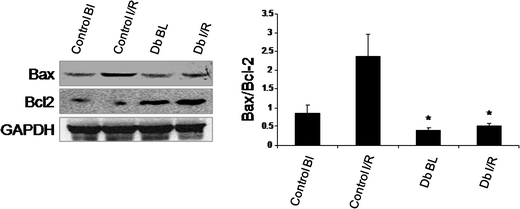 |
| Fig. 4 Western blot analysis of Bax, Bcl2 protein from the cytosolic fraction of control, ischemic and DB treated heart samples. GAPDH was used as the loading control. Figures are representative images of three different groups, and each experiment was repeated at least thrice. Bar diagram for Bax/Bcl2 ratio. Results are shown as mean ± SEM. * p < 0.05 vs. ischemic control. | |
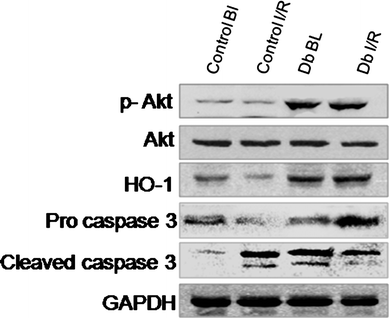 |
| Fig. 5 Western blot analysis of p-Akt, heme oxygenase protein HO-1, procaspase 3 and cleaved caspase 3 from the cytosolic fraction of control, ischemic and DB treated heart samples. GAPDH was used as the loading control. Figures are representative images of three different groups, and each experiment was repeated at least thrice. | |
Discussion
In the present study, we showed that administration of DB extract in vivo improved cardiac function as well as reduced the myocardial infarct size and cardiomyocyte apoptosis following myocardial I/R in rats. We further found that the cardioprotective effects of the yam extract could be explained in part by its modulation of apoptosis-related protein signals.
Although there are some reports on the protective effect of wild yam in postmenopausal women for a healthy heart, there are no studies to explore the relationship between the putative anti-apoptotic effects of DB on the functional recovery of the ischemic reperfused myocardium. The present study demonstrated that DB treatment preserved the left ventricular function as reflected by a significant increase in the indices of contractility and relaxation.
It is well established that cell death during I/R occurs via necrotic and apoptotic pathways.15,16 The mitochondrial death pathway appears to play an important role in the execution of apoptosis in cardiac myocytes.17 We found that DB reduced I/R injury with an associated reduction in apoptotic cell death as evidenced by the modulation of genes related to apoptosis especially Bcl2 and Bax. It is well known that anti-apoptotic protein Bcl2 has potent cardioprotective effects.18 It exerts cardioprotection by multiple mechanisms such as antioxidant effect, inhibition of pro-apoptotic proteins, mitochondrial membrane stabilization and inhibition of release of cytochrome C. Pro-apoptotic protein Bax has been reported to be activated in cardiac cells in response to oxidative stress and during ischemia.19 In the present study, Bax activity was down-regulated in the DB treated rat hearts as compared to the control rat hearts resulting in considerable reduction in the Bax/Bcl2 ratio. The altered Bcl − 2/Bax ratio attenuates cleavage of Caspase 3 which is well shown in our results. There was a significant expression of Procaspase 3 in the DB treated group and a down regulated expression of Cleaved Caspase 3.
Polyphenols and flavonoids from plant products have a direct action on the up regulation of phase II enzyme like HO-1 in a dose dependent manner.21 One of the possible mechanisms by which DB modulates apoptosis may be due to it antioxidant action. The results of the present study suggest such a possibility as DB extract resulted in the overexpression of HO-1. Cardio-selective over expression of HO-1 protein improves vascular function by increasing superoxide dismutase and catalase activity.22 In addition, up regulation of HO-1 exerts a cardio-protective effect after myocardial I/R in mice, and this effect is probably mediated via the anti-apoptotic action of HO-1.23 The results of the present study indicating significant reduction in the expression of HO-1 after I/R in the control heart is consistent with the results of a previous study.20 Finally, DB prevented I/R-induced down regulation of the survival protein Akt phosphorylation.
Dioscorea bulbifera contains a large number of polyphenols and organic acids, some of which may function as antioxidants. It contains significant amount of polyphenols and possesses potent antioxidant action.24 The polyphenolic components of yam range from 13 to 166 mg/100 gm comprising catechins, chlorogenic acids, proanthocyanidins and anthocyanins.25 Moreover it also contains flavonoids such as kampferol-3,5-dimethyl ether, myricetin, quercitin 3-O-galactopyranoside, diosbulbin and myricetin 3-O-galactopyranroside.26 Most importantly, Dioscorea bulbifera yam contains the steroid diosgenin, the principal material to manufacture birth control pills as it can overcome the negative effects of DHEA and progesterone. A recent study revealed that diosgenin in DB exhibits cardioprotection by reducing lipid peroxidation and membrane liability to lysosomal damage in isoproterenol induced myocardial infarction.10
In conclusion, the results of the present study suggest that Dioscorea bulbifera extract ameliorates rat myocardial ischemia and reperfusion injury with an associated reduction in apoptotic cell death. It is an important endangered medicinal plant that is commercially exploited for the extraction of diosgenin, a precursor of steroid used to produce birth control pills. The results that Dioscorea bulbifera, a yam variety called air potato can also protect the heart from I/R injury demonstrates its effectiveness as molecular target for ischemic heart as it might be useful for potential drug development for this purpose.
Acknowledgements
The first author is grateful to the Department of Science and Technology, Govt of India for providing the BOYSCAST Fellowship (2007) to work in the laboratory of Dr Dipak K. Das funded by the NIH at UCONN Health Centre, Farmington, CT, USA. The research was supported in part by NIH HL 34360, HL 22559 and HL 33889.
References
- A. Hamacher-Brady, N. R. Brady and R. A. Gottlieb, Cardiovasc. Drugs Ther., 2006, 20, 445–462 CrossRef CAS.
- R. A. Gottlieb and R. L. Engler, Ann. N. Y. Acad. Sci., 1999, 874, 412–426 CrossRef CAS.
- Y. C. Jin and Y. M. Kim, Vasc. Pharmacol., 2009, 50, 71–77 CrossRef CAS.
- Y. C. Jin, K. J. Kim, Y. M. Kim, Y. M. Ha, H. J. Kim, E. J. Yun, K. H. Bae, Y. S. Kim and S. S. Kang, Exp. Biol. Med., 2008, 233, 280–1288 Search PubMed.
- I. T. Nizamutdinova, Y. C. Jin, J. S. Kim, M. H. Yean, S. S. Kang, Y. S. Kim, J. H. Lee, H. G. Seo and H. J. Kim, Planta Med., 2008, 74, 14–18 CrossRef CAS.
- S. Mukherjee, H. Gangopadhyay and D. K. Das, J. Agric. Food Chem., 2008, 56, 609–617 CrossRef CAS.
- E. S. Ayensu and D. G. Coursey, Econ. Bot., 1972, 26, 301–318.
- C. Djerassi, Steroids, 1992, 57, 631–641 CrossRef CAS.
- V. P. Kamboj, Herbal Med. Curr. Sci., 2000, 78, 35–39 Search PubMed.
- K. S. Jayachandran, H. R. Vasanthi and G. V. Rajamanickam, Mol. Cell. Biochem., 2009, 327, 203–210 CrossRef CAS.
- D. T. Engelman, M. Watanabe, R. M. Engelman, J. A. Rousou, E. Kisin, V. E. Kagan, N. Maulik and D. K. Das, Cardiovasc. Res., 1995, 29, 133–140 CAS.
- I. Lekli and S. Das, J. Agric. Food Chem., 2008, 56, 5331–5337 CrossRef CAS.
- I. Lekli, G. Szabo, B. Juhasz, S. Das, M. Das, E. Varga, L. Szendrei, R. Geszteyli and J. Varadi, Am. J. Physiol.: Heart Circ. Physiol., 2007, 294, H859–866 CrossRef.
- S. Mukherjee and I. Lekli, Biochim. Biophys. Acta.: Mol. Basis Dis., 2008, 1782, 498–583 CrossRef CAS.
- N. Maulik and D. K. Das, Heart Failure Rev., 1999, 4, 165–175 Search PubMed.
- E. Murphy and C. Steenbergen, Physiol. Rev., 2008, 88, 581–609 CrossRef CAS.
- D. R. Green and J. C. Reed, Science, 1998, 281, 1309–1312 CrossRef CAS.
- H. R. Vasanthi, S. Mukherjee, I. Lekli, D. Ray, V. Gayathri and D. K. Das, J. Cardiovasc. Pharmacol., 2009, 53, 499–506 CrossRef CAS.
- A. B. Gustafasson and R. A. Gottlieb, J. Clin. Immunol., 2003, 23, 447–459 CrossRef.
- C. K. Andreadi, L. M. Howells, P. A. Atherfold and M. M. Manson, Mol. Pharmacol., 2006, 69, 1033–1040 CAS.
- Adam L. Kruger, S. Peterson, S. Turkseven, P. M. Kaminski, F. F. Zhang, S. Quan, M. S. Wolin and N. G. Abraham, Circulation, 2005, 111, 3126–3134 CrossRef.
- S. R. Vulapalli, Z. Chen, H. L. Balvin, C. T. Wang and C. S. Liang, Am. J. Physiol. Heart Circ. Physiol., 2002, 283, 688–694.
- M. Raj Bhandari and J. Kawabata, Food Chem., 2004, 88, 163–168 CrossRef CAS.
- I. Muzac Tucker, H. N. Asemota and M. H. Ahmad, J. Sci. Food Agric., 1993, 62, 219–224 CrossRef CAS.
- P. M. Kang, A. Haunstetter and H. Aoki, Circ. Res., 2000, 87, 118–125 CAS.
- H. Gao, M. Kuroyanagi, L. Wu, N. Kawahara, T. Yasuno and Y. Nakamura, Biol. Pharm. Bull., 2002, 25, 1241–1243 CrossRef CAS.
|
This journal is © The Royal Society of Chemistry 2010 |