DOI:
10.1039/C0AY00382D
(Technical Note)
Anal. Methods, 2010,
2, 1614-1617
Influence of polyaniline on the potentiometric determination of risedronate with ion-selective membranes
Received
16th June 2010
, Accepted 22nd July 2010
First published on
18th August 2010
Abstract
A novel kind of potentiometric sensor for the determination of risedronate in pharmaceutical products is proposed. This potentiometric sensor is comprised of a poly(vinyl chloride) (PVC) membrane based on an anion-exchanger, tridodecylmethylammonium chloride (TDDMACl), and coated with a polyaniline (PANI) layer. The potentiometric response towards a highly hydrated risedronate species was strongly promoted by the presence of the PANI layer. In contrast to uncoated membranes, the PANI-coated membrane exhibited a sensitivity of −39.9 ± 0.9 mV decade−1 and the detection limit reached 0.45 mg mL−1. The results of the potentiometric analysis of risedronate agreed with those obtained with a validated chromatographic method. The TDDMACl-based membrane coated with the PANI layer differentiated 0.94 wt % and 3.44 wt % of a specific contaminant in 1.6 mg mL−1 risedronate.
Introduction
Risedronate sodium (Fig. 1A) belongs to a class of drugs called bisphosphonates that are widely used in the treatment and prevention of diseases associated with bone loss.1 The pharmacological function of these active compounds is determined by their P–C–P fragments, where two phosphate groups are covalently linked to a carbon atom. Analyses of bisphosphonates are usually done using high-performance liquid chromatography (HPLC) coupled with a variety of spectroscopic methods.2 Direct detection techniques with UV, fluorescence, or electrochemical detectors require the pre- and post-column derivatization of bisphosphonates. In contrast to other bisphosphonates, risedronate is neither natively fluorescent nor an electroactive compound.3 Moreover, risedronate cannot be readily derivatized. As a result, the pharmaceutical industry requires the development of analytical detection techniques with simple sample preparation and the possibility of automation for risedronate analysis. Due to the accelerated methodology development in the field of potentiometric sensors and growing demands of the pharmaceutical industry, potentiometry with ion-selective electrodes (ISEs) should be a method that fulfils the given requirements.4
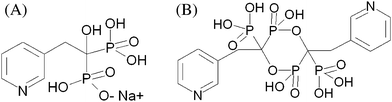 |
| Fig. 1 Chemical structures of sodium risedronate (A) and its specific contaminant (B). | |
An increasing amount of work is being done on preparing drug-selective electrodes.5 However, the traditional strategy based on exchange either an ion-exchanger or plasticizer into the poly(vinyl chloride) (PVC) matrix has been used for a long time. Unfortunately, such an approach is not always suitable for the determination of hydrophilic species.
We have recently shown that polyaniline (PANI) as an outer layer on a plasticized PVC membrane surface favoured the transport of highly hydrated sulfates from aqueous medium.6 The observed phenomenon resulted from stronger PANI hydration in the presence of highly hydrated anions, which facilitated their transport through the inter-particle space of the PANI layer.7 Here, PANI as a hydration-promoting medium is tested for the potentiometric detection of highly hydrated risedronate. This study evaluates the influence of PANI on the potentiometric determination of risedronate in both the model and in real samples. Moreover, we discuss the applicability of the proposed sensor to detect a specific contaminant (Fig. 1B) in risedronate.
Experimental
Materials
High-molecular-weight poly(vinyl chloride) (PVC), 2-nitrophenyl octyl ether (NPOE), tridodecylmethylammonium chloride (TDDMACl) and tetrahydrofuran (THF; stored over a molecular sieve) were purchased from Fluka (Selectophore, Switzerland). Aniline (>99%) was supplied by Sigma-Aldrich (Germany). Acids and various inorganic salts used in potentiometric measurements were of analytical grade from Lachema (Czech Republic). Ammonium dihydrogen phosphate (≥99.0%), tetrabutylammonium bromide (≥99.0%) and ethylenediaminetetraacetic acid disodium salt (Reag. Ph. Eur., 99–101%) were supplied by Fluka (Germany). Sodium hydroxide (98%) was purchased from Lach. Ner. (Czech Republic). HPLC-grade methanol was produced by J. T. Baker (Holland). The working standard of sodium risedronate (Fig. 1A), a specific contaminant of sodium risedronate (Fig. 1B) and tablets were obtained from Zentiva k.s. (Prague, Czech Republic). The analyzed tablets were composed of: microcrystalline cellulose, crospovidone, magnesium stearate, hypromellose, talc, macrogol 6000, titanium dioxide, ferric oxide red, ferric oxide yellow.
1 mg of TDDMACl, 33 mg of NPOE and 66 mg of PVC were dissolved in 1.0 mL THF at ambient temperature. To prepare 0.5 mm thick membranes, 1.0 mL of the solution was deposited onto a metallic ring with an internal diameter of 16 mm resting on a glass plate, and dried in air. The membranes were coated with the PANI layer according to the procedure described recently by Shishkanova et al.6
Potentiometric measurements
A 10 mm diameter disk was punched from the prepared membrane, glued to a polymeric ring-support with an 8 mm internal diameter using a PVC/THF mixture, and mounted in an electrode body for potentiometric measurements. An aqueous solution of 0.1 M Na2SO4 and 10−6 M NaCl was used as the electrode's inner filling solution. Before the potentiometric measurements, the PANI-coated membranes were immersed in 2 × 10−2 M NaOH for 10 min and then washed with distilled water. All measurements were carried out at the ambient temperature with cells of the following type:
Ag | AgCl | 3 M KCl | sample solution | membrane | inner filling solution | AgCl | Ag.
The electromotive force (ΔE) was measured with a PHI 04 MG digital ion-meter (Labio, Czech Republic) controlled by custom-made data-logging software programmed in LabView 6.1 (National Instruments, USA). The solvent used for the dissolution and dilution of all solutions used for the potentiometric measurements was HCl at pH 3.8 ± 0.1.
A stock solution of risedronate was prepared by dissolving 1.7332 g of working standard in a 100-mL volumetric flask. Risedronate calibration solutions ranging from 15.3 μg mL−1 to 15.3 mg mL−1 were prepared by appropriate serial dilution of stock solution.
The intra-day potentiometric precision (expressed as relative standard deviation, RSD) was evaluated by determination of risedronate in working standard solutions at two concentrations (1.41 and 14.1 mg mL−1) three times with experimental electrode within one day.
The stability of the potentiometric parameters was tested with prepared membranes over a period of one year. When not used for measurements, the membranes were stored in distilled water.
A 2 mg mL−1 solution containing a specific contaminant (Fig. 1 B) was mixed with pure sodium risedronate (1.6 mg mL−1) to yield various levels of contaminated risedronate samples.
A program found at http://www.molinspiration.com was used to calculate the log P values of anionic species.
HPLC measurements
HPLC experiments were run on an Agilent 1100 Series (Santa Clara, USA) with an Inertsil ODS 2 column (250 mm × 3.2 mm × 5 μm). The mobile phase consisted of 0.005 M ammonium dihydrogen phosphate, 0.002 M tetrabutylammonium bromide and 0.0017 M ethylenediaminetetraacetic acid disodium salt aqueous solution (pH 7.2) mixed with methanol in the ratio 75
:
25 (v/v). The column was equilibrated for 8 h. The flow rate of the mobile phase was set to 0.8 mL min−1. Detection wavelength was 262 nm, column temperature 40 °C and injected volume 20 μL. This method was validated. The standard solution was prepared as follows: 21.0 mg of working standard of sodium risedronate was dissolved in 5 mL of water and placed in an ultrasonic bath for 5 min. After cooling, the solution was diluted to 10 mL with distilled water. 1 mL of this solution was then diluted to 5 mL with the mobile phase. The standard solution was injected five times and the sample solution twice.
Analysis of active pharmaceutical ingredient
The content of risedronate in tablets was assayed using the proposed potentiometric sensor and the validated chromatographic method. To adequately assess the results obtained, both the chromatographic and potentiometric analysis were carried out with the same samples. The nominal content of risedronate in the analyzed tablets was 35 mg per tablet.
The pharmaceutical chemicals were processed according to the following procedure: Four tablets (1.1450 g) were finely ground in a small dish. The resulting powder (1.1111 g) was transferred to a 100-mL volumetric flask with 50 mL of HCl at pH 3.8 ± 0.1. The solution was sonicated for 30 min in an ultrasonic bath. After filtering, the filtrate was diluted to exactly 100 mL in a volumetric flask. To evaluate the matrix effect, potentiometric analysis was based on the calibration plot method and the standard addition method.8 The aliquots of the filtrate were then either diluted or spiked to obtain risedronate concentrations of 0.54 and 8.37 mg mL−1.
The HPLC analysis was based on the procedure described above (see section HPLC measurements).
Results and discussion
Because risedronate has two ionisable phosphate groups, its polarity is too strong and interface transfer is too problematic. The high hydrophilicity of risedronate is the main reason why it cannot be detected by a PVC membrane based on the anion-exchanger TDDMACl. We decided to take a step back from the traditional strategy of seeking acceptable plasticizers or replacing the TDDMACl for TDDMA – risedronate as the active membrane component. Our approach was to use a PANI layer deposed onto the PVC membrane as a hydration-promoting medium facilitating the transfer of highly hydrated risedronate at the membrane | aqueous electrolyte interface.
Potentiometric sensitivity, linearity and stability
The potentiometric response of an uncoated TDDMACl-based membrane toward risedronate was expected to be poor (Fig. 2 A, ■). In contrary to an uncoated TDDMACl-based membrane, the sensitivity of the PANI-coated membranes was dependent on pH. The optimum value for the potentiometric detection of risedronate was pH 3.8 ± 0.1 (Fig. 2 A). This choice was determined by the ionization of risedronic acid and the sensitivity of the potentiometric sensor. At pH 3.8, risedronic acid preferentially exists as mixture of mono- and dicharged anions (pKa1 = 1.35, pKa2 = 2.87, pKa3 = 7.03, pKa4 = 11.3).9 The potentiometric sensitivity is related to the charge of the determined analyte. Therefore the highest sensitivity and consequently better precision of the potentiometric determination was expected for mono- and dicharged ions. Coating the TDDMACl-based membrane with the PANI layer markedly increased its potentiometric response. The sensitivity of TDDMACl-based membranes coated with PANI towards risedronate was −39.9 ± 0.9 mV decade−1 within the concentration range from 1.53 mg mL−1 to 15.3 mg mL−1 and the detection limit reached 0.45 mg mL−1.
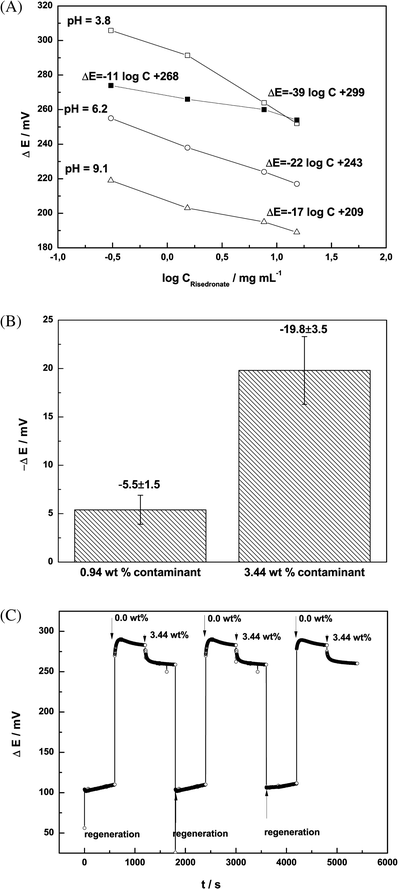 |
| Fig. 2 (A) pH dependence of the potentiometric response for an uncoated (■) and the PANI-coated (pH 3.8 (□), pH 6.2 (○), pH 9.1 (△)) TDDMACl-based membrane toward risedronate. (B) Changes in potentiometric signal observed for TDDMACl-based membranes coated with PANI layer with various ratios of risedronate and contaminant (n = 4). In these experiments ΔE was defined as a difference of electromotive force measured for untainted risedronate (ΔEuntainted risedronate) and measured for contaminated risedronate (ΔEcontaminated risedronate). Because of an addition of contaminant led to decreasing of electrode response, the resulting values of ΔE indicated the negative values (i.e., ΔE = ΔEuntainted risedronate – ΔEcontaminated risedronate). (C) Recordings of potentiometric signals observed for three experimental membranes with untainted 1.6 mg mL−1 risedronate (0.00 wt %) and contaminated risedronate (3.44 wt %). | |
Importantly, neither the sensitivity nor linear concentration range changed over a period of one year. These experimental findings indicate that the PANI layer is a beneficial addition to the surface of an anion-exchanger PVC-membrane, not only for detecting highly hydrated species, but also to maintain stable potentiometric parameters over time.
Potentiometric precision
The intra-day precision was tested with repeated measurements of working standard solutions at two concentrations (Table 1). Recoveries of 103.0–100.4 and a relative standard deviation ranging between 3.0 and 0.8% demonstrated that the electrodes are suitable for risedronate analysis. The potential variability did not exceed a few millivolts.
Table 1 Parameters of direct potentiometric determination of risedronate in working standards with the PANI layer coated TDDMACl - based membrane (n = 3)
Added/mg mL−1 |
Found/mg mL−1 |
RSD (%) |
Relative error (%) |
1.41 |
1.45 ± 0.11 |
3.0 |
2.8 |
14.10 |
14.15 ± 0.28 |
0.8 |
0.4 |
Potentiometric detection of a contaminant in risedronate
The ability of a TDDMACl-based membrane coated with a PANI layer to discriminate a contaminant in risedronate was tested. The specific contaminant of risedronate used was a dimer of risedronic acid (Fig. 1B). With the proposed potentiometric sensor, the discrimination should be based upon differences in their hydrophilicity. According to the calculated log P values, the active pharmaceutical ingredient and its contaminant have highly comparable and simultaneously poor lipophilicity, i.e. risedronate (log P = −4.829) and contaminant (log P = −5.151). As a point of comparison, phosphate, known to be a very hydrophilic anion has log P = −4.53, whereas chloride, known to be a lipophilic anion has log P = −2.145. Despite the very high hydratation energy of bisphoshponates, the TDDMACl-based membrane coated with the PANI layer differentiated 0.94 wt % and 3.44 wt % of the contaminant from 1.6 mg mL−1 sodium risedronate (Fig. 2B). The fact that the discrimination was obtained between very hydrophilic structural analogues can be explained by the selective accumulation of hydrophilic anionic species to the membrane | aqueous electrolyte interface. The similar accumulation leads to preconcentration of the highly hydrated anions by the outer PANI-layer at the interface and, as consequence, amplification of the potentiometric response.10 Importantly, the potentiometric signals recorded with three experimental membranes towards the untainted risedronate (0.00 wt %) and contaminated risedronate (3.44 wt %) were reproducible (Fig. 2C). The data presented here shows that the weight percent of contaminant in risedronate may be estimated using the calibration curve ΔE = f(log (weight percent contaminant)). To date, there has only been one study devoted to the development of a quantitative potentiometric test for contaminants (namely, oversulfated chondroitin sulfate) in heparin preparations. The basis of this detection was differences in charge density for contaminated heparin and for the untainted heparin.11 Our approach is interesting in that it offers a novel principle for discriminating between analytes based on minor differences in their hydrophilicity.
Application to real samples
In order to assess the specificity of the proposed method, potentiometric determinations of risedronate were performed on commercially available tablets. In parallel, the same samples were analyzed with a validated HPLC method. The results of these measurements are presented in Table 2. Importantly, the potentiometric analysis was applied without any prior sample treatment (neither separation nor derivatization). It should be born in mind that the final concentration of risedronate during the potentiometric detection should be in the linear concentration range or close to the detection limit of the electrode used. The good agreement between the results obtained from both methods demonstrated that the proposed electrode was sufficiently suitable and additives and excipients did not interfere with the quantification of risedronate in the tablets. Consequently potentiometric detection can be considered to be effective not only for model samples but also for real products.
Method |
Procedure |
Risedronate content/mg mL−1 |
n = 4.
n = 3.
|
HPLC |
Calibration plot |
0.54 |
1.01 |
8.37 |
Potentiometry with experimental ISEs |
Calibration plot |
0.49 ± 0.06 a |
0.99 ± 0.11 a |
7.92 ± 0.95 b |
Standard addition method |
— |
1.02 ± 0.06 a |
— |
Conclusion
In summary, we report a very simple and inexpensive screening potentiometric procedure for routine risedronate content verification. A novel approach might also be beneficial for the potentiometric detection of bisphosphonates not containing a chromophore group. Work on developing this strategy with other systems of pharmaceutical interest is underway.
Acknowledgements
This research was supported by the Ministry of Education, Youth, and Sports of the Czech Republic (MSM 6046137307). We thank Dr Martin Krondak for the data-logging software programmed in LabView6.1 (National Instruments, USA), used during the potentiometric measurements.
References
- J. E. Dunford, K. Thompson, F. P. Coxon, S. P. Luckman, F. M. Hahn, C. D. Poulter, F. H. Ebetino and M. J. Rogers, J. Pharmacol. Exp. Ther., 2001, 296, 235–242 CAS.
- C. K. Zacharis and P. D. Tzanavaras, J. Pharm. Biomed. Anal., 2008, 48, 483–496 CrossRef CAS.
- P. T. Vallano, S. B. Shugarts, W. F. Kline, E. J. Woolf and B. K. Matuszewski, J. Chromatogr., B: Anal. Technol. Biomed. Life Sci., 2003, 794, 23–33 CrossRef CAS.
- S. Dai, J. M. Esson, O. Lutze, N. Ramamurthy, V. C. Yang and M. E. Meyerhoff, Pharm. Sci & Tech. Today, 1999, 19, 1–14 Search PubMed; J. H. Yun, I. S. Han, L.-C. Chang, N. Ramamurthy, M. E. Meyerhoff and V. C. Yang, Pharm. Sci & Tech. Today, 1999, 2, 102–110 Search PubMed.
- K. Vytřas, J. Pharm. Biomed. Anal., 1989, 7, 789–812 CrossRef CAS; N. N. Golovnev, G. V. Novikova and I. I. Golovneva, Mikrosist. Tekh., 2001, 10, 15–17 Search PubMed; A. Salem, B. N. Barsoum and E. L. Izake, Anal. Chim. Acta, 2003, 498, 79–91 CrossRef CAS; M. Giahi, M. Arvand, M. Mirzaei and M. A. Bagherinia, Anal. Lett., 2009, 42, 870–880 CrossRef CAS.
- T. V. Shishkanova, D. Sykora, H. Vinšová, V. Král, I. Mihai and N. P. Gospodinova, Electroanalysis, 2009, 21, 2010–2013 CrossRef CAS.
- N. P. Gospodinova, D. A. Ivanov, D. V. Anokhin, I. Mihai, L. Vidal, S. Brun, J. Romanova and A. Tadjer, Macromol. Rapid Commun., 2009, 30, 29–33 CrossRef CAS.
-
J. Koryta and K. Štulik, Ion-selective electrodes, Academia, Prague, 1st edn, 1984, ch. 5, pp. 97–106 Search PubMed.
-
Bisphosphonates: Calcium Antiresorptive Agents, http://www.duc.auburn.edu/%7Ederuija/endo_bisphos.pdf (20/4/2010) Search PubMed.
- E. Bakker and E. Pretsch, TrAC, Trends Anal. Chem., 2008, 27, 612–618 CrossRef CAS.
- L. Wang, S. Buchanan and M. E. Meyerhoff, Anal. Chem., 2008, 80, 9845–9847 CrossRef CAS.
|
This journal is © The Royal Society of Chemistry 2010 |