DOI:
10.1039/C0AY00430H
(Paper)
Anal. Methods, 2010,
2, 1962-1968
Received
5th July 2010
, Accepted 4th September 2010
First published on
15th October 2010
1. Introduction
Catechol (CA) and Dopamine (DA) are important biological redox compounds, which are capable of transferring two electrons coupled with two protons reversibly in a variety of biochemical reactions including as neurotransmitters in the central nerve system.1 Selective detection of the catecholamines without interferences from the co-existing biological species such as ascorbic acid (AA), uric acid (UA), cystine (CySH) and peroxide (H2O2) is a challenging research interest. Tyrosinase and polyphenol oxidase enzymes coupled with electron-shuttling co-factors on the working electrode or absorbance-/fluorescence-probes were specifically used to detect the catecholamines in neutral pHs by electrochemical or UV-Vis/fluorescence based bio-sensing methods, respectively.2–6 The enzyme based detection methodologies are often complicated, expensive, and time consuming, and several precautions should be taken to address the stability and temperature concerns.3 In this respect non-enzyme based selective sensing systems are attractive, if they could be able to detect the catecholamines selectively at physiological pHs. Here in, we are reporting an interesting system based on the ferrocene species (fc)-ferrocene and ferrocenium ion-entrapped Nafion (Nf) membrane modified glassy carbon electrode (GCE/{Nf-fc}-MME) - for highly selective, enzyme-less sensing of the catecholamines, CA and DA, in a physiological medium of pH 7 phosphate buffer solution (PBS), without any interference from AA, UA, CySH and H2O2.
Ferrocene (fc, Fe0(C5H5)2) is a basic organometallic compound considered to be a bench-mark model reversible electron-transfer system in non-aqueous medium.7 Because of its neutral charge and non-functional group characters, the ferrocene and its derivatives are poorly adsorbed on the electrode surface and hence it is always difficult to prepare fc based chemically modified electrodes.2,8,9 On the other hand, several functionalized fc derivatives were tested to chemically immobilize on the electrode surface, for example, self-assembled monolayers (SAMs) of ferrocenylalkane thiols,10N-2-methylferrocenecaprioc acid and thiol appended fullerene-ferrocene on Au electrode,11,12N-(3-(trimethoxysilyl)propyl)ferrocene polymerized material,13 ferrocenylmethyltrimethylammonium-iodide polypyrrole conducting polymer,14 ferrocenecarboxylic acid-modified ordered mesoporous carbon,15etc. However, the electrode fouling effect was found to be a serious problem for all the electrodes.2,12 Meanwhile, Nf is a well known polyperfluoro ionomeric membrane having proton conducting behavior, widely used as a membrane in fuel cells.16 Nf contains a hydrophobic poly(tetrafluoroethylene) component with regularly spaced short perfluorovinyl ether side-chains, each terminated with a highly hydrophilic sulfonic acid group.17–19 The perfluorosulfonate ionomers possess network structures with interconnected reverse micellar like ion clusters, widely used as a source to ion-exchange the cationic species.18 Nafion-fc combined matrixes have been reported for various applications, including as a cofactor for the glucose biosensor,20,21 nitric oxide sensor22etc. Concerning the existing enzyme-less sensors for catechol and its derivative, carbon and its derived surfaces including carbon nanotubes (CNTs),23–25 were reported as efficient detecting electrodes,23–25 but often with tremendous interference from co-exiting species, as we mentioned earlier, with serious electrode instability character (may be due to extensive de-activation of the surface). In addition, in some cases, instead of any catalytic signals, catechol strongly adsorbed, for example, on pre-anodized glassy carbon and screen-printed electrodes, and encapsulated inside CNTs on CNT-modified electrode.26–28 Meanwhile, mediator modified carbon electrodes were also reported for catechol estimation purpose.29,30 First time in this work, unlike the conventional irreversible electrocatalytic behaviour,23–30 enhanced reversible electrochemical redox behaviour was observed for catechol and dopamine selectively on the GCE/{Nf-fc}-MME, and these electrochemical features were further cautiously utilized for the amperometric sensing of the respective compounds at low working potentials in physiological solutions.
Experimental
Chemicals and reagents
Nafion® 117 perflourinated ion-exchange powder, and 5% weight solution in a mixture of lower aliphatic alcohols were obtained from Wago Chemical, Japan. The chemicals viz. ferrocene, catechol and dopamine of analytical grade were purchased from Sigma Aldrich, USA and used as received. Aqueous solutions were prepared using deionized and alkaline KMnO4 distilled water (designated as DD water). Unless otherwise stated, pH 7 phosphate buffer solution (PBS) of 0.1 M ionic strength was used as supporting electrolyte in this study.
Apparatus
Voltammetric measurements were carried out using CHI model 660C electrochemical work station, USA with 10 mL working volume. The three electrode system consisted of glassy carbon (GCE) of 0.0707 cm2 geometrical surface area and its membrane modified electrode (MME) as a working electrode, Ag/AgCl with 3 M KCl as a reference electrode and platinum wire as a counter electrode. The Bio-analytical system (BAS, USA) polishing kit was used to polish the GCE surface. The surface of the GCE was cleaned both mechanically (polished with 1 μm alumina powder, cleaned with acetone and washed with DD water) and electrochemically (by performing cyclic voltammetry (CV) for 10 cycles in the potential window −0.4 V to 1.0 V vs. Ag/AgCl at a potential scan rate (v) of 50 mV s−1 in pH 7 PB solution.
Procedures
Nf-fc colloidal solution was first prepared by mixing 10 mg of ferrocene with 100 μL of 5 wt% Nafion solution (designated as {Nf-fc}colloid), followed by 10 min of sonication at room temperature (T ∼ 28 °C). The mixture appeared as a clear yellow colored colloidal solution, and was stable for over 2 months at room-temperature. The {Nf-fc}colloid served as a stock solution to prepare the fc-membrane modified electrodes. The GCE/{Nf-fc}-MME was prepared by the following procedure: 2 μL of the {Nf-fc}colloid solution (Nafion 2 wt% as optimal) was drop-coated on cleaned GCE surface and allowed to dry for 20–30 min. The film was further electrochemically pretreated by continuous cycling (n = 40) in pH 7 PBS in the potential window −0.4 to 0.8 V vs. Ag/AgCl at v = 50 mV s−1. There was a marked change in the redox behavior with the system up to 30 cycles and after that stable responses were noticed. Scheme 1 illustrates the possible structure of fc immobilized Nafion membrane matrix. Initially, the fc can be retained in the hydrophobic domains of Nafion, while later, since the oxidized form of fc, ferrocenium ion (fc+), is water soluble, it can transfer to the hydrophilic channels and get electro-statically stabilized with the anionic sulfonic acid group. It is expected that there will be some dynamic equilibrium, fc ⇔ fc+, within the hydrophilic and hydrophobic regions. Since dissolved oxygen did not influence the present electrochemical system, experiments were all performed with normal dissolved oxygen (DO). Amperometric i-t method was used for the quantitative electrochemical detection of the catecholamines at a fixed operating potential of 200 mV vs. Ag/AgCl in pH 7 PBS. Scanning electron-microscopy (FEI Quanta FEG 200 High resolution SEM instrument) was done with 3 μL {Nf-fc}colloid drop-coated conducting glass plate. Electrochemical impedance spectroscopic measurements in a frequency window of 10−3 to 105 Hz, and the data fitting analyses were carried out using the built-in programs in CHI 660C instrument.
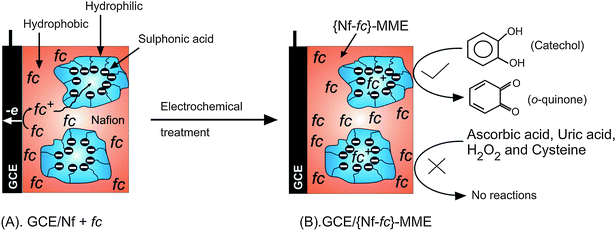 |
| Scheme 1 Illustration for GCE/{Nf-fc}-MME preparation, its possible structure and selective catechol mediation. | |
3. Results and discussion
Fig. 1A and B are typical SEM pictures of {Nf-fc}-MME on conducting glass plate under different magnifications. Clear and smooth membrane structure with ice-flakes like arrangement on the surface can be noticed. There are few spots on the plate at the boundary of the flakes, and these may be due to some precipitated fc particulates within the Nafion membrane modified substrate. But on the whole, a smooth homogenous structure was noticed within the {Nf-fc}-MME.
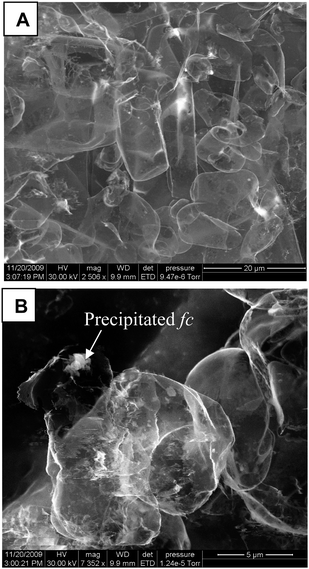 |
| Fig. 1 SEM photo graphs of a {Nf-fc}-MME on conducting glass substrate at different magnifications. | |
Fig. 2A and B display typical cyclic voltammetric responses of GCE/{Nf-fc}-MME without and with CA and DA in pH 7 PBS. The bare GCE/{Nf-fc}-MME showed well-defined redox behavior with an equilibrium potential (E1/2) and peak-to-peak separation (ΔEp) of 0.15 ± 0.005 V vs. Ag/AgCl and 200 mV, respectively, due to the bound ferrocene/ferrocenium (fc/fc+) redox active sites within the Nafion membrane. The E1/2 obtained in this work is similar to that reported by Rubinstein for the ferrocenium ion-Nafion membrane modified electrode prepared by electrochemical doping method in saturated aqueous solution.31 The fc/fc+ redox currents were enhanced thrice in presence of CA or DA with a lower ΔEp value of ∼150 mV, suggesting that the mechanically immobilized fc improved electron-transfer of these compounds and catalyzed their electro-redox reactions on GCE/{Nf-fc}-MME. Control experiments for the electrochemical behavior of CA or DA on GCE and GCE/Nf systems resulted in poor redox features with very weak current signals, in close agreement with the literature reports on the unmodified surfaces in neutral pHs.25 As the redox peaks of CA are centered at E1/2 = 100 ± 5 mV vs. Ag/AgCl in the potential region of fc to fc+ oxidation (E1/2 = 150 ± 5 mV vs. Ag/AgCl) (Fig. 2), the mediated catechol redox reactions at the fc/fc+ electrode can be represented as follows:
| 2{fc+}nf + catechol ⇌ 2{fc+}nf + o-quinone + 2e− | (II) |
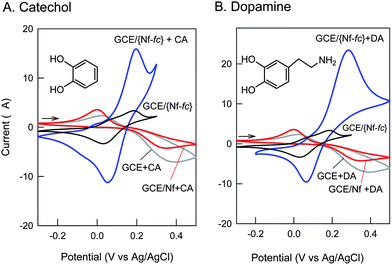 |
| Fig. 2 CV response of 1 mM concentration of Catechol, CA (A) and Dopamine, DA (B) on different working electrodes in pH 7 PBS at a scan rate of 10 mV s−1. | |
Note that the Nafion membrane has cation exchanging property with strong anionic sulfonic groups, which can repel the negatively charged species from the surface. Interestingly, advantage has been taken of this factor to achieve total elimination of interfering biological compounds, such as, ascorbic acid, uric acid, cysteine and nitrite, which failed to show any faradic signal with this electrode (CV data not shown), and hence providing selective redox mediation of some of the neurotransmitters, CA and DA, on GCE/{Nf-fc}-MME.
Effect of wt% of Nafion (1–5%) on the GCE/{Nf-fc}-MME formation in terms of mediation of CA by fc+/fc was further investigated as shown in Fig. 3. The CV responses of the respective membrane modified electrodes were taken after 40 continuous CV cyclings in pH 7 PBS. Increasing the Nafion % led to an increase in the CA mediated current signal up to 2–3%, and after that a decreased trend was noticed. Current (ipa) maximum of ∼15 μA was noticed for the 2–3% Nafion based GCE/{Nf-fc}-MME for 1 mM CA in pH 7 PBS, while the thick 5% Nafion based membrane modified system failed to show any such mediated current signal. Besides, about 180 mV negative potential shift in presence of CA was noticed with the thick Nafion film. The absence of any CA mediated current might be due to some kinetic limitations, in which, the electrochemical fc ⇔ fc+ might be a slow step and the interaction of the solution phase CA (or DA) with the Nafion bound fc/fc+ species might be very weak. Since the 2% Nafion system showed appreciable fc/fc+ redox and its mediation response to CA, it was chosen as an optimal condition for further electro-analytical process.
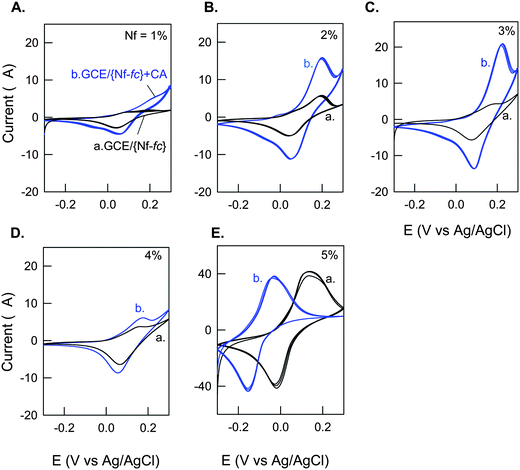 |
| Fig. 3 Effect of Nafion wt% on CV response of GCE/{Nf-fc}-MME without (a) and with (b) 1 mM Catechol (CA) in pH 7 PBS at v = 10 mV s−1. | |
Scan rate (v) effect on the electrochemical behavior of GCE/{Nf-fc}-MME without and with CA was studied as in the Fig. 4 A and B, respectively. For the bare GCE/{Nf-fc}-MME, two peaks appeared at 100 and 200 mV, with the 200 mV peak predominant at low scan rates up to 20 mV s−1. The peak currents increased systematically with increase in scan rate; however, beyond 20 mV s−1 scan rate, the peak at 100 mV increased at the expense of the 200 mV peak. On the other hand, CA oxidation also proceeded with CV response increasing with scan rate, but the 200 mV peak showed exponentially increasing currents especially at scan rates >20 mV s−1. Since the ferrocene species is located at different domains, namely hydrophobic and hydrophilic sites as in the Scheme 1, it is expected that both the domains could have discretely involved in the mediation process. The hydrophilic domain may be responsible for the electron-transfer behavior at relatively less operating potential than the other case, as the analyte CA is hydrophilic in nature. Double logarithmic plot of log(ipa) vs. log (v) yielded linear lines with slope (∂log ipa/∂log v) values of 0.5 and 0.81, respectively, for the cases without and with CA on the GCE/{Nf-fc+}-MME, indicating that fc/fc+ redox reaction in GCE/{Nf-fc}-MME was diffusion-controlled, and CA oxidation was mixed adsorption-diffusion controlled reaction. Diffusion of the fc or fc+ within the Nafion membrane and electron-hopping among neighbors are the prime reaction steps for the diffusion controlled mechanism of the bare GCE/{Nf-fc+}-MME. The mixed mechanism for CA indicates that the mediated CA oxidation process might have occurred inside the outer-layers of Nafion membrane. Exact mechanistic details are, however, unknown at present. Inset Fig. 4B is a plot of current function, ipa/v1/2versusv1/2 for CA electrochemical response at GCE/{Nf-fc+}-MME. The current function decreased with increase in the scan rate, which is a typical example for the EC' electrocatalytic mechanism,32 supporting further the proposed reactions (Eqn (I) and (II)).
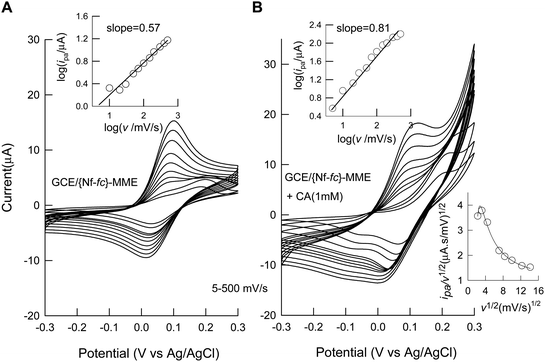 |
| Fig. 4 Effect of scan rate on CV response of GCE/{Nf-fc}-MME without (A) and with (B) 1 mM Catechol (CA) in pH 7 PBS. Inset plots are log(ipa) vs. log (v) and ipa/v1/2 (current function) vs.v1/2 (for catechol). | |
Fig. 5A displays the CV response of the GCE/{Nf-fc+}-MME with increase in the CA concentrations in pH 7 PBS. The peak current at 200 mV vs. Ag/AgCl constantly increased with increase in the [CA] along with some positive shift in the peak potential. Meanwhile, a lower oxidation peak current at ∼0 V vs. Ag/AgCl was also noticed at [CA] > 5 mM. As indicated already for the bare GCE/{Nf-fc}-MME, two different forms of fc species existing in hydrophilic and hydrophobic domains and their corresponding mediation reactions may be responsible for the CA oxidation peaks at 0 and 200 mV vs. Ag/AgCl respectively, in Fig. 5A. Interface resistance between the hydrophobic channel-bound fc species and CA might be the cause for the positive potential shift of the 200 mV oxidation peak with CA concentration. Such resistance related effect could be minute for the 0 mV CA oxidation peak corresponding to hydrophilic channel-bound fc species.
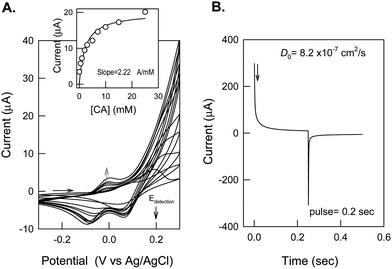 |
| Fig. 5 (A) CV response of GCE/{Nf-fc}-MME with increasing catechol concentration in pH 7 PBS at a scan rate of 10 mV s−1. (B) Chrono-amperometric response of GCE/{Nf-fc}-MME with 1 mM Catechol at a potential step 300 to −150 mV vs. Ag/AgCl in pH 7 PBS. | |
Since, the 200 mV peak currents were larger and systematic, they were taken for further calibration by CV. Inset of Fig. 5A shows a typical plot of peak current measured at 200 mV vs. [CA]. The plot was linear up to 7 mM of CA with a current sensitivity and regression values of 2.22 μA mM−1 and 0.9989 respectively. A saturation in the peak current response was noticed after [CA] > 7 mM. This observation partially supports the active site ({fc}nf/{fc+}nf)-mediated oxidation reaction proposed in eqn (I) and (II). Repeated CV cycling of the GCE/{Nf-fc+}-MME for the CA mediation did not show any marked alternation in the peak current. There was no sign of any analyte peaks, when the electrode was transferred from a CA-containing experimental solution to a blank solution (data not enclosed), unlike the previous studies viz., activated GCE for covalent immobilization of catechol27 and encapsulation of catechol on multi walled carbon nanotubes (MWNT) modified electrodes28 with strong adsorption effect. This is a clear advantage for easy renewal of the working surface. Before analytical studies, the working electrode was subjected to chrono-amperometric and impedance analysis to estimate the diffusion coefficient and the kinetic parameters.
Typical chrono-amperometric response of the GCE/{Nf-fc+}-MME obtained by setting the working electrode potential at 0.3 V (step 1) and −0.15 V (step 2) with a forward and reverse pulse width of 0.2 s is represented in Fig. 5B. Using the plot ivs.t−½, based on the Cottrell equation i = nFACD½Π−½t−½,32 diffusion coefficient, D, for the solution phase CA was calculated as 8.2 × 10−7 cm2 s−1, which is about 8 times higher than the value of 1 × 10−7 cm2 s−1 reported by Atta et al. for the poly(3-methylthiophene) modified Pt electrode in 0.1 M pH 7.5 PBS by cyclic voltammetric method.33 The difference in the D values might be due to the different evaluation techniques adopted in the two studies.
Fig. 6 shows the electrochemical impedance spectroscopy (EIS) responses of GCE/{Nf-fc}-MME, GCE/Nf and GCE electrodes recorded at an applied potential of 150 mV vs. Ag/AgCl with 1 mM of the CA uniformly. All the three systems qualitatively showed a semicircle at high frequencies followed by a linear line at low frequencies corresponding to faradic electron-transfer and diffusional processes, respectively. However, the quantitative values were different. The corresponding spectra were subjected to Randle's circuit analyses using the equivalent circuit of mixed kinetic and diffusional control shown in Fig. 6, where Rs = solution phase resistance, RCT = charge transfer resistance, Cdl= double layer capacitance and W1 = Warburg impedance for the diffusion to quantitatively find out the circuit component values for the different systems. The kinetic parameters, the exchange current density, Io, and the apparent heterogeneous rate constant, khapp, were further deduced from the RCT from the following standard equations:32
where
c = concentration of
analyte (
catechol) and other symbols have their usual significance.
Table 1 provides the corresponding data. The
RCT value for the CA
oxidation at GCE/{Nf-
fc}-MME was calculated to be 0.21 K ohm, which is ∼100 times lower than that of the same substrate on unmodified
electrode (23.74 K ohm). These observations clearly show the efficient electron-transfer behavior of the CA system on the GCE/{Nf-
fc}-MME.
Parameter |
GCE/Nf |
GCE |
GCE/{Nf-fc}-MME |
R
s (Ω) |
152 |
68.84 |
168 |
C
dl (F) |
1.75 × 10−6 |
0.52 × 10−6 |
10.16 × 10−6 |
R
CT (KΩ) |
23.74 |
49.34 |
0.21 |
W
1
|
1.80 × 10−5 |
1.8 × 10−5 |
3.33 × 10−5 |
I
o (A) |
5.39 × 10−7 |
2.59 × 10−7 |
6.16 × 10−5 |
k
h
app (cm s−1) |
3.95 × 10−5 |
1.90 × 10−5 |
4.51 × 10−3 |
Finally the analytical utility of the GCE/{Nf-fc}-MME for the amperometric sensing of CA and DA in pH 7 PBS were demonstrated in Fig. 7A and B. At an applied potential of 200 mV vs. Ag/AgCl, the membrane modified electrode showed systematic increase in the current for the 250 μM spikes of CA and DA discretely. Under identical working conditions, the unmodified electrodes, GCE and GCE/Nf, showed poor current responses. Calibration plot for the GCE/{Nf-fc}-MME was linear up to 2 mM and 5 mM, respectively, for CA and DA with a current sensitivity of 1.1 μA mM−1 for both the cases. Relative standard deviation of the last 4th–8th spikes of the compounds yielded values of 0.92 and 2.64%, respectively. The calculated detection limit (S/N = 3) values were 10.8 μM and 22.7 μM respectively in this work. Fig. 8 shows the amperometric response of the other interferencing chemicals at an applied potential of 200 mV vs. Ag/AgCl. As can be seen, cysteine, H2O2 and uric acid showed almost nil response, while the ascorbic acid showed very feeble sensing current signals. Note that fc systems were often reported to be the best electro-catalysts for ascorbic acid and uric acid oxidation.34–36 We have successfully eliminated the interference of the ascorbic acid due to the presence of the Nafion, where the anionic form of ascorbic acid (pKa = 4.10) was effectively repelled from the anionic sulfonic groups. The most likely reason for the cysteine, uric acid and H2O2 elimination at the GCE/{Nf-fc}-MME could be that the {Nf-fc} sites were not active towards oxidation of these chemicals. Such amperometric selectivity (Fig. 8) is rarely achieved with the chemically modified electrodes at neutral pHs. For instance, Nafion/lead-ruthenium oxide pyrochlore chemically modified electrode was reported to be efficient for catechol and dopamine sensing,29 at the same time the electrode could oxidize the cysteine and uric acid too effectively.37,38 The developed sensor is sensitive enough for use in physiological real sample analysis. For example, the CA real sample concentration in human urine (benzene exposed factory workers) was reported to be in the window of 90.8–345 μM.39 Note that the present sensor electrode system showed a detection limit value of 10.8 μM, which is about 9 times lower than the real sample concentration, without any interference from uric acid. Finally, stability of the working electrode was tested for couple of days (Fig. S1, ESI†). Negligible difference in the redox features was noticed. Thus, the choice of the Nafion membrane as a matrix for the fc/fc+ redox seems to have a promising role in enhancing the redox activity of fc/fc+ for the selective sensing of important neurotransmitters, catechol and dopamine.
![Typical amperometric i-t responses of GCE/{Nf-fc}-MME (250 μM each) for the detection of catechol (A) and dopamine (B) at an applied potential of 200 mV vs. Ag/AgCl in pH 7 PBS under hydrodynamic condition. Inset figures are plots of respective Current vs. [analyte].](/image/article/2010/AY/c0ay00430h/c0ay00430h-f7.gif) |
| Fig. 7 Typical amperometric i-t responses of GCE/{Nf-fc}-MME (250 μM each) for the detection of catechol (A) and dopamine (B) at an applied potential of 200 mV vs. Ag/AgCl in pH 7 PBS under hydrodynamic condition. Inset figures are plots of respective Current vs. [analyte]. | |
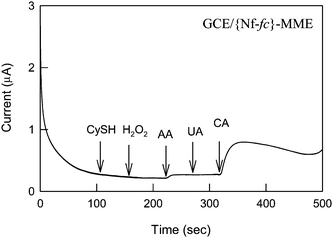 |
| Fig. 8 Amperometric i-t response of GCE/{Nf-fc}-MME with different analytes (250 μM each) at an applied potential of 200 mV vs. Ag/AgCl under hydrodynamic conditions in pH 7 PBS. | |
4. Conclusions
Ferrocene bound Nafion membrane modified glassy carbon electrode was successfully demonstrated for the enzyme-less detection of some neurotransmitters, catechol (CA) and dopamine (DA) without any interferences from cysteine, uric acid, hydrogen peroxide and ascorbic acid at an applied potential of 200 mV vs. Ag/AgCl in pH 7 phosphate buffer solution. The basic mediation mechanism was found to be an electron transfer-coupled chemical reaction in which, the fc/fc+ redox mediates the catechol/o-quinone conversion quasi-reversibly. Nafion films with moderate wt% (2–3%) were found to be optimal for the best mediation of CA and DA. Both cyclic voltammetric and amperometric responses showed systematic increase in the current signals with increase in the concentration of the compounds. Calculated current sensitivity value by amperometric method was 1.1 μA mM−1 for both the compounds. The electrode was found to be stable for a couple of days with negligible difference in the detection current signals.
5. Acknowlegement
Authors gratefully acknowledge the Department of Science and Technology (DST), India for the financial support.
6. References
- R. M. Wightman, E. Strope, P. M. Plotsky and R. N. Adams, Nature, 1976, 262, 145–146 CrossRef CAS.
- S. Cosnier, Biosens. Bioelectron., 1999, 14, 443–456 CrossRef CAS.
- F. Daigle and D. Leech, Anal. Chem., 1997, 69, 4108–4112 CrossRef CAS.
- J. K. Mbouguen, E. Ngameni and A. Walcarius, Biosens. Bioelectron., 2007, 23, 269–275 CrossRef CAS.
- F. F. Orlando and I. da Cruz Vieira, Anal. Chim. Acta, 1997, 354, 51–57 CrossRef CAS.
- D. Fiorentino, A. Gallone, D. Fiocco, G. Palazzo and A. Mallardi, Biosens. Bioelectron., 2010, 25, 2033–2037 CrossRef CAS.
-
K. Izutsu, Electrochemistry in Nonaqueous Solutions, Wiley-VCH, Tokoyo, 2001 Search PubMed.
- S. L. Brooks, R. E. Ashby, A. P. F. Turner, M. R. Calder and D. J. Clarke, Biosensors, 1987, 3, 45 CAS.
- G. C. Zhao, M. Q. Xu and Q. Zhang, Electrochem. Commun., 2008, 10, 1924–1926 CrossRef CAS.
- T. Kondo, M. Takechi, Y. Sato and Kohei Uosaki, J. Electroanal. Chem., 1995, 381, 203–209 CrossRef CAS.
- X. Wang, Z. Gershman, A. B. Kharitonov, E. Katz and I. Willner, Langmuir, 2003, 19, 5413–5420 CrossRef CAS.
- V. T. Hoang, L. M. Rogers and F. D'Souza, Electrochem. Commun., 2002, 4, 50–53 CrossRef CAS.
- C. A. Beasley and R. W. Murray, Langmuir, 2009, 25, 10370–10375 CrossRef CAS.
- S. Paul, N. N. Chavan and S. Radhakrishnan, Synth. Met., 2009, 159, 415–418 CrossRef CAS.
- J. C. Ndamanisha and L. Guo, Biosens. Bioelectron., 2008, 23, 1680–1685 CrossRef CAS.
- Kenneth A. Mauritz and Robert B. Moore, Chem. Rev., 2004, 104, 4535–4585 CrossRef CAS.
- J.-M. Zen and A. S. Kumar, Acc. Chem. Res., 2001, 34, 772–780 CrossRef CAS.
- A. S. Kumar, T. Tanase and M. Iida, Langmuir, 2007, 23, 391–394 CrossRef CAS.
- A. S. Kumar and P. Swetha, J. Electroanal. Chem., 2010, 642, 135–142 CrossRef CAS.
- S. Dong, B. Wang and B. Liu, Biosens. Bioelectron., 1992, 7, 215–222 CrossRef CAS.
- D.-M. Zhou, H.-X. Ju and H.-Y. Chen, Sens. Actuators, B, 1997, 40, 89–94 CrossRef.
- T. M. Vergheese and S. Berchmans, Electrochim. Acta, 2006, 52, 567–574 CrossRef CAS.
- C. D. Allred and R. L. McCreery, Anal. Chem., 1992, 64, 444–448 CrossRef CAS.
- S. H. DuVall and R. L. McCreery, Anal. Chem., 1999, 71, 4594–4602 CrossRef CAS.
- Z. Xu, X. Chen, X. Qu and S. Dong, Electroanalysis, 2004, 16, 684–687 CrossRef CAS.
- R. L. McCreery, Chem. Rev., 2008, 108, 2646–2687 CrossRef CAS.
- A. S. Kumar, S. Sornambikai, P. Gayathri and J.-M. Zen, J. Electroanal. Chem., 2010, 641, 131–135 CrossRef CAS.
- A. S. Kumar and P. Swetha, Langmuir, 2010, 26, 6874–6877 CrossRef.
- J.-M. Zen and I.-L. Chen, Electroanalysis, 1997, 9, 537–540 CAS.
- J.-M. Zen, H. H. Chung and A. S. Kumar, Anal. Chem., 2002, 74, 1202–1206 CAS.
- I. Rubinstein, J. Electroanal. Chem., 1984, 176, 359–362 CrossRef CAS.
-
A. J. Bard and L. R. Faulkner, Electrochemical Methods,2nd edition, John Wiley & Sons, Inc., Newyork, 2001 Search PubMed.
- N. F. Atta, I. Marawi, K. L. Petticrew, H. Zimmer, H. B. Mark Jr and A. Galal, J. Electroanal. Chem., 1996, 408, 47–52 CrossRef.
- L. F. andez and H. Carrero, Electrochim. Acta, 2005, 50, 1233–1240 CrossRef.
- J. C. Ndamanisha and L. Guo, Biosens. Bioelectron., 2008, 23, 1680–1685 CrossRef CAS.
- M. H. P. Azar and R. Ojani, Talanta, 1995, 42, 1839–1842 CrossRef CAS.
- J. M. Zen, A. S. Kumar and J. C. Chen, Anal. Chem., 2001, 73, 1169–1175 CrossRef CAS.
- J. M. Zen and J. S. Tang, Anal. Chem., 1995, 67, 1892–1895 CrossRef CAS.
- O. Inoue, K. Seiji, M. Kasahara, H. Nakatsuka, T. Watanabe, S.-G. Yin, G.-L. Li, S.-X. Cai, C. Jin and M. Ikeda, Br. J. Ind. Med., 1998, 45, 487–492.
Footnote |
† Electronic supplementary information (ESI) available: Fig. S1: Cyclic voltammetric response of GCE/{Nf-fc}-MME in pH 7 PBS recorded at different days. See DOI: 10.1039/c0ay00430h |
|
This journal is © The Royal Society of Chemistry 2010 |
Click here to see how this site uses Cookies. View our privacy policy here.