DOI:
10.1039/C0FO00021C
(Paper)
Food Funct., 2010,
1, 269-277
Application of response surface methodology to optimize pressurized liquid extraction of antioxidant compounds from sage (Salvia officinalis L.), basil (Ocimum basilicum L.) and thyme (Thymus vulgaris L.)
Received
24th May 2010
, Accepted 26th July 2010
First published on
2nd November 2010
Abstract
The present study optimized pressurized liquid extraction (PLE) conditions using Dionex ASE® 200, USA to maximize the antioxidant activity [Ferric ion Reducing Antioxidant Power (FRAP)] and total polyphenol content (TP) of the extracts from three spices of Lamiaceae family (sage, basil and thyme). Optimal conditions with regard to extraction temperature (66–129 °C) and solvent concentration (32–88% methanol) were identified using response surface methodology (RSM). For all three spices, results showed that 129 °C was the optimum temperature with regard to antioxidant activity. Optimal methanol concentrations with respect to the antioxidant activity of sage and basil extracts were 58% and 60% respectively. Thyme showed a different trend with regard to methanol concentration and was optimally extracted at 33%. Antioxidant activity yields of the optimal PLE were significantly (p < 0.05) higher than solid/liquid extracts. Predicted models were highly significant (p < 0.05) for both total phenol (TP) and FRAP values in all the spices with high regression coefficients (R2) ranging from 0.651 to 0.999.
1. Introduction
Numerous studies have demonstrated that spices and their extracts have many useful technological and biological properties, for example, it is well known that spice extracts have potent antioxidant properties1,2 and more recent studies have shown that they may possess other useful biological properties such as anti-carcinogenic, anti-diabetic and anti-inflammatory properties.3 This has led to the use of extracts from spices in many food applications.4 The discovery of these beneficial properties has lead to intensive research some of which has indicated that these properties are derived from high levels of antioxidative polyphenols. For example, sage is particularly rich in polyphenols5,6 and several studies have shown that sage (Salvia officinalis L.) essential oil can improve the memory and has shown promise in the treatment of Alzheimer's disease.4 Basil (Ocimum basilicum L.) extracts have exhibited a spectrum of properties including bactericidal, anti-inflammatory, antioxidative, antiulcer, antidiarrheal, chemopreventive, blood-sugar lowering, a nervous system stimulatory and radiation protection.7–9 Beric et al.10 investigated protective effect of essential oil of basil against oxidative DNA damage and mutagenesis.
Thyme (Thymus vulgaris L.) also possesses various beneficial effects, e.g., antiseptic, carminative, antimicrobial, and antioxidative properties.11 Thyme and its extracts are used orally to treat dyspepsia and other gastrointestinal disturbances, cough due to cold, whooping cough, bronchitis, laryngitis and tonsillitis. The literature is indicating that the compounds responsible for these displayed biological activities are polyphenolic secondary metabolites, such as rosmarinic acid, carvacrol, caffeic acid, ferulic acid, thymol.12 Spice phenolics are an interesting target for optimization of extraction conditions, the extraction parameters can influence the composition of the extract. Moreover, spice extracts also named as oleoresins and essential oils, are traditionally extracted out using conventional solid/liquid extraction at atmospheric pressures.13 Pressurized liquid extraction is relatively a new automated technique, which uses high pressure and this allows the user to carry out extractions at temperatures above the boiling temperature of the solvent.14 The use of high pressures and temperatures increases the target compound's solubility, solvent diffusion rate and mass transfer, while decreasing the solvent viscosity and surface tension.15 PLE offers many advantages over traditional solid/liquid extraction techniques. Disadvantages of solid/liquid extraction methods include: the use of large quantities of toxic organic solvents, the involvement of elaborate labour pools, the long extraction times, low selectivity and/or low extraction yields. In contrast, PLE uses less solvent, has shorter extraction times, is automated and retains samples in an oxygen and light-free environment.16 Therefore, PLE could be considered as an efficient green extraction technique. A considerable number of studies have focussed on the use of PLE from an environmental perspective.17,18 Several authors have reported the use of PLE to extract specified target compounds from spices.19–22 The technique was recently shown to be particularly useful for maximizing the antioxidative properties of extracts from microalgae,23 anthocyanins from dried red grape skin,24 vitamin E rich oil from grape seeds.25 However, none have concentrated on optimizing the PLE conditions for maximizing the antioxidant activity of spice extracts using response surface methodology (RSM). To maximize antioxidant capacity of the extracts, PLE parameters such as temperature and solvent concentration, must be optimized. RSM is a statistical technique which allows the user to identify optimal conditions for selected responses while minimizing the number of experiments required. Central composite design (CCD) is the most popular form of RSM as it has been utilized by a number of researchers to optimize various food processing methods such as milling,26 and extraction.27 The objective of the work was to optimize the analytical scale extraction conditions (temperature and solvent concentration) of PLE using response surface methodologies. PLE has been recognized as a high throughput extraction system for analyzing target analytes but optimized conditions for extraction of antioxidant compounds from spices have not been given previously. Therefore the extraction conditions of PLE were optimized to get significantly (p < 0.05) higher efficiency in extraction than conventional solid/liquid extraction method. Previous work has indicated that methanol is a highly efficient solvent for analytical scale extraction of polyphenols. Preliminary work on extraction of phenolic antioxidants from spices showed that methanol had higher efficiency as a solvent than ethanol and water. Therefore, this efficient solvent was used to test the PLE process; its optimization and modeling can therefore be used for other solvents.
2. Materials and methods
2.1. Samples and reagents
Dried and powdered sage, basil and thyme were sourced from AllinAll Ingredients Ltd., Dublin, Ireland. Folin-Ciocalteu Reagent, sodium carbonate, sodium acetate anhydrous, acetic acid, ferric chloride hexahydrate, 2,4,6-tri(2-pyridyl)-s-triazine, 6-hydroxy-2,5,7,8-tetramethylchroman-2-carboxylic acid, gallic acid, caffeic acid and rosmarinic acid were purchased from Sigma-Aldrich.
PLE was performed on a Dionex ASE 200 (Dionex Corp., Sunnyvale, CA) system. Dried and powdered spices (0.5 g) were placed in between two layers of diatomaceous earth in a 22 mL Dionex (ASE 200) stainless-steel cell. The cells were equipped with a stainless steel frit and a cellulose filter (Dionex Corp.) at the bottom to avoid the collection of suspended particles in the collection vial. A dispersing agent (diatomaceous earth) was used to reduce the solvent volume used for the extraction. The extraction cell was arranged in the cell tray and was extracted using conditions specified using RSM guided experimental design. The range of solvent combinations and extraction temperatures used in PLE system are presented in Table 1.
Table 1 Combinations of methanol concentration and temperature with their coded terms obtained from RSM and their respective TP and FRAP values
Run |
Methanol % (coded term) |
Temperature (°C) (coded term) |
TP (g GAE/100 g DW) |
FRAP (g Trolox/100 g DW) |
Sage |
Basil |
Thyme |
Sage |
Basil |
Thyme |
1 |
60 (0) |
97(0) |
5.81 ± 0.006 |
3.05 ± 0.005 |
2.16 ± 0.002 |
15.24 ± 0.014 |
7.65 ± 0.026 |
5.92 ± 0.017 |
2 |
40(−1) |
75(−1) |
5.37 ± 0.007 |
2.47 ± 0.003 |
1.95 ± 0.004 |
14.36 ± 0.009 |
6.91 ± 0.016 |
5.00 ± 0.023 |
3 |
60(0) |
97(0) |
5.81 ± 0.012 |
3.07 ± 0.005 |
1.98 ± 0.001 |
15.17 ± 0.018 |
7.39 ± 0.023 |
5.54 ± 0.025 |
4 |
60(0) |
97(0) |
5.74 ± 0.008 |
3.08 ± 0.002 |
1.87 ± 0.005 |
15.30 ± 0.052 |
7.76 ± 0.043 |
5.67 ± 0.014 |
5 |
80(+1) |
120(+1) |
5.40 ± 0.006 |
3.15 ± 0.006 |
2.62 ± 0.010 |
15.33 ± 0.075 |
7.10 ± 0.056 |
6.90 ± 0.022 |
6 |
80(+1) |
75(−1) |
4.31 ± 0.004 |
1.57 ± 0.001 |
1.75 ± 0.005 |
12.77 ± 0.037 |
3.67 ± 0.012 |
4.79 ± 0.031 |
7 |
32(−α) |
97(0) |
5.57 ± 0.009 |
2.70 ± 0.002 |
2.35 ± 0.002 |
14.77 ± 0.023 |
6.96 ± 0.033 |
6.34 ± 0.035 |
8 |
60(0) |
97(0) |
6.11 ± 0.017 |
3.06 ± 0.004 |
2.15 ± 0.001 |
15.86 ± 0.021 |
7.84 ± 0.013 |
6.18 ± 0.042 |
9 |
60(0) |
66(−α) |
5.59 ± 0.014 |
2.05 ± 0.002 |
1.44 ± 0.003 |
15.30 ± 0.065 |
4.81 ± 0.036 |
4.08 ± 0.028 |
10 |
40(−1) |
120(+1) |
6.06 ± 0.009 |
3.36 ± 0.005 |
2.85 ± 0.006 |
15.61 ± 0.053 |
9.07 ± 0.056 |
7.54 ± 0.017 |
11 |
60(0) |
129(+α) |
6.16 ± 0.016 |
3.77 ± 0.004 |
3.54 ± 0.011 |
16.20 ± 0.086 |
9.42 ± 0.045 |
9.07 ± 0.051 |
12 |
60(0) |
97(0) |
5.80 ± 0.004 |
3.07 ± 0.007 |
2.17 ± 0.006 |
15.22 ± 0.056 |
7.17 ± 0.026 |
5.82 ± 0.026 |
13 |
88(+α) |
97(0) |
4.14 ± 0.003 |
1.97 ± 0.006 |
2.39 ± 0.003 |
13.86 ± 0.036 |
4.98 ± 0.015 |
6.29 ± 0.036 |
The automated PLE cycle was as follows: the cell containing the sample was pre-filled with the extraction solvent, pressurized (103.42 bar), and then heated for 5 min followed by a static period of 5 min. The sample was extracted with methanol concentration and temperature as specified in the design during this 5 min. Then, the cell was rinsed with fresh extraction solvent (60% of the extraction cell volume) and purged with a flow of nitrogen (10.34 bar during 90 s). Extracts (34 mL) were collected into 60 mL glass vials. The solvent used was previously degassed with nitrogen to avoid the oxidation of the analytes under the operating conditions. The extracts were stored at −20 °C in darkness until analysis. The experiment was performed in two batches which included three replications in each sample. Prior to HPLC and in vitro antioxidant activity analysis the extract was filtered through a 0.45 μm PTFE filters (Millipore, USA).
2.3. Conventional solid/liquid extraction
Solid/liquid extractions were carried out according to the method of Shan et al.2 with slight modifications. Briefly, dried and ground samples (0.5 g) were homogenized for 1 min at 24,000 rpm using an Ultra-Turrax T-25 Tissue homogenizer (Janke & Kunkel, IKA®-Labortechnik, Saufen, Germany) in 25 mL of 80% methanol at room temperature (∼23 °C). The homogenized sample suspension was shaken overnight with a V400 Multitude Vortexer (Alpha laboratories, North York, Canada) at 1,500 rpm at room temperature (≅ 25 °C). The sample suspension was then centrifuged for 15 min at 2,000 g (MSE Mistral 3000i, Sanyo Gallenkamp, Leicestershire, UK) and immediately filtered through 0.22 μm polytetrafluoroethylene (PTFE) filters. The extracts were kept at −20 °C until subsequent analysis. The experiment was performed in two batches which included three replications in each sample.
2.4. Determination of total phenolic content
The total phenolic content was determined using Folin-Ciocalteu Reagent (FCR) as described by Singelton et al.28 The experiment was performed in two batches which included three replications in each for both samples and standard. Methanolic gallic acid solutions (10–400 mg/L) were used as standards. In each replicate, 100 μL of the appropriately diluted sample extract, 100 μL methanol, 100 μL FCR and finally 700 μL 20% Na2CO3 were added together and vortexed. The mixture was incubated for 20 min in the dark at room temperature. After incubation the mixture was centrifuged at 13,000 rpm for 3 min. The absorbance of the supernatant was measured at 735 nm by spectrophotometer. The total phenolic content was expressed as gallic acid equivalent [g GAE/100 g dry weight (DW)] of the sample.
2.5. Ferric ion reducing antioxidant power (FRAP) assay
The FRAP assay was carried out as described by Stratil et al.29 with slight modifications. The FRAP reagent was: 38 mM sodium acetate anhydrous in distilled water at pH 3.6, 20 mM FeCl3·6H2O in distilled water and 10 mM 2,4,6-tri(2-pyridyl)-s-triazine (TPTZ) in 40 mM HCl in a ratio of 10
:
1
:
1. This reagent was prepared before each experiment. To each sample, 100 μL of appropriately diluted sample extract and 900 μL of FRAP reagent was added, mixed and was incubated at 37 °C for 40 min in the dark. In the case of the blank, 100 μL of methanol was added to 900 μL of FRAP reagent. The absorbance of the resulting solution was measured at 593 nm by spectrophotometer. Trolox (6-hydroxy-2,5,7,8-tetramethylchroman-2-carboxylic acid), which is a synthetic antioxidant at concentrations ranging from 0.1 mM to 0.4 mM, was used as a reference antioxidant standard. FRAP values were expressed as g Trolox/100 g DW of the sample.
2.6. Determination of melanoidins
To estimate the degree of formation of browning compounds such as melanoidins the absorbance at 420 nm was measured; this wavelength has previously been used to detect browning defects in fruit juices.30 The extracts were diluted four times before measuring the absorbance as some of the extracts had very high absorbance (>2.50).
2.7. HPLC analysis of the extracts
High performance liquid chromatography (HPLC) of the filtered sample extracts were carried out according to the method of Tsao and Yang.31 The chromatographic system (Shimadzu-Model no SPD-M10A VP, Mason Technology, Dublin 8, Ireland) consisted of a pump, a vacuum degasser, a Diode-Array Detector and was controlled through EZ Start 7.3 software (Shimadzu) at 37 °C. An Agilent C18 column (15 cm × 4.6 cm, 5 μm, Agilent Technologies., USA) was utilized with a binary mobile phase of 6% acetic acid in 2 mM sodium acetate (final pH 2.55, v/v, solvent A) and acetonitrile (solvent B). Solvent A was prepared first by making 2 mM sodium acetate water solution, which was then mixed with acetic acid at a ratio of 94
:
6 by volume. All solvents were filtered through a 0.45 μm membrane filter prior to analysis. The flow rate was kept constant at 1.0 mL/min for a total run time of 80 min. The following gradient program was carried out: 0–15% B in 45 min, 15–30% B in 15 min, 30–50% B in 5 min, 50–100% B in 5 min and 100 − 5% B in 10 min. The sample injection volume was 10 μL. The standards of rosmarinic acid and caffeic acid were dissolved in methanol for quantification purpose (100 μg/mL). The detection wavelength of 280 nm was used for the detection of gallic acid. Rosmarinic acid and caffeic acid were detected at 320 nm. Identification of the compounds was achieved by comparing their retention times and UV-Vis spectra with those of authenticated rosmarinic acid standard by using the inline DAD with a 3D feature. Results are expressed as mean values of three assays for each replicated experiment.
2.8. Statistical analysis
Optimal PLE conditions were determined by RSM which was performed using the Design Expert Version 7.1.3 software (Stat-Ease, Inc., Minneapolis, MN). A CCD was used to investigate the effects of two independent variables, solvent concentration and extraction temperature, on the dependent variables, TP and FRAP. The data obtained from the CCD design was fitted with a second order polynomial equation. The equation was as follows: | Y = β0 + ∑2i=1βiXi + ∑2i=1βiiX2i + ∑i∑j=i + 1βijXiXj | (1) |
where Y is the predicted response; β0 is a constant; βi is the linear coefficient; βii is the quadratic coefficient, βij is the interaction coefficient; and Xi and Xj are independent variables. The adequacy of the model was determined by evaluating the lack of fit, coefficient of regression (R2) and the Fisher test value (F-value) obtained from the analysis of variance (ANOVA). Statistical significance of the model and model variables was determined at the 5% probability level (p < 0.05). The software uses the quadratic model equation shown above to build response surfaces. Three-dimensional response surface plots were generated by maintaining one response variable at its optimal level and plotting that against two factors (independent variables). The input range of the selected variables was determined by preliminary RSM experiments. This was set as 40% (coded as −1) to 80% (coded as +1) for methanol concentration and 75 °C (coded as −1) to 120 °C (coded as +1). The two independent variables were coded at five levels (−α, −1, 0, 1, α), which resulted in an experimental design of 13 experimental points, including five central points. The actual values of the factors with their coded terms for the experimental designs are given in Table 1. Statistical analyses were carried out on the basis of actual values.
2.9. Model validation
The predictive performance of the developed models describing the combined effect methanol concentration (X1) and temperature (X2) on independent variables (FRAP, TP) of sage, basil and thyme were validated in a separate set of selected conditions. These assessments were carried out by calculating the model performance indices: accuracy factor (AF, eqn (2a)) and bias factor (BF, eqn (2b)).32 | 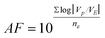 | (2a) |
| 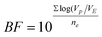 | (2b) |
The criterion used to characterize the fitting efficiency of the data to the model was the multiple correlation coefficients (R2) and their average mean deviation (E, eqn (2a)).
| 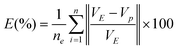 | (2c) |
where, n
e is the number of
experimental data, V
E is the experimental value and V
P is the predicted value.
3. Results and discussion
3.1. Optimization of PLE conditions from sage, basil and thyme with respect to total phenols and FRAP values
Fig. 1 illustrates response surface plots showing the effect of methanol concentration and temperature on TP and FRAP values of sage, basil and thyme. Using PLE, the optimal temperature for obtaining extracts with high antioxidant activity was 129 °C in all the spices tested. In fact, temperature was found to be the dominant factor in maximising the phenolic content and antioxidant activity as measured by FRAP values of the extracts. This result was in agreement with the findings of Zgorka33 who reported that concentrations of isoflavone obtained from Clover (Trifolium L.) using PLE increased with increasing the temperature from 75 °C to 125 °C with no evidence of thermal degradation of the target analytes. Several other studies using PLE also reported the similar effect of temperature on extraction yield of phenolics from plant materials.34,35 The results were expected since an increase in temperature enhances the solubility of many compounds. High temperatures might also have increased the diffusion rate of the compounds resulting in antioxidant compounds being extracted at much higher rates. Interestingly PLE offers a unique possibility of using high temperature at very high pressure (1500 psi) while preventing degradation of the extracted compounds.33 This is because high pressure generally increases the stability of covalent bonds within bio-molecules. In the present study, evidence of thermal degradation could only be detected at temperatures of 150 °C or above. In terms of polyphenol content the spices were ranked in the following descending order sage > basil > thyme.
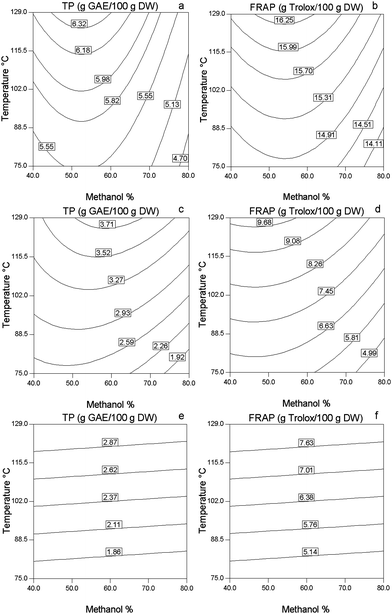 |
| Fig. 1 Contour plots of PLE extracts of sage (a,b), basil (c,d) and thyme (e,f) showing the effect of methanol concentration and temperature on TP (g GAE/100 g DW) and FRAP (g Trolox/100 g DW) values. | |
A methanol concentration of 50–60% showed better performance with regard to antioxidant activity and total phenolic content of extracts from sage. Thus RSM assisted optimization demonstrated that optimum PLE conditions for sage were 58% methanol coupled with a temperature of 129 °C (Fig. 1a,b and Table 2). Similar results were reported by Akowuah et al.36 using conventional solid/liquid extraction. They found 50% aqueous methanol extract of Orthosiphon stamineus had higher rosmarinic acid content and antioxidant capacity as measured using DPPH scavenging assay than other methanol concentrations (0% and 100%) tested. In the present study both factors, temperature and methanol concentration in the PLE had a significant (p < 0.05) linear effect on the total phenolic content of sage extracts (Fig. 2a,b). Moreover, methanol concentration showed a significant (p < 0.05) quadratic effect on total phenols of sage PLE extracts. The second order polynomial equations presented in Table 2 show the relationship between the factors and response variables. Only the significant model terms were included in the equations. In the case of FRAP values of sage PLE extracts, only temperature had a significant (p < 0.05) effect at a linear level, while methanol concentration showed significant (p < 0.05) quadratic effect. Optimal sage PLE extracts also had a significantly (p < 0.05) higher TP (14%) and FRAP values (20%) as compared to the conventional solid/liquid extracts (Table 3). ANOVA analysis of the designs showed that the models of TP and FRAP values of sage were significant (p < 0.05). The regression coefficient, as obtained from ANOVA, was higher for TP values (R2 = 0.94) than that of FRAP values (R2 = 0.65). It is stipulated that these models could be used for prediction of the data. In fact, the predicted TP and FRAP values of sage correlated strongly with actual values (Pearson's correlation coefficient = 0.971 for TP and 0.882 for FRAP).
Table 2 Second order polynomial equations and regression coefficients of the response variables of pressurized liquid extractions at a temperature range of 66 to 129 °C with X1 = Methanol concentration (%), X2 = Temperature (°C), Y = Response variables (TP or FRAP)
Spice name |
Response variables |
Second order polynomial equation |
R2 |
Optimal PLE (% methanol/°C) |
Sage |
TP (g GAE/100 g DW) |
Y = 1.17639 + 0.132731X1 + 0.0142696X2 − 0.00130094X12 |
0.943 |
58/129 |
FRAP (g Trolox /100 g DW) |
Y = 6.71513 + 0.197932X1 + 0.0282219X2 − 0.00164943X12 |
0.651 |
Basil |
TP (g GAE/100 g DW) |
Y = −1.19361 + 0.0577599X1 + 0.0328361X2 + 0.000383333X1X2 − 0.000904373X12 − 0.000146668X22 |
0.999 |
60/129 |
FRAP (g Trolox /100 g DW) |
Y = −2.73633 + 0.170073X1 + 0.0673262X2 − 0.00183409X12 |
0.942 |
Thyme |
TP (g GAE/100 g DW) |
Y = −0.316668 + 0.0263058 X2 |
0.819 |
33/129 |
FRAP (g Trolox /100 g DW) |
Y = −0.2506 + 0.065017 X2 |
0.890 |
Name of the spice |
Treatment |
Gallic acid (mg/g DW) |
Melanoidins (Absorbance at 420 nm) |
TP (g GAE/100 g DW) |
FRAP (g Trolox/100 g DW) |
Sage |
Optimal |
1.10 ± 0.002 |
0.243 ± 0.002 |
6.92 ± 0.005 |
17.42 ± 0.045 |
150 °C |
1.63 ± 0.006 |
0.552 ± 0.002 |
7.37 ± 0.009 |
18.35 ± 0.063 |
175 °C |
2.52 ± 0.005 |
0.583 ± 0.002 |
7.68 ± 0.016 |
19.12 ± 0.087 |
200 °C |
2.65 ± 0.010 |
0.708 ± 0.004 |
7.77 ± 0.037 |
20.34 ± 0.104 |
Solid/liquid |
1.07 ± 0.002 |
0.316 ± 0.001 |
6.08 ± 0.015 |
14.54 ± 0.063 |
Basil |
Optimal |
0.76 ± 0.001 |
0.428 ± 0.002 |
4.12 ± 0.011 |
9.76 ± 0.042 |
150 °C |
1.14 ± 0.003 |
0.656 ± 0.003 |
4.62 ± 0.008 |
10.51 ± 0.062 |
175 °C |
1.41 ± 0.002 |
0.750 ± 0.001 |
4.83 ± 0.005 |
11.72 ± 0.035 |
200 °C |
1.57 ± 0.011 |
0.822 ± 0.003 |
5.41 ± 0.014 |
13.15 ± 0.043 |
Solid/liquid |
0.61 ± 0.001 |
0.680 ± 0.001 |
2.18 ± 0.004 |
5.84 ± 0.009 |
Thyme |
Optimal |
0.64 ± 0.002 |
0.521 ± 0.002 |
3.32 ± 0.012 |
9.05 ± 0.013 |
150 °C |
1.13 ± 0.002 |
0.682 ± 0.002 |
3.74 ± 0.007 |
9.94 ± 0.046 |
175 °C |
1.37 ± 0.004 |
0.713 ± 0.003 |
4.18 ± 0.006 |
11.74 ± 0.074 |
200 °C |
1.41 ± 0.006 |
0.813 ± 0.002 |
4.79 ± 0.009 |
12.25 ± 0.037 |
Solid/liquid |
0.52 ± 0.004 |
0.375 ± 0.001 |
2.46 ± 0.003 |
8.20 ± 0.024 |
![Standardised pareto chart of sage (a,b), basil (c,d) and thyme (e,f) showing the effect of different factor terms [A: Methanol concentration, B: Temperature, AA: (Methanol concentration)2, BB: (Temperature)2 and AB: Methanol concentration X Temperature] on TP and FRAP values. Bars exceeding the vertical line on the graph indicate that the corresponding factor terms are significant (p < 0.05).](/image/article/2010/FO/c0fo00021c/c0fo00021c-f2.gif) |
| Fig. 2 Standardised pareto chart of sage (a,b), basil (c,d) and thyme (e,f) showing the effect of different factor terms [A: Methanol concentration, B: Temperature, AA: (Methanol concentration)2, BB: (Temperature)2 and AB: Methanol concentration X Temperature] on TP and FRAP values. Bars exceeding the vertical line on the graph indicate that the corresponding factor terms are significant (p < 0.05). | |
Extracts from basil were optimally extracted at 60% methanol and 129 °C using PLE (Fig. 1c,d and Table 2). Temperature had a greater effect on the TP and FRAP values of the extracts than methanol concentration (Fig. 2c, d). At optimal methanol concentration (60%), when the temperature was increased from 66 °C, lowest temperature used, to 129 °C, highest temperature used, the total phenolic content was increased from 2.05 g GAE/100 g DW to 3.77 g GAE/100 g DW which corresponds to an 83.9% increase. Over the same temperature range, FRAP values were increased by 96% from 4.81 g Trolox/100 g DW to 9.42 g Trolox/100 g DW. Pressurized liquid extracts of basil had almost twice TP and FRAP values than solid/liquid extracts (Table 3). The models for TP and FRAP values were significant (0<0.001) with high regression coefficients of 0.999 (TP) and 0.942 (FRAP).
The models also showed very high F-values (3694.20 for TP and 49.29 for FRAP) and a non-significant (p > 0.05) lack of fit. The predicted values for TP and FRAP showed high correlation (Pearson's correlation coefficient, r = 0.999 for TP and 0.970 for FRAP) when compared with actual experimental data.
Total phenolic content and FRAP values of thyme pressurized liquid extracts exhibited a sharp increase with the increase of temperature (Fig. 1e,f). For example TP and FRAP values increased by 46% and 51%, respectively, when the temperature was increased from 75 °C to 120 °C at 40% methanol concentration. Optimal PLE conditions for thyme were 33% methanol and a temperature of 129 °C. Similar to the other tested Lamiaceae spices, thyme showed high regression coefficients (Table 2). Of the three spices, thyme was optimally extracted with the lowest ratio of methanol to water (2
:
1), indicating that thyme may contain more hydrophilic antioxidant compounds than the other two spices examined.
For thyme, methanol concentration had no significant (p < 0.05) effect on the level of total phenolic content or FRAP activity. Temperature was the only factor which had significant effect on TP and FRAP of the thyme extracts at a linear level (Fig. 2e, f). As for sage and basil, FRAP and TP values of optimally extracted thyme PLE were significantly (p < 0.05) higher than solid/liquid extracts (Table 3).
The models for TP and FRAP in thyme were also very significant (p < 0.0001) and highly predictive. The regression coefficients for TP and FRAP values were 0.819 and 0.890, respectively (Table 2), and the lack of fit was non-significant (p > 0.05). The experimental TP and FRAP values showed a high degree of similarity with the predicted values from the models with Pearson's correlation coefficients (r) of 0.951 and 0.945 for TP and FRAP respectively. This study also validated the developed model, using a distinct set of data obtained from additional test runs, which is recommended by Carrasco et al.37 The experimental data were plotted against the predicted values from eqn (3) for polynomial models. Predicted values of FRAP and TP obtained using model equations were in good agreement with the experimental values. The experimental and predicted values were closely correlated, as demonstrated by regression coefficient (R2). The predicted values were within the 95% confidence interval.
| Yi = β0 + β1X1 + β2X2 + β11X21 + β22X22 + β12 (X1X2) | (3) |
where Yi is predicted response for
FRAP and TP content.
The applicability of the models was also quantitatively evaluated by comparing the bias and accuracy factors for each of the parameter (eqn (2a) and (2b)). In most cases, as shown in (Table 4), the accuracy factor (AF) values for the predicted model were close to 1.00. The bias factor (BF) values for the predicted models were also close to 1.00, ranging from (0.96–1.02) for all the parameters studied. These results demonstrate that there was a good agreement between predicted and observed values. Ross et al.38 reported that predictive models should ideally have an AF = 1.00, indicating a perfect model fit where the predicted and actual response values are equal. However, Ross et al.38 and Carrasco et al.37 reported that the AF of a fitted model increases by 0.10–0.15 units for each predictive variable in the model. Since the current model forecasted a response from two predictive variables (temperature, solvent concentration), it was expected to have AF values ranging from 1.20 to 1.3038 or an equivalent percentage error range of 20–30%. Despite some variations, results obtained from the validated predicted model and actual experimental values showed that the established models reliably predicted FRAP and TP content. The predicted values were in close agreement with experimental values (Table 4) and were found to be not significantly different at p > 0.05 using a paired t-test. In addition variations between the predicted and experimental values obtained for total antioxidant activity by FRAP assay and TP content were within acceptable error range as depicted by average mean deviation (E%, Table 4); therefore, the predictive performance of the established model may be considered acceptable.
Table 4 Bias factor (BF), accuracy factor (AF), actual, predicted and average mean deviation (E%) for the response (TP and FRAP) studied
Spice |
Solvent Conc/temp |
TP |
FRAP |
TP |
FRAP |
BF |
AF |
BF |
AF |
Predicted |
Observed |
E% |
Predicted |
Observed |
E% |
Sage |
58/129 |
0.96 |
1.04 |
0.98 |
1.02 |
6.18 |
6.92 |
3.56 |
16.35 |
17.42 |
2.04 |
80/100 |
1.01 |
1.01 |
0.99 |
1.01 |
4.89 |
4.75 |
1.02 |
14.81 |
15.20 |
0.84 |
40/70 |
0.99 |
1.01 |
1.00 |
1.00 |
5.40 |
5.51 |
0.64 |
13.96 |
14.15 |
0.42 |
Basil |
60/129 |
0.97 |
1.03 |
0.99 |
1.01 |
3.77 |
4.12 |
2.83 |
9.42 |
9.76 |
1.16 |
80/129 |
0.98 |
1.02 |
0.98 |
1.02 |
2.52 |
2.67 |
1.83 |
5.86 |
6.15 |
1.55 |
40/70 |
1.03 |
1.03 |
1.02 |
1.06 |
2.32 |
2.10 |
3.53 |
5.84 |
5.52 |
1.96 |
Thyme |
33/129 |
1.02 |
1.02 |
1.00 |
1.00 |
3.54 |
3.32 |
2.20 |
9.08 |
9.05 |
0.11 |
80/100 |
0.97 |
1.03 |
0.99 |
1.01 |
2.32 |
2.55 |
3.08 |
6.25 |
6.46 |
1.07 |
40/70 |
1.01 |
1.01 |
0.99 |
1.03 |
1.52 |
1.48 |
1.00 |
4.30 |
4.45 |
1.11 |
3.2. Effect of elevated temperatures (>129 °C) on rosmarinic acid and other phenolic acids in relation to antioxidant activity of pressurized liquid extracts of Lamiaceae spices
Pressurized liquid extracts obtained using optimized parameters had significantly (p < 0.05) higher amounts of rosmarinic acid than the conventional solid/liquid extracts (Table 5) in the samples tested. Since all three spices used showed the best performance at 129 °C, the highest temperature used in the design, extraction temperatures of 150 °C, 175 °C and 200 °C were tested with the respective optimal methanol concentrations. For all the increased temperatures, a sharp decrease (range: 38–72%) in the levels of rosmarinic acid in the extracts were observed as shown in Fig. 3 and Table 5. However, total phenolic content and FRAP values increased with higher temperatures as shown in Table 3. One possible explanation for this behaviour is the degradation of rosmarinic acid into compound/s which had higher antioxidant activity than the parent compound. Other authors have also reported the loss of rosmarinic acid occurred at relatively low temperatures in conventional solid/liquid extraction (105 °C).39 In the present study, decreases in rosmarinic acid levels at temperatures of 150 °C or higher, were accompanied by increases in the caffeic acid content of the extracts with the highest level being reached at 200 °C (Fig. 3 and Table 5). Since rosmarinic acid is an ester of caffeic acid and 3, 4-dihydroxyphenyl lactic acid, caffeic acid may be one of the degradation products. The antioxidant activity as measured by FRAP assay of pure caffeic acid (423.72 g Trolox/100 g DW) was higher than that of rosmarinic acid (406.30 g Trolox/100 g DW). The other possible degradation product 3,4-dihydroxyphenyl lactic acid was also reported to have high antioxidant activity.40 Moreover, the quantity of gallic acid which is a powerful antioxidant (346.21 g Trolox/100g DW as measured by FRAP assay) in the extracts obtained at 150 °C or above was significantly higher than that of the extracts obtained at 129 °C (Table 3). In fact, the extracts of sage obtained at 200 °C contained 2.4 times the gallic acid concentration than the extracts obtained at 129 °C. Higher concentration of gallic acid, caffeic acid and 3,4-dihydroxyphenyl lactic acid might explain the increase of antioxidant capacity at temperatures of 150 °C or above. Quantity of other phenolics such as luteolin-7-O-glucoside, apigenin-7-O-glucoside, carnosic acid of PLE extracts of sage, basil and thyme obtained at 150 °C or above did not show significant (p > 0.05) change in comparison to optimal PLE extracts. In addition, the present study observed the formation of Maillard reaction products (melanoidins) at temperatures of 150 °C or above. The absorbance at 420 nm of the PLE extracts obtained at 150 °C or above was significantly higher than that of optimal and solid/liquid extracts (Table 3). The increase in antioxidant activity of the extracts obtained at 150 °C or above may be related to the production of melanoidins, reported to have antioxidant capacity.41 However, since the Maillard reaction has been reported to produce potentially harmful compounds42 and changed the natural phenolic profile of the extracts, extractions carried out at temperatures in the range of 150–200 °C are not recommended. Therefore, PLE at 129 °C with respective methanol concentration was determined to be the optimum for extracting antioxidant compounds from the studied spices.
Name of the spice |
Treatment |
Rosmarinic acid (mg/g DW) |
Significancea |
Caffeic acid (mg/g DW) |
Significancea |
3,4-Dihydroxyphenyl lactic acid (mg/g DW) |
Significancea |
Same letters in each sample indicate no significant (p ≥ 0.05) difference.
|
Sage |
Optimal |
15.881 |
D |
0.429 |
B |
Not detected |
A |
150 °C |
12.941 |
B |
0.550 |
C |
0.766 |
B |
175 °C |
10.361 |
A |
0.575 |
C |
1.065 |
C |
200 °C |
10.440 |
A |
0.631 |
D |
1.352 |
D |
Solid/liquid |
14.975 |
C |
0.126 |
A |
Not detected |
A |
Basil |
Optimal |
4.255 |
D |
0.601 |
B |
0.044 |
A |
150 °C |
3.755 |
C |
0.897 |
C |
0.051 |
A |
175 °C |
2.464 |
B |
1.174 |
D |
1.041 |
B |
200 °C |
2.517 |
A |
1.459 |
E |
1.214 |
C |
Solid/liquid |
3.410 |
B |
0.068 |
A |
0.041 |
A |
Thyme |
Optimal |
4.401 |
C |
0.207 |
B |
0.071 |
A |
150 °C |
3.224 |
B |
0.253 |
C |
0.181 |
B |
175 °C |
1.462 |
A |
0.288 |
D |
0.376 |
C |
200 °C |
1.233 |
A |
0.302 |
D |
0.565 |
D |
Solid/liquid |
3.36 |
B |
0.098 |
A |
0.032 |
A |
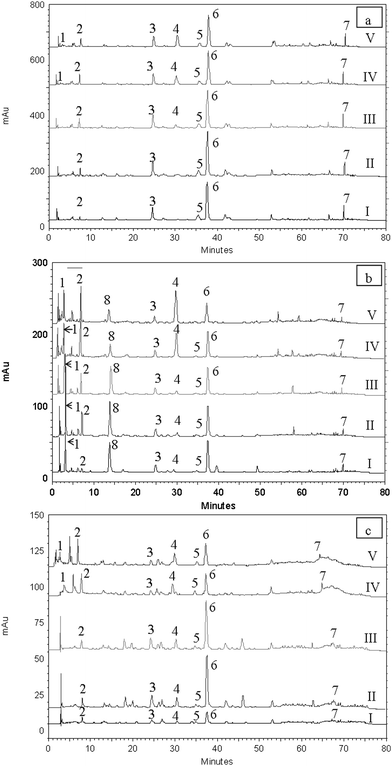 |
| Fig. 3 HPLC chromatograms of PLE extracts obtained at 75 °C (I), 129 °C (II), 150 °C (III), 175 °C (IV) and 200 °C (V) using respective optimal solvent concentrations in sage (a), basil (b) and thyme (c) showing the changes in peaks of different polyphenols (1 = gallic acid, 2 = caffeic acid, 3 = luteolin-7-O-glucoside, 4 = 3,4-dihydroxyphenyl lactic acid, 5 = apigenin-7-O-glucoside, 6 = rosmarinic acid, 7 = carnosic acid and 8 = ferulic acid). | |
4. Conclusion
The present study demonstrated that total phenolic content and FRAP values of the PLE extracts of the spices tested increased significantly with the increase of temperature. PLE temperature of 129 °C performed the best. Methanol concentration showed significant effect on the parameters in sage and basil. The optimal methanol concentration for sage and basil was 58% and 60%, respectively. Results of the model validation showed that the developed model had acceptable predictive performance, as assessed by mathematical and graphical model performance indices. The coefficient of determinations (R2) for predicted FRAP and TP content showed good correlation with the experimental data at the 95% confidence level. Thermal degradation of rosmarinic acid was only detected at temperatures higher than 150 °C. Optimal PLE extracts of all the spices tested showed significantly (p < 0.05) higher total phenol content and FRAP values than the conventional solid/liquid extracts. Rosmarinic acid content in optimal PLE extracts was also higher than the solid/liquid extracts. Therefore optimised PLE could be used as a high throughput extraction system for spice phenolics.
Acknowledgements
This work was supported by the ABBEST scholarship programme of Dublin Institute of Technology, Dublin, Ireland. The authors are thankful to Teagasc, Ashtown Food Research Centre, Ashtown, Dublin for providing laboratory facilities. We would like to thank AllinAll Ingredients Ltd, Dublin 12 for providing spice samples and Hilde Henny Wijngaard for her advice in RSM.
References
- M. B. Hossain, N. P. Brunton, C. Barry-Ryan, A. B. Martin-Diana and M. Wilkinson, Rasayan J. Chem., 2008, 1, 751–756 Search PubMed.
- B. Shan, Y. Z. Cai, M. Sun and H. Corke, J. Agric. Food Chem., 2005, 53, 7749–7759 CrossRef CAS.
- E. K. Perry, A. T. Pickering, W. W. Wang, P. J. Houghton and N. S. L. Perry, J. Pharm. Pharmacol., 1999, 51, 527–534 CrossRef CAS.
-
K. Hirasa, M. Takemasa, Spice science and technology, Dekker Inc, New York, 1998 Search PubMed.
- H. L. Madsen and G. Bertelsen, Trends Food Sci. Technol., 1995, 6, 271–277 CrossRef CAS.
- M. Wang, J. Li, M. Rangarajan, Y. Shao, E. J. LaVoie, T. C. Huang and C. T. Ho, J. Agric. Food Chem., 1998, 46, 4869–4873 CrossRef CAS.
- M. A. Klem, M. G. Nair, G. M. Sraassburg and D. L. Dewitt, Phytomedicine, 2000, 7, 7–13.
- T. K. Maity, S. C. Mandal, B. P. Saha and M. Pal, Phytother. Res., 2000, 14, 120–121 CrossRef CAS.
- J. Prakash and S. K. Gupta, J. Ethnopharmacol., 2000, 72, 29–34 CrossRef CAS.
- T. Beric, B. Nikolic, J. Stanojevic, B. Vukovic-Gacic and Knezevic-Vukcevic, Food Chem. Toxicol., 2008, 46, 724–732 CrossRef CAS.
- R. Baranauskiene, P. R. Venskutonis, P. Viskelis and E. Dambrauskiene, J. Agric. Food Chem., 2003, 51, 7751–7758 CrossRef CAS.
- W. Zheng and S. Y. Wang, J. Agric. Food Chem., 2001, 49, 5165–5170 CrossRef CAS.
- M. Suhaj, J. Food Compos. Anal., 2006, 19, 531–537 CrossRef CAS.
- A. Beyer and M. Biziuk, Food Chem., 2008, 108, 669–680 CrossRef CAS.
- X. Lou, H. Janssen and C. A. Cramers, Anal. Chem., 1997, 69, 1598–1603 CrossRef CAS.
- J. D. Denery, K. Dragull, C. S. Tang and Q. X. Li, Anal. Chim. Acta, 2004, 501, 175–181 CrossRef CAS.
- W. Siriwong, K. Thirakhupt, D. Sitticharoenchai, J. Rohitrattana, P. Thongkongowm, M. Borjan and M. Robson, Ecol. Indic., 2009, 9, 878–882 CrossRef CAS.
- T. Okuda, N. Yamashita, H. Tanaka, H. Matsukawa and K. Tanabe, Environ. Int., 2009, 35, 815–820 CrossRef CAS.
- E. Ibanez, A. Kubatova, F. J. Senorans, S. Cavero, G. Reglero and S. B. Hawthorne, J. Agric. Food Chem., 2002, 51, 375–382.
- A. L. Dawidowicz, E. Rado, D. Wianowska, M. Mardarowicz and J. Gawdzik, Talanta, 2008, 76, 878–884 CrossRef CAS.
- Y. Yang, B. Kayan, N. Bozer, B. Pate, C. Baker and A. M. Gizir, J. Chromatogr., A, 2007, 1152, 262–267 CrossRef CAS.
- M. Ollanketo, A. Peltoketo, K. Hartonen, R. Hiltunen and L. M-Riekkola, Eur. Food Res. Technol., 2002, 215, 158–163 CrossRef CAS.
- M. Herrero, L. Jaime, P. J. Martin-Alvarez, A. Cifuentes and E. Ibanez, J. Agric. Food Chem., 2006, 54, 5597–5603 CrossRef CAS.
- Z. Y. Ju and L. R. Howard, J. Agric. Food Chem., 2003, 51, 5207–5213 CrossRef CAS.
- L. dos Santos Freitas, R. A. Jacques, M. F. Richter, A. L. D. Silva and E. B. Caramão, J. Chromatogr., A, 2008, 1200, 80–83 CrossRef CAS.
- S. K. Ghodke, L. Ananthanarayan and L. Rodrigues, Food Chem., 2009, 112, 1010–1015 CrossRef CAS.
- W. Huang, Z. Li, H. Niu, D. Li and J. Zhang, J. Food Eng., 2008, 89, 298–302 CrossRef CAS.
- V. L. Singelton, R. Orthofer and R. R. Lamuela-Raventos, Methods Enzymol., 1999, 299, 152–178.
- P. Stratil, B. Klejdus and V. Kuban, J. Agric. Food Chem., 2006, 54, 607–616 CrossRef CAS.
- A. Ibarz, J. Pagán, R. Panadés and S. Garza, J. Food Eng., 2005, 69, 155–160 CrossRef.
- R. Tsao and R. Yang, J. Chromatogr., A, 2003, 1018, 29–40 CrossRef CAS.
- A. Patras, B. K. Tiwari, N. P. Brunton and F. Butler, Food Chem., 2009, 114, 484–491 CrossRef CAS.
- G. Zgórka, Talanta, 2009, 79, 46–53 CrossRef CAS.
- A. H. Zaibunnisa, S. Norashikin, S. Mamot and H. Osman, Lebensm.-Wiss. Technol. - Food Sci. Technol., 2009, 42, 233–238 Search PubMed.
- S. Santoyo, I. Rodríguez-Meizoso, A. Cifuentes, L. Jaime, G. García-Blairsy Reina, F. J. Señorans and E. Ibáñez, Lebensm.-Wiss. Technol. - Food Sci. Technol., 2009, 42, 1213–1218 Search PubMed.
- G. A. Akowuah, Z. Ismail, I. Norhayati and A. Sadikun, Food Chem., 2005, 93, 311–317 CrossRef CAS.
- E. Carrasco, R. Garcia-Gimeno, R. Seselovsky, A. Valero, F. Perez and G. Zurera, Food Sci. Technol. Int., 2006, 12, 47–56 Search PubMed.
- T. Ross, P. Dalgaard and S. Tienungoon, Int. J. Food Microbiol., 2000, 62, 231–245 CrossRef CAS.
- L. Almela, B. Sánchez-Muñoz, J. A. Fernández-López, M. J. Roca and V. Rabe, J. Chromatogr., A, 2006, 1120, 221–229 CrossRef CAS.
- Y. Lu and L. Y. Foo, Phytochemistry, 2002, 59, 117–140 CrossRef CAS.
- F. J. Morales and M. B. Babbel, J. Agric. Food Chem., 2002, 50, 2788–2792 CrossRef CAS.
-
P. Arvidsson, M. A. J. S. Van Boekel, K. Skog, M. Jagerstad, Formation of mutagenic Maillard reaction products. In J. O'Brien, H. E. Nursten, M. J. C. Crabbe, & J. M. Ames (ed.), The Maillard reaction in foods and medicine. Cambridge, UK: Royal Society of Chemistry. 1998, pp. 219–224 Search PubMed.
|
This journal is © The Royal Society of Chemistry 2010 |
Click here to see how this site uses Cookies. View our privacy policy here.