DOI:
10.1039/C0AY00419G
(Paper)
Anal. Methods, 2010,
2, 1779-1783
Received
1st July 2010
, Accepted 5th August 2010
First published on
23rd September 2010
Abstract
High performance liquid chromatography (HPLC) on titania column was performed for phosphatidylcholine (PC) separation and analysis. To fully understand the retention mechanism of PC on titania, the effect of mobile phase type, %acetonitrile (%ACN), phosphate buffer concentration, buffer pH and flow rate were all studied. Separation depended on the eluent conditions and PC retention was attributed to the cooperation of hydrophilic interaction and ligand exchange. PC in soybean lecithin products was completely separated from other components within 6 min and quantitative analysis was achieved by an external standard method, with a correlation coefficient (R2) of 0.9947. The method precision and detection limit of PC were 3.40% and 0.2 μg, respectively, with the recovery ranging from 91% to 101%. Moreover, PC and glycerol monostearate standard mixture was successfully isolated by the proposed method, which underlined the selective affinity of titania for phosphorylated lipids. With the superiority of simplicity, rapidity and accuracy, this HPLC method based on titania is a competent method for the determination of PC in lecithin samples with prospects in the field of organo-phosphates analysis.
Introduction
Titania has been drawing increasing attention in recent years, owing to its great mechanical strength, excellent pH stability and amphoteric ion exchanger characteristics.1,2 One of the most fascinating features of titania may be its ligand-exchange behavior towards organo-phosphates such as phosphoproteins, phosphopeptides, nucleotides and phospholipids, this results from the fact that unsaturated titania ions (IV) are strong Lewis acid sites which have selective affinity for the strong electronegative phosphonate group of the above-mentioned compounds.3 Furthermore, with increase of positive charge density on the titania surface under acidic conditions, the selective affinity of titania towards electronegative organo-phosphates strengthens. During the past decade, considerable efforts have been made to titania based on enrichment and separation of organo-phosphates.3–8 For instance, Hata and coworkers combined titania and monolithic columns to isolate phosphopeptides from nonphosphorylated peptides.5 Hsieh et al. developed a titanium dioxide (TiO2) nanoparticle pipette-tip for selective enrichment of phosphopeptides.4 Zhou and Lucy performed a titania packed column to separate AMP, ADP, ATP and a set of their pathway intermediates.3
Phospholipids (PLs) are divided into two main groups: glycerophospholipids and sphingolipids, which play important roles in various cellular responses such as cellular membrane components and different biological pathways.8,9 Glycerophospholipids include principally phosphatidylcholine (PC, the chemical structure was shown in Fig. 1), phosphatidylinositol (PI), phosphatidylethanolamine (PE) and phosphatidylserine (PS).8,10 Conventional determination of PLs is mainly achieved by thin-layer chromatography (TLC) and high performance liquid chromatography (HPLC).7,9–18 TiO2 has been extensively studied as a new packing material for organo-phosphates separation and enrichment. However, to the best of our knowledge, PLs isolation and analysis by HPLC on titania column has not been reported to date. Herein, a HPLC method on a normal-phase titania column was developed to determine PC in lecithin samples. The effect of mobile phase type, %acetonitrile (%ACN), buffer concentration, buffer pH and flow rate were studied to fully understand the retention behaviors of PC on titania column. Furthermore, qualitative and quantitative determination of PC in soybean lecithin products by HPLC on titania was successfully developed for the first time.
Experimental
Reagents and materials
Sodium phosphate monobasic, sodium phosphate dibasic, ACN, methanol (MeOH), hexane and isopropyl alcohol were all of LC grade and obtained from Kermel Chemical Reagent Co., Ltd. (Tianjin, China). Double distilled water was used for the preparation of the mobile phase. L-α-phosphatidylcholine (PC) of analytical grade as standard was purchased from SigmaAldrich (St. Louis, MO, USA). Glycerol monostearate was obtained from Changsha Organic Reagent Factory (Changsha, China). Sample materials: soybean phosphatidylcholine (PC-50) (PC content is ca. 50%) was from Meiyasi Phospholipid Technology Co., Ltd. (Beijing, China); Soybean lecithin oil was purchased from the local market; ARSVICA lecithin capsules were from Guangdong Changxing Scientific Health Care Provisions Co., Ltd. (Chaozhou, China); Tomvitm and Cal-SUM lecithin capsules were obtained from Fulaixin Biological Technology Co., Ltd. (Nanning, China). All solutions were filtered through 0.45 μm membrane filters (Millipore China Ltd., Shanghai, China) prior to use.
Chromatographic condition
HPLC experiments were performed with an Agilent 1200 series HPLC system consisting of a single-position pump (model G1310A), a UV detector (model G1314B) operated at a wavelength of 205 nm and an injection valve (model G1328B) with a 20 μL loop. A bare TiO2 Sachtopore-NP column (250 mm × 4.6 mm I.D., 3 μm, 300 Å, Zirchrom, Anoka, MN, USA) was used at the condition of 40 °C with a Yamatake HT-230A column oven. The eluents in different pH and concentration were prepared from 0.2 M phosphate stock solutions. The buffer concentration quoted in this paper were that present after ACN and MeOH addition. All the standards and samples were dissolved in MeOH (In the case of lecithin capsules, the oil in the capsule was centrifuged at 4000 r min−1 for 15 min. The residue could not be dissolved in MeOH and the supernatant was diluted with MeOH for HPLC determination). The resolution of PC was measured by the PC peak and the pre-peak. The quantification method in HPLC is based on the measurement of peak area and the comparison with that of standard PC under the same condition. All samples were dissolved in the same solvent as the eluent except in the section of discussion of %ACN in sample.
Results and discussion
In conventional determination of PLs by HPLC based on silica columns, the systems of chloroform–MeOH,9–15 ACN–MeOH16 and hexane–isopropanol7,17 were usually selected as eluents. Hydrophilic interaction chromatography (HILIC) provides an alternative approach to effectively separate small polar compounds on polar stationary phases and it has been applied to analyze a set of nucleotides3 and carboxylates19 based on a titania column. HILIC is considered as a variant of normal phase chromatography that uses a “reversed phase” eluent, in which water is the stronger eluent and the retention mechanism is partitioning.20 When a high percentage of organic modifier such as ACN is added to the eluent, a water-enriched layer is formed on the hydrophilic stationary phase. Analytes partition between the water-enriched layer and the bulk hydrophobic eluent.19 PC is an amphoteric surfactant with a hydrophilic headgroup and lipophilic fatty acyl side-chains and MeOH serves as a good solvent for PC. Thus, HILIC with MeOH–ACN–water eluent is probably suitable to determine PC which can partition between the special soluble layer and the mobile phase, making appropriate retention and complete separation of PC come true. Therefore, the MeOH–ACN–water system with high %ACN was selected in further study to find the optimal elution condition.
%ACN in sample
Ideally samples in liquid chromatography should be dissolved in the same solvent as the eluent. Differences in viscosity between the sample and eluent can lead to peak distortion, while differences in the solvent strength can lead to band broadening.3 The influence of %ACN in sample on the retention and efficiency of PC was first tested. Minor changes in retention but dramatic increase (3.4-fold) in efficiency occurred as the %ACN in sample was varied (see Fig. S1†). It is consistent with the result of Zhou and Lucy.3 To this end, all further studies were performed with samples dissolved in the same solvent as the eluent.
In this section, phosphate buffer was added in the mobile phase, which is always adopted in HPLC analysis of organo-phosphates based on titania. Konishi et al. used a monolithic TiO2 rod column to separate AMP, ADP and ATP eluted by 50 mM phosphate buffer in 60% ACN.21 A similar work was also performed by Zhou and Lucy on a titania packed column with 10 mM phosphate buffer in 75% ACN as the mobile phase.3 Furthermore, buffer pH plays an important role on the retention of analytes. To separate and enrich phosphorylated peptides more effectively, acetic acid22 and trifluoroacetic acid (TFA)4 were used to add in eluent. It has been demonstrated that titania strongly trapped water-soluble organic phospholipids under acidic conditions.7,23 Ikeguchi and Nakamura added acetic acid in the loading solution to enhance the ability of titania to concentrate PLs before HPLC analysis.7 TFA was used by Calvano and co-workers to enrich PLs on TiO2 microcolumns.8 To better understand the retention mechanism of PLs on titania, the effect of buffer pH was studied in this section.
The retention factors of PC and glycerol monostearate as well as the resolution of PC in PC-50 basically remained unchanged when the eluent pH increased from pH 5.0 to 8.0 (Fig. S2†). Zhou and Lucy have also reported that the retention for the early eluted AMP3 and 1,2,3,4-benzenetetracarboxylate19 did not change a lot when buffer pH was changed. However, these results seem inconsistent with the previous observations that titania usually has a strong selective affinity to organo-phosphates at acidic conditions. Actually, it was confirmed in Zhou and Lucy's recent work that a certain amount of the pore volume of a titania phase is occupied by a water-enriched layer when high %ACN is present in the ACN–water eluent.3 Similarly, replacing water by MeOH makes the water layer change into a MeOH layer, which gave inspiration to our work.
The normal-phase chromatograph column of titania is different from the precolumns or tips packed by TiO2 particles and the retention mechanism of analytes is various. When MeOH and water are present in high %ACN eluent, a water–MeOH layer will probably be formed on the titania surface drawing amphoteric PC molecules close to the column surface. On this occasion, hydrophilic interaction and ligand exchange between the analyte and the column surface both take effect and they cooperate for the retention of PC on titania. However, the ligand exchange of PC on titania surface is rather weak when the strong elution buffer of phosphate is present in the mobile phase. Glycerol monostearate does not undergo ligand exchange or electrostatic interactions and prefers the void volume of the column which is not occupied by the water–MeOH layer due to its lipophilic nature. Hence, HILIC is the dominant retention mechanism for both PC and glycerol monostearate. What directly affects the retention of PC and glycerol monostearate is the thickness of the water–MeOH layer in HILIC mode, which is not seriously affected by the changes of eluent pH when the buffer concentration and %organic solvent are kept constant. So the retention did not change significantly with the change of buffer pH.
Buffer concentration
The effect of buffer concentration was shown in Table 1. It can be seen that the retention factor of PC decreased from 0.43 to 0.39 (9% decrease) when the concentrations of phosphate changed from 0 to 2.5 mM and a similar phenomenon was also found in the case of glycerol monostearate (15% decrease). The result was not in accordance with that reported by Zhou and Lucy that the retention of carboxylates increased with the increasing of phosphate buffer concentration.19 In their work, two possible causes have been hypothesized: firstly, there is a water-enriched layer formed on the surface of titania particles when the mobile phase contains high organic solvents. Higher buffer concentration might drive the solvated salt to the layer so that the water layer volume increases and therefore the retention time increases.19,20,24,25 Secondly, higher salt concentration weakens the electrostatic repulsion between the stationary phase and the analytes. Hence the retention time of analytes extended. In this study, however, the retention factors of PC and glycerol monostearate both decreased moderately when the buffer concentration increased. Although the results were similar for PC and glycerol monostearate, the explanations were different. In this study, as the eluent is a MeOH–ACN–water system with high %ACN, a water–MeOH layer would probably be formed on the titania surface. As lipophilic glycerol monostearate does not undergo ligand exchange or electrostatic interactions, the expansion of the water–MeOH layer on the titania surface caused by higher salt concentration might drive the more lipophilic glycerol monostearate to be excluded from the water–MeOH layer and make it elute a little faster by the mobile phase.
Concentration/mM |
k
|
Efficiency |
R
|
PC |
Glycerol monostearate |
PC |
Glycerol monostearate |
PC in PC-50 |
Conditions: flow rate, 1.0 mL min−1; eluent, MeOH–ACN–water = 10/75/15 (v/v/v), pH 6.0, buffer concentrations changed from 0 to 2.5 mM; analyte, 0.1 mg mL−1 PC, 4 mg mL−1 glycerol monostearate and 0.5 mg mL−1 PC-50 sample; column temperature, 40 °C; injection, 20 μL; UV detection at 205 nm.
|
0 |
0.43 |
0.39 |
6400 |
1500 |
2.7 |
1.5 |
0.42 |
0.38 |
6600 |
3700 |
1.8 |
2.5 |
0.39 |
0.33 |
6700 |
4500 |
1.8 |
It is a little more complicated in the case of PC. On one hand, higher salt concentration rendered the expansion of the water–MeOH layer on the titania surface, drawing more PC into the layer and hence resulting in an increase of PC retention. On the other hand, PC can undergo ligand exchange with the titania column while phosphates is the strongest eluent as demonstrated in the work of Zhou and Lucy.19 Once the mobile phase contains some phosphate buffer, PC would be eluted faster. Thus, the increase of phosphate buffer is a two-edged sword in this case. The two retention modes of hydrophilic interaction and ligand exchange cooperate to yield the final result.
As mentioned before, a suitable eluent condition to achieve a complete isolation of PC would be found. Considering that the resolution of PC in PC-50 (Table 1) hit a higher value when there was no buffer present in the eluent and meanwhile the efficiency for PC did not get significant improvement with the addition of phosphate buffers, the mobile phase without phosphate buffer was chosen in the further work. In addition, the study of the %ACN effect provides other evidence for this conclusion and it will be discussed in the following section.
%ACN
As can be seen from Fig. 2, there was poor improvement for the retentions of PC (8% increase) and glycerol monostearate (6% increase) when %ACN changed from 25 to 80 in phosphate buffer eluent. Nevertheless, when the mobile phase did not contain any phosphate buffer, the retention factors of PC dramatically changed (48% increase) at the range of 75%–80% ACN and the trend was different from glycerol monostearate retention behavior. As we stated before, HILIC is the dominant retention mechanism for both PC and glycerol monostearate when phosphate buffer is added to the eluent. Although the increase of %ACN could enhance the water–MeOH layer on the titania surface that may help to prolong retention time, the phosphate would replace PC to undergo ligand exchange with titania, so that the effect of %ACN on retention increase is weakened. Under the situation without no buffer present in eluent, the water–MeOH layer formed on the titania surface is enriched, drawing more PC molecules closer to the titania surface, increasing PC ligand exchange with titania and prolonging PC retention.
Here, the selective affinity of titania for organo-phosphates takes effect, yielding the result that PC retains much more strongly on titania column than glycerol monostearate. In fact, we can take advantage of such unique characteristic of titania to separate organo-phosphates from their analogues.
Meanwhile, the resolution of PC in PC-50 increased dramatically with the increase of %ACN, demonstrating that increasing %ACN can make it possible to separate PC and other components completely at no salt condition. Furthermore, the mixture of PC and glycerol monostearate was prepared and successfully isolated (R = 4.14) by the mobile phase of MeOH–ACN–water = 10/79/11 (v/v/v) (Fig. 3). This result proves that the titania chromatography column has the ability to simply separate phospholipids from nonphosphated lipids without any special extraction and enrichment.
Flow rate
The effect of flow rate on the retention and efficiency of PC was finally investigated. The retention factor of PC decreased from 0.71 to 0.41 (42% decrease) when the flow rate changed from 0.8 to 1.6 mL min−1 (Fig. S3†). At the same time, the efficiency decreased from 2300 to 1900 and a platform was presented between the range from 1.0 to 1.4 mL min−1. In order to find an appropriate flow rate, a resolution experiment was conducted (Fig. S3†). The result showed that a flow rate of 1.0 mL min−1 was appropriate, because at this point the efficiency and resolution were higher, and furthermore, no serious band broadening and peak tailing were observed which would occur at a flow rate of 0.8 mL min−1. So the further quantitative studies would be performed at aflow rate of 1.0 mL min−1, comprehensively considering the retention, efficiency, resolution and peak shape of PC.
Application
Separation of lecithin samples
Based on the investigations of eluent conditions made above, the separation of different lecithin samples was performed on a titania chromatography column. High %ACN eluent without any phosphate buffer was used to maintain relative strong retention and high resolution of PC. The %ACN in eluent should be strictly controlled since a tiny change in %ACN can dramatically influence the retention and resolution of PC. Finally, all the lecithin products were isolated at MeOH–ACN–water = 10/79/11(v/v/v), as 78% ACN can not provide enough resolution, while 80% ACN would result in long elution and peak broadening of PC. As shown in Fig. 4, PC was completely separated from other components in all the lecithin samples, with the resolutions (R) of PC all above 6.0. Separation and analysis of PC in lecithin samples were finished within 6 min without any step of pre-fraction and enrichment, making it a time-saving, simple and convenient method compared with similar studies performed by silica columns,6,7,9 which must be capable for the potential analysis of real-world lecithin samples.
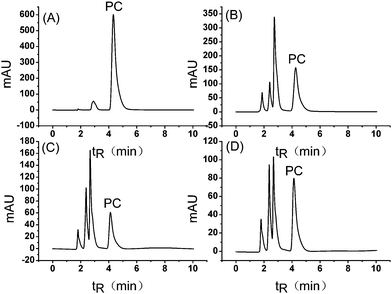 |
| Fig. 4 Chromatograms of PC standard and three kinds of lecithin samples on titania. Conditions: flow rate, 1.0 mL min−1; eluent, MeOH–ACN–water = 10/79/11(v/v/v), no buffer; analyte, (A) 1.0 mg mL−1 PC, (B) 0.5 mg mL−1 PC-50, (C) 1.0 mg mL−1 soybean lecithin oil and (D) 1.0 mg mL−1 ARSVICA capsules; column temperature, 40 °C; injection, 20 μL; UV detection at 205 nm. | |
Quantitative analysis
The PC in soybean phospholipids has been separated completely from other components within 6 min. PC quantitative analysis was achieved with an external standard method. The calibration curve was linear in the range of 0.1–1.0 mg mL−1 of PC and the linear equation is y = 13217x + 745.99 with a correlation coefficient (R2) of 0.9947. The contents of PC in five different soybean lecithin samples are shown in Table 2.
Table 2 Contents of PC in soybean lecithin products.a
Method precision and detection limit
To evaluate the HPLC method on titania, precision and detection limit studies were also performed. PC of 0.1 mg mL−1 and glycerol monostearate of 4 mg mL−1 were used and injected six times under the same conditions. The relative standard deviations (RSD) of PC and glycerol monostearate were 3.40% and 0.93%, respectively. The detection limits (S/N = 3) obtained were 0.2 μg for PC and 0.3 μg for glycerol monostearate, respectively. The result was satisfactory and demonstrated that analyzing PLs by this method was stable and credible.
Recovery
The aim of the analysis described in this section was to evaluate the performance of the method based on titania by determining the recovery of the PC standard from the PC-50 sample. Four different amounts of PC standard were added to 110 μL of 0.5 mg mL−1 of PC-50 sample solution (PC content was 26.61 μg) and then the mixture was diluted into 200 μL. Finally, the mixtures were determined by HPLC to get the test values. As can be seen from Table 3, the recovery of the method ranged from 91% to 101%, proving that the HPLC method was credible and accurate.
Table 3 Recovery of PC by HPLC method on titania columna
Initial/μg |
Added/μg |
Total/μg |
Found/μg |
Recovery (%) |
Conditions: flow rate, 1.0 mL min−1; eluent, MeOH–ACN–water = 10/79/11(v/v/v), no phosphate buffer was present; column temperature, 40 °C; injection, 20 μL; UV detection at 205 nm.
|
26.61 |
10.00 |
36.61 |
33.38 |
91 |
26.61 |
10.00 |
36.61 |
36.84 |
101 |
26.61 |
15.00 |
41.61 |
40.33 |
97 |
26.61 |
15.00 |
41.61 |
41.76 |
100 |
26.61 |
20.00 |
46.61 |
46.18 |
99 |
26.61 |
20.00 |
46.61 |
45.16 |
97 |
26.61 |
25.00 |
51.61 |
50.39 |
98 |
26.61 |
25.00 |
51.61 |
51.71 |
100 |
Conclusions
The factors that affect the retention behavior of PC on titania have been studied. The hydrophilic interaction and ligand exchange mechanism cooperate to retain PC on titania. High %ACN and no phosphate in the eluent are the keys to make such cooperation take place and provide appropriate retention and high resolution of PC. The influence of buffer pH and concentration on the retention behavior of PC was minor. Separation and analysis of PC in lecithin products by HPLC method on titania was accomplished within 6 min without any pre-treatment. The superiority of rapidity, simplicity, convenience, accuracy and credibility makes the HPLC method on titania a competent technique in the field of organo-phosphates analysis.
Acknowledgements
This work was supported by the National Natural Science Foundation of China (Grant No. 20875069) and the Science Foundation for Young Teachers of Tianjin University of Commerce (Grant No. 090107).
Notes and references
- Y. Chen, Y. Yi, J. D. Brennan and M. A. Brook, Chem. Mater., 2006, 18, 5326–5335 CrossRef CAS
.
- J. Konishi, K. Fujita, K. Nakanishi, K. Hirao, K. Morisato, S. Miyazaki and M. Ohira, J. Chromatogr., A, 2009, 1216, 7375–7383 CrossRef CAS
.
- T. Zhou and C. A. Lucy, J. Chromatogr., A, 2008, 1187, 87–93 CrossRef CAS
.
- H.-C. Hsieh, C. Sheu, F.-K. Shi and D.-T. Li, J. Chromatogr., A, 2007, 1165, 128–135 CrossRef CAS
.
- K. Hata, H. Morisaka, K. Hara, J. Mima, N. Yumoto, Y. Tatsu, M. Furuno, N. Ishizuka and M. Ueda, Anal. Biochem., 2006, 350, 292–297 CrossRef CAS
.
- Y. Ikeguchi and H. Nakamura, Anal. Sci., 1999, 15, 229–232 CAS
.
- Y. Ikeguchi and H. Nakamura, Anal. Sci., 2000, 16, 541–543 CAS
.
- C. D. Calvano, O. N. Jensen and C. G. Zambonin, Anal. Bioanal. Chem., 2009, 394, 1453–1461 CrossRef CAS
.
- Y. Wang, China Oil and Fat, 2008, 33, 73–75 Search PubMed
.
- H. Xia, H. An, Y. Liu and C. Yu, Chin. J. Anal. Chem., 2001, 29, 1046–1048
.
- A. Avalli and G. Contarini, J. Chromatogr., A, 2005, 1071, 185–190 CrossRef CAS
.
- K.-G. Zink and K. Mangelsdorf, Anal. Bioanal. Chem., 2004, 380, 798–812 CrossRef CAS
.
- J. S. Rhee and M. G. Shin, J. Am. Oil Chem. Soc., 1982, 59, 98–99 CrossRef CAS
.
- L. M. Rodríguez-Alcalá and J. Fontecha, J. Chromatogr., A, 2010, 1217, 3063–3066 CrossRef CAS
.
- D. M. Kariotoglou and S. K. Mastronicolis, Lipids, 2001, 36, 1255–1264 CrossRef CAS
.
- W. J. Hurst and R. A. Martin, Jr., J. Am. Oil Chem. Soc., 1984, 61, 1462–1463 CrossRef CAS
.
- A. A. Carelli, M. I. V. Brevedan and G. H. Crapiste, J. Am. Oil Chem. Soc., 1997, 74, 511–514 CrossRef CAS
.
- D. Adlercreutz and E. Wehtje, J. Am. Oil Chem. Soc., 2001, 78, 1007–1011 CrossRef CAS
.
- T. Zhou and C. A. Lucy, J. Chromatogr., A, 2010, 1217, 82–88 CrossRef CAS
.
- N. S. Quiming, N. L. Denola, Y. Saito, A. P. Catabay and K. Jinno, Chromatographia, 2008, 67, 507–515 CrossRef CAS
.
- J. Konishi, K. Fujita, K. Nakanishi, K. Hirao, K. Morisato, S. Miyazaki and M. Ohira, J. Chromatogr., A, 2009, 1216, 7375–7383 CrossRef CAS
.
- M. W. H. Pinkse, P. M. Uitto, M. J. Hilhorst, B. Ooms and A. J. R. Heck, Anal. Chem., 2004, 76, 3935–3943 CrossRef CAS
.
- Y. Ikeguchi and H. Nakamura, Anal. Sci., 1997, 13, 479–483 CrossRef CAS
.
- Y. Guo and S. Gaiki, J. Chromatogr., A, 2005, 1074, 71–80 CrossRef CAS
.
- M. Liu, E. X. Chen, R. Ji and D. Semin, J. Chromatogr., A, 2008, 1188, 255–263 CrossRef CAS
.
Footnote |
† Electronic supplementary information (ESI) available: Supplementary Fig. S1–S5. See DOI: 10.1039/c0ay00419g |
|
This journal is © The Royal Society of Chemistry 2010 |
Click here to see how this site uses Cookies. View our privacy policy here.