Adsorptive selenite removal from water using a nano-hydrated ferric oxides (HFOs)/polymer hybrid adsorbent†
Received
10th July 2009
, Accepted 28th August 2009
First published on
25th September 2009
Abstract
Selenite (SeO32−) is an oxyanion of environmental significance due to its toxicity when taken in excess. In the present study, a hybrid adsorbent (HFO-201) was prepared by irreversibly impregnating hydrated ferric oxide (HFO) nanoparticles within a commercial available anion-exchange resin (D-201), and its adsorption towards selenite from water was investigated in batch and column tests. HFO-201 exhibited improved sorption selectivity toward selenite as compared to the polymeric anion exchanger D-201. Two possible adsorption interactions were responsible for selenite removal by HFO-201, the electrostatic interaction from the ammonium groups bound to the D-201 matrix, and the formation of inner-sphere complexes between the loaded HFO nanoparticles and selenite. In a wide pH range (i.e., 3–8), increasing solution pH was found to result in a decrease of selenite removal on HFO-201. Adsorption isotherms fit the Freundlich model well, and selenite adsorption increased with increasing ambient temperature, indicating its endothermic nature. Column adsorption tests suggested that satisfactory removal of selenite from 2 mg/L to less than 0.01 mg/L could be achieved by HFO-201 even in the presence of the commonly encountered anionic competition at greater concentration, with the treatment capacity of ∼1200 bed volume (BV) per run, while that for D-201 was only less than 30 BV under otherwise identical conditions. Furthermore, the exhausted HFO-201 was amenable to efficient in situ regeneration with a binary NaOH–NaCl solution.
Environmental impact
A new hybrid adsorbent HFO-201 was fabricated for enhanced removal of selenite from aqueous media by impregnating hydrated ferric oxide (HFO) nanoparticles within the inner pores of a macroporous polymeric anion exchanger D-201. HFO-201 greatly outperformed D-201 in selenite removal from the background of competing anions at much greater levels, with an effective treatment volume ∼40 times higher than the latter one. Moreover, selenite removal by HFO-201 results in its conspicuous decrease from 2.0 mg/L to below 0.010 mg/L (the drinking water standard recommended by WHO), and the exhausted HFO-201 is amenable to efficient regeneration for multiple use by a binary NaCl–NaOH solution. We believe the study can provide a potential technological choice for enhanced selenite removal from contaminated waters.
|
Introduction
Selenium is a trace element in the natural environment that exists in four different oxidation states (-II, 0, IV, VI) and organic selenium,1 among which the oxyanionic species selenite [Se(IV)] and selenate [Se(VI)] are the predominant forms of Se in most aqueous media2–4 and selenite is more mobile and readily transported in ground water.5 Selenium is essential to humans and animals. However, it proves toxic when assimilated in excess,6,7 and the margin of Se is very narrow between nutritionally optimal and potentially toxic dietary exposures for vertebrate animals. In view of this, a strict drinking water standard of 10 ppb was proposed for Se by WHO.8
Various treatment processes including reverse osmosis, nanofiltration, microalgal–bacterial treatment, and adsorption have been developed for selenium removal from contaminated waters.9–12 Though some of the approaches are capable of removing selenite to meet increasingly stringent regulations, most of these technologies are economically undesirable. Among these available processes, adsorption seems attractive for selenium removal, provided the selenium-specific and cost-efficient adsorbents.13 In the past decades, ferric oxides have proved to exhibit specific adsorption of some oxyanionic pollutants, viz., arsenite/arsenate,14–16 selenite,5,17 or phosphate,16,18,19 from contaminated waters. Moreover, they are innocuous, inexpensive, readily available, and chemically stable over a wide pH range. Unfortunately, they are always present as fine or ultrafine particles and, thus, it is difficult to directly use them in fixed-bed columns or any other flow-through systems due to their poor mechanical rigidity and excessive pressure drop. An effective and feasible method to overcome such technical bottleneck is to dope particulate ferric oxides within traditional adsorbents of larger particle size. Until now, a variety of traditional adsorbents such as activated carbon,20 diatomite,21,22 cellulose,23,24 and polymeric adsorbents25,26 were employed as the substrate to support hydrated ferric oxides (HFOs), whereas polymeric exchangers have proved to be one of the most promising host materials. It is not only due to the greatly improved permeability in flow-through systems, but also to the Donnan membrane effect exerted by the charged functional groups covalently bound to the polymeric exchanger matrix, which is expected to enhance preconcentration and permeation of the targeted pollutants prior to their removal by the loaded ferric oxides.25–27
In the present study, a hybrid adsorbent (HFO-201) was developed by doping HFOs within the commercial available anion-exchange resin (D-201), and its selenite adsorption was evaluated by batch and fixed-bed column tests. Results indicated that HFO-201 exhibited satisfactory removal toward selenite in terms of high capacity and improved selectivity, as well as feasible regeneration for possible multiple use.
Experimental
Materials
All chemicals were of reagent grade and used without further purification. A stock selenite solution (1000 mg Se L−1) was prepared by dissolving Na2SeO3 in deionized water. The commercially available polymeric anion exchanger D-201, of poly(styrene-divinylbenze) matrix and ammonium functional groups (∼4.3 meq/g), was provided by Zhengguang Electrical Resin Co. Ltd. (Hangzhou, China). The D-201 spherical beads were sieved to keep particle sizes ranging from 0.4 to 0.8 mm. Prior to use, they were subjected to extraction with ethanol in a Soxhlet apparatus to remove the possible residue impurities and then vacuum desiccated at 323 K until reaching a constant weight.
Preparation and characterization of HFO-201
The anion exchanger resin D-201 was chosen as the host material to fabricate the hybrid adsorbent for selenite removal. Detailed processes for preparing HFO-201 can be referred to our proprietary technique.28,29 In brief, the preparation procedure consisted of the following steps: First, D-201 beads were soaked into a binary FeCl3–HCl solution to allow the uptake of FeCl4− onto the inner surface of D-201. Second, the FeCl4− ions preloaded on D-201 beads were in situ decomposed and simultaneously precipitated onto the inner-pore surface of D-201 by transferring them into a NaCl–NaOH solution; the treated D-201 beads were then rinsed with the deionized water until the conductivity of the filtrate was close to that of the deionized water, followed by rinsing with a diluted ethanol solution. Finally, the solid particles were moderately thermally treated to yield the hybrid adsorbent HFO-201.
The specific surface area and the pore size distribution of the adsorbents were determined by N2 adsorption at 77 K on a Micromeritics ASAP-2010C Instrument (USA). Mineralogy of the loaded HFO was determined by powder X-ray diffraction patterns over a wide range of angles (5–55°) using a high-resolution X-ray diffractometer (XTRA, Switzerland). SEM and TEM analysis was also conducted using a LEO-1530 VP electron microscope (Germany) and a Hitachi Model H-800 transmission electron microscope respectively (Hitachi, Japan).
Batch adsorption experiments
Batch adsorption experiments were conducted using a traditional bottle-point method to obtain equilibrium data. A predetermined amount of the adsorbent was introduced into 250-mL flasks containing 100 mL of selenite solution at desired concentrations. The flasks were then transferred to a G25 model incubator shaker with thermostat, and shaken under 160 rpm until the sorption process reached equilibrium. The solution’s pH was adjusted by using 0.10 M HCl or NaOH solutions, and NaCl, NaHCO3, Na2SO4 or KH2PO4 solutions were introduced as the sources of the competing anions when necessary. After reaching equilibrium, samples were filtered through a 0.45-µm syringe filter for selenite and pH determination.
As for the adsorption kinetics, 0.50 g of adsorbent was introduced to 1000-mL of solution containing 20 mg Se/L of selenite in a flask and shaken at 160 rpm. A 0.5-mL aliquot of supernatant was sampled at various time intervals for data analysis. The amount of selenite loaded on adsorbent particles was calculated by conducting a mass balance between the initial and final selenite concentrations.
Fixed-bed column experiments
Bench-scale column experiments were carried out in small Pyrex glass columns (12 mm in diameter), equipped with a water bath to maintain a constant ambient temperature, and 5-mL HFO-201 or D-201 beads were packed within two separate columns to compare their adsorption performance. The synthetic selenite solution containing other competing anions (Cl−, HCO3−, SO42−) was used as the feeding solution. A Lange-850 variable-speed peristaltic pump was used to control the flow volume rate, and an automated fraction collector was used to collect the effluent samples at fixed time intervals. The hydrodynamic conditions, namely superficial liquid velocity (SLV) and empty bed contact time (EBCT), are presented in Fig. 7. In-situ regeneration experiments were also conducted. The selenite-loaded HFO-201 was regenerated by a binary NaCl–NaOH solution (both 5% in mass).
Analysis
The concentration of the soluble selenite of the samples was determined by hydride generation atomic absorption spectrometry (HGAAS).1 The amount of Fe(III) loaded within HFO-201 was analyzed by the ferrozine spectrophotometric method after being extracted by a strong acid solution.30
Results and discussion
Characterization of HFO-201
As shown in Fig. 1a, the hybrid adsorbent HFO-201 retained the spherical geometry and developed a deep brown color as a result of the HFO loadings. The salient properties of D-201 and HFO-201 are summarized in Table 1. The average pore diameter of HFO-201 was somewhat decreased, as the loaded HFO may block some of pores, which was further demonstrated by different SEM micrographs of D-201 and HFO-201 (Fig. 1b). However, the BET surface area of HFO-201 was slightly increased after HFO loadings. This possibly results from the high surface area-to-volume ratio nature of HFO. The TEM image of HFO-201 indicated that HFO was dispersed into the inner surface of D-201 as nanoparticles (Fig. 1c), and the loaded HFO was amorphous, as implied by the XRD pattern (Fig. 1d).
Table 1 Salient properties of polymeric anion exchanger (D-201) and its HFO-loaded derivative (HFO-201)
Adsorbent |
D-201 |
HFO-201 |
Matrix structure |
Poly(styrene-divinylbenzene) |
Functional groups |
R–N+(CH3)3 |
R–N+(CH3)3; HFO |
BET surface area (m2/g) |
25.6 |
26.7 |
Average pore diameter (nm) |
29.3 |
20.6 |
Apparent density (g/cm3) |
0.515 |
0.726 |
HFO content (Fe %) |
0 |
9.3 |
Effect of the solution’s pH
Fig. 2 illustrates the effect of the solution’s pH on selenite removal from the background of 500 mg/L sulfate by HFO-201. As illustrated in Fig. 2, effective selenite removal was achieved in a wide range of pHs (i.e., 3–8), but it decreased as the pH increased especially to >8, where the removal efficiency decreased dramatically. The pH-dependent adsorption behavior could be explained by that the HFO nanoparticles are considered to be a weak diprotic acid with pKa1 = 6.5 and pKa2 = 9.0, and positively charged forms of HFO
are prevalent at lower pH. With the increase of pH, more HFOs are deprotonated and even negatively charged at alkaline pH; consequently, the electrostatic repulsive forces between negatively charged HFO and similarly negatively charged selenite species (HSeO3− and SeO32−) are operative, which is unfavorable for selenite adsorption. The pH-dependent adsorption behaviors also illuminated that the loaded selenite could be stripped from HFO-201 by alkaline solutions.
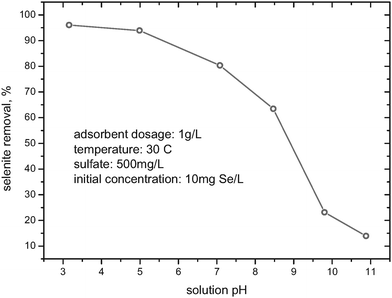 |
| Fig. 2 Effect of solution’s pH on selenite removal by HFO-201. | |
Effect of competing anions
Fig. 3 presents selenite adsorption of HFO-201 and D-201 as a function of the competing SO42−. As shown in Fig. 3, initial addition of SO42− from 0 to 200 mg/L resulted in a dramatic decrease of selenite removal for both HFO-201 and D-201. However, further increase in the added SO42− does not pose any noticeable effect on the selenite adsorption by HFO-201, while that by D-201 was almost completely exhausted. As mentioned earlier, two possible adsorption mechanisms are responsible for the selenite removal by HFO-201, the electrostatic interaction from the ammonium groups bound to the D-201 matrix, and the formation of inner-sphere complexes between the loaded HFO nanoparticles and selenite.5,31 Generally speaking, the electrostatic interaction is non-specific, and the added sulfate can greatly compete with selenite for the adsorption sites of D-201 (i.e., the ammonium groups). However, HFO sequestrated selenite mainly through the formation of inner-sphere complexes,5,31 while SO42− ions usually forms outer-sphere surface complexes on HFO particularly at pH > 6.32,33 Consequently, further increase in the concentration of SO42− ions (i.e., from 200 to 1500 mg/L) did not bring any noticeable effect on the selenite adsorption of HFO-201.
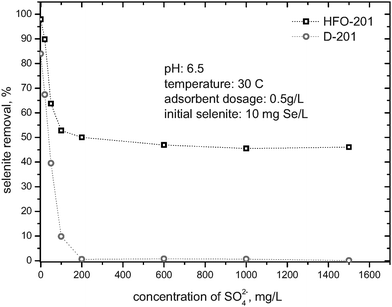 |
| Fig. 3 Effect of the added sulfate anion on selenite removal by HFO-201 and D-201. | |
The effect of other competing anions (i.e., Cl−, HCO3−, PO43−) on the selenite removal of HFO-201 were also examined, and the results presented in Fig. 4 show that all the anions impacted selenite adsorption to various degrees, and that PO43− offers the strongest competition effect. This is possibly because PO43− is also bound with HFO through inner-sphere surface complexes,18 while other anions such as Cl−, HCO3−, SO42−, are weakly bound with surface sites of HFO by forming outer-sphere surface complexes.32,33 Similar results were also reported on selenite adsorption by Fe(III)-coated GAC.13
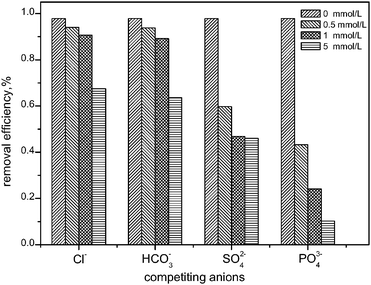 |
| Fig. 4 Effect of the competing anions (i.e., Cl−, HCO3−, SO42−, and PO43−) on selenite removal by HFO-201. | |
Adsorption isotherm and thermodynamic analysis
Selenite adsorption isotherms at different temperatures (15, 30, 45 °C) by HFO-201 are shown in Fig. 5. As shown in Fig. 5, selenite adsorption increased with an increase of ambient temperature, indicating the endothermic nature of the adsorption process. The experimentally collected equilibrium data were then fitted with the Freundlich isotherm model
Where qe is the amount of Se adsorbed in equilibrium, Ce is the concentration of Se in equilibrium, Kf is a constant, as a measure of the adsorption capacity, and n is a measure of the adsorption intensity. The regression coefficients of the adsorption isotherms are larger than 0.99, indicating that the Freundlich model can fit the selenite adsorption reasonably. The free energy change (ΔG), enthalpy change (ΔH), and entropy change (ΔS) for selenite adsorption were calculated as34
Where T is the absolute temperature, R (8.314 J/mol·K) is the universal gas constant. The positive values of ΔH and the negative values of ΔG in Table 2 confirmed that selenite adsorption by HFO-201 is essentially an endothermic and spontaneous one.
Table 2 Thermodynamic parameters of selenite adsorption by HFO-201 (Qe = 0.5 mmol/g)
T (K) |
ΔG (KJ·mol−1) |
ΔH (KJ·mol−1) |
ΔS (J·mol−1·K−1) |
288 |
−8.28 |
8.37 |
57.8 |
303 |
−8.08 |
54.3 |
318 |
−8.22 |
52.2 |
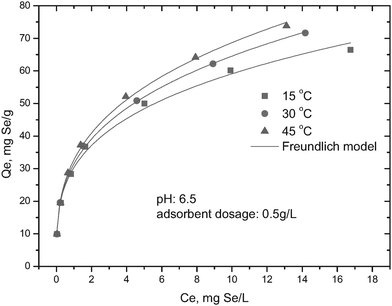 |
| Fig. 5 Temperature-dependent selenite adsorption isotherms onto HFO-201. | |
Adsorption kinetics
Fig. 6 presents the plot of selenite removal versus contact time at three different temperatures. The results revealed that a 200-min adsorption was sufficient to reach adsorption equilibrium, and increasing ambient temperature will shorten the equilibrium time for selenite adsorption. A simple kinetic model, pseudo-first-order model, was then used to describe the process of adsorption,
Where qe and qt are the amount adsorbed in the equilibrium and at time t, respectively, and k1 is the adsorption kinetic constant. Correlation coefficients larger than 0.99 indicate that selenite uptake by HFO-201 can be approximated favorably by the pseudo-first-order model.
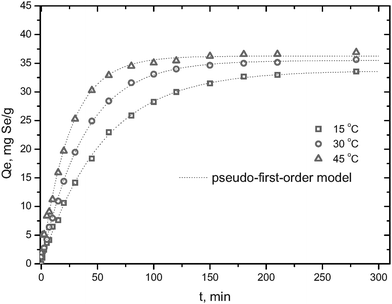 |
| Fig. 6 Adsorption kinetics of selenite onto HFO-201 at three different temperatures. | |
Fixed-bed column experiments
Column selenite adsorption from a synthetic feeding solution onto HFO-201 or D-201 was also performed to evaluate the potential performance of the hybrid adsorbent for practical use. Fig. 7 illustrates an effluent history of a separate fixed-bed column packed with either HFO-201 or D-201 for a feeding solution containing selenite anion and competing anions (Cl−, HCO3−, PO43−). Obviously, HFO-201 significantly outperformed D-201 in selenite removal under otherwise identical conditions. In detail, before a significant breakthrough occurred, the treatment volume for HFO-201 was about 1200 BV, whereas that for D-201 was only about 30 BV. Furthermore, selenite in the effluent before a significant breakthrough by HFO-201 was below 10 µg/L, which is the maximum allowable level of selenium for drinking water recommended by the WHO.
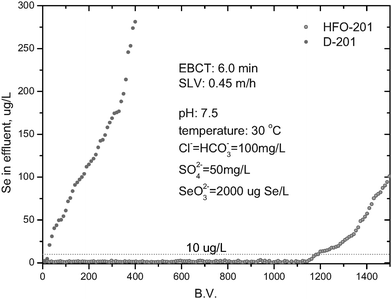 |
| Fig. 7 Breakthrough curves of selenite adsorption from simulated waters onto HFO-201 and D-201 at 30 °C. | |
At the completion of the column flow-through experiment, the exhausted HFO-201 column was subjected to in situ regeneration at 303 K by using a HCl–NaCl solution (both 5% in mass) as the regeneration agent. More than 98% of the selenite could be retrieved from the exhausted HFO-201 within 5 BV of regenerant (data not shown), further indicating its potential application in selenite-contaminated water treatment.
Conclusions
In the current study, a hybrid adsorbent (HFO-201) was prepared by doping nanosized hydrated ferric oxide (HFO) within a polymeric anion exchanger for enhanced selenite removal from waters. Effective selenite removal was achieved by HFO-201 in a wide pH range of 3–8, and lower pH is more favorable for selenite adsorption. The coexisting anions (sulfate, phosphate, chloride, and hydrocarbonate) would compete with selenite adsorption onto HFO-201 to various degrees; however, HFO-201 still exhibits preferable selenite adsorption due to the specific HFO-selenite interaction (i.e., forming inner-sphere complexes). Column adsorption tests proved that HFO-201 significantly outperformed D-201 in selenite removal from the background of competing anions at greater levels, with an effective treatment volume ∼40 times bigger than the latter one. In addition, selenite removal by HFO-201 results in a conspicuous decrease of this toxic element from 2.0 mg/L to below 0.010 mg/L (the drinking water standard recommended by WHO). The exhausted HFO-201 is amenable to efficient regeneration by binary NaCl–NaOH for possible multiple use.
Acknowledgements
We greatly acknowledge the financial support from the Program for New Century Excellent Talents in University of China (NCET07-0421), the Ministry of Water Resources (200808104), the Ministry of Education of China (200802840034), and Jiangsu Department of Science and Technology (BE2009669).
References
- Y. Q. Zhang, J. N. Moore and W. T. Frankenberger, Environ. Sci. Technol., 1999, 33, 1652–1656 CrossRef CAS.
- F. Séby, M. Potin-Gautier, E. Giffaut and O. F. X. Donard, Analusis, 1998, 26, 193–198 CrossRef CAS.
- F. Séby, M. Potin-Gautier, E. Giffaut, G. Borge and O. F. X. Donard, Chem. Geol., 2001, 171, 173–194 CrossRef CAS.
-
L. W. Jacobs, American Society of Agronomy, Inc., 1989, Madison, WI.
- K. M. Parida, B. Gorai, N. N. Das and S. B. Rao, J. Colloid Interface Sci., 1997, 185, 355–362 CrossRef CAS.
- H. W. Lakin, Adv. in Chem. Series, 1973, 123, 96–111 Search PubMed.
- D. Cherdwongchareonsuk, A. P. Aguas, R. Henrique, S. Upatham and A. S. Pereira, Hum. Exp. Toxicol., 2003, 22, 551–557 CrossRef CAS.
-
World Health Organization, Guideline for drinking-water quality (first addendum to 3rd edition): Recommendations, 2006, WHO: Geneva Search PubMed.
- B. J. Marinas and R. E. Selleck, J. Membr. Sci., 1992, 72, 211–229 CrossRef CAS.
-
Y. K. Kharaka, J. J. Thordsen, R. A. Schroeder and J. G. Setmire, Minor Elements 2000: Processing And Environmental Aspects Of As, Sb, Se, Te, And Bi, 2000, 371–379 Search PubMed.
- V. Mavrov, S. Stamenov, E. Todorova, H. Chmiel and T. Erwe, Desalination, 2006, 201, 290–296 CrossRef CAS.
- D. Peak, J. Colloid Interface Sci., 2006, 303, 337–345 CrossRef CAS.
- N. Zhang, L. S. Lin and D. C. Gang, Water Res., 2008, 42, 3809–3816 CrossRef CAS.
- M. Arienzo, P. Adamo, J. Chiarenzelli, M. R. Bianco and A. D. Martino, Chemosphere, 2002, 48, 1009–1018 CrossRef CAS.
- J. H. Yoe, J. Am. Chem. Soc., 1930, 52, 2785–2790 CrossRef CAS.
- M. Streat, K. Hellgardt and N. L. R. Newton, Process Saf. Environ. Prot., 2008, 86, 21–30 Search PubMed.
- C. M. Su and D. L. Suarez, Soil Sci. Soc. Am. J., 2000, 64, 101–111 CAS.
- N. Khare, D. Hesterberg and J. D. Martin, Environ. Sci. Technol., 2005, 39, 2152–2160 CrossRef CAS.
- L. Zeng, X. M. Li and J. D. Liu, Water Res., 2004, 38, 1318–1326 CrossRef CAS.
- M. Jang, W. F. Chen and F. S. Cannon, Environ. Sci. Technol., 2008, 42, 3369–3374 CrossRef CAS.
- M. Jang, S. H. Min, T. H. Kim and J. K. Park, Environ. Sci. Technol., 2006, 40, 1636–1643 CrossRef CAS.
- M. Jang, S. H. Min, J. K. Park and E. J. Tlachac, Environ. Sci. Technol., 2007, 41, 3322–3328 CrossRef CAS.
- X. J. Guo and F. H. Chen, Environ. Sci. Technol., 2005, 39, 6808–6818 CrossRef CAS.
- X. J. Guo, Y. H. Du, F. H. Chen, H. S. Park and Y. N. Xie, J. Colloid Interface Sci., 2007, 314, 427–433 CrossRef CAS.
- L. Cumbal and A. K. Sengupta, Environ. Sci. Technol., 2005, 39, 6508–6515 CrossRef CAS.
- P. Puttamraju and A. K. Sengupta, Ind. Eng. Chem. Res., 2006, 45, 7737–7742 CrossRef CAS.
- Q. R. Zhang, B. C. Pan, W. M. Zhang, B. J. Pan, K. Jia and Q. X. Zhang, Environ. Sci. Technol., 2008, 42, 4140–4145 CrossRef CAS.
-
B. C. Pan, X. Q. Chen and W. M. Zhang, Chinese Patent No.: CN1777023A, 2005.
- Q. J. Zhang, B. C. Pan, X. Q. Chen, W. M. Zhang, B. J. Pan, Q. X. Zhang, L. Lv and X. S. Zhao, Sci. China, Ser. B: Chem., 2008, 51, 379–385 CrossRef CAS.
- M. V. Dawson and S. J. Lyle, Talanta, 1990, 37, 1189–1191 CrossRef CAS.
- C. M. Su and D. L. Suarez, Soil Sci. Soc. Am. J., 2000, 64, 101–111 CAS.
- G. Lefevre, Adv. Colloid Interface Sci., 2004, 107, 109–123 CrossRef CAS.
- H. Wijnja and C. P. Schulthess, J. Colloid Interface Sci., 2000, 229, 286–297 CrossRef CAS.
- B. C. Pan, Q. X. Zhang, F. W. Meng, X. T. Li, X. Zhang, J. Z. Zheng, W. M. Zhang, B. J. Pan and J. L. Chen, Environ. Sci. Technol., 2005, 39, 3308–3313 CrossRef CAS.
Footnote |
† Part of a themed issue dealing with water and water related issues. |
|
This journal is © The Royal Society of Chemistry 2010 |
Click here to see how this site uses Cookies. View our privacy policy here.