DOI:
10.1039/C0AY00165A
(Paper)
Anal. Methods, 2010,
2, 1106-1111
An amperometric polyphenol biosensor based on polyvinyl chloride membrane
Received
10th March 2010
, Accepted 10th May 2010
First published on
4th June 2010
Abstract
A method is described for construction of a polyphenol biosensor employing polyvinylchloride membrane bound banana fruit polyphenol oxidase. The biosensor showed optimum response within 30 s, when operated at 0.4 V in 0.1 M sodium phosphate buffer, pH 7.5 and 35 °C. The detection limit of the method was 7.5 × 10−7 M. The analytical recovery of added L-DOPA was 93.50%. Within batch and between batch coefficients of variation were < 0.82% and < 1.65% respectively. The sensor was employed for amperometric determination of polyphenols in tea leaves, alcoholic beverages and water. The enzyme electrode was used 200 times over 60 days, when stored at 4 °C.
Introduction
Polyphenolic compounds are widespread in nature. They are important constituents of natural products such as plant leaves, fruits and vegetables and also of industrial waste.1 Many of these phenolic compounds have toxic effects on animals and plants of which they easily penetrate the skin and membranes, determining a broad spectrum of genotoxic, mutagenic and hepatotoxic effects and also affecting the biocatalysed reaction rates in respiration and photosynthesis.2–4 The detection of polyphenols is usually done by HPLC, spectrometry and GC-MS.5–12 However, these techniques are expensive, time-consuming, require costly reagents, several operations and separation steps and are thus tedious and cumbersome. Moreover, these techniques also lead to the emergence of large amount of effluents, which are not environmentally friendly.13 Nevertheless, immobilized enzyme based biosensors are comparatively more simple, sensitive and rapid. There are some reports regarding the Clark type of oxygen electrode and especially some tissue electrodes,1–3,14 but their detection limit was only 5–10 μmol/L. Few biosensors have been reported for detection of phenolic compounds based on polyphenol oxidase such as the amperometric biosensor based on a polyethersulfone membrane,15 ferric oxide nanoparticles and the chitosan nanocomposite based biosensor,16 the ZnO nanorod modified Au electrode17 and the electrodeposited polyaniline film.18 A general problems for many polyphenol biosensors was the lack of the necessary operational and storage stability needed for commercial exploitation, limited electron transport and complexity in the purification steps of the enzyme. Compared to commercial enzyme, purified enzymes from plant sources provide a cheaper and more stable alternative to be used for construction of polyphenol biosensors. The present report describes the construction of a comparatively more stable, reproducible and reliable amperometric polyphenol biosensor based on polyvinyl chloride (PVC) membrane bound banana fruit polyphenol oxidase (PPO). PVC membrane is highly stable, conducting, non fragile, resistant to chemicals and bacterial contamination.19
Materials and methods
Chemicals
3,4-Dihydroxy phenylalanine (L-DOPA) from HiMedia (Mumbai), glycerol and polyvinyl pyrrolidone (PVP) from SRL, Mumbai, polyvinyl chloride (PVC) and BSA from SIGMA Chemicals Co, USA, isopropyl myristate and tetrahydrofuran (THF) from Merck, Germany were used. Tea leaves and alcoholic beverages of various commercial brands were purchased from the local market. Different water samples from the well and canal from a nearby rural area of Rohtak and local tap water were used.
Extraction of PPO from banana fruit
Twenty-five grams of pulp of fresh ripened banana fruit was homogenized with 100 mL of 0.1 M phosphate buffer (pH 7.0) containing 2.5 g of PVP-360 as stabilizer, in a liquefier for 2 min at 4 °C. The suspension was filtered through a Whatman filter in a funnel on ice and centrifuged at 3000 rpm for 30 min at 4 °C. The supernatant (crude enzyme) was collected and stored at 4 °C until used.
Assay of PPO
The assay of PPO in crude extract was carried out by measuring the change in A475 in a UV visible spectrophotometer (Make: Shimadzu, Model 1700) due to conversion of DOPA into dopachrome. The reaction mixture containing 100 μL of crude enzyme and 3.0 ml of 10 mM L-DOPA solution in 0.1 M sodium phosphate buffer (pH 7.0) was incubated at 25 °C. One enzyme unit is defined as a change in A475 by 0.001/min/ml under the specified assay conditions.
The protein concentration in PPO preparations was determined by the Lowry20 method using bovine serum albumin as standard.
Preparation of PVC membrane
The PVC membrane was prepared as described by the method of Reddy et al.21 PVC powder (0.06 g) and 150 μl of isopropylmyristate were dissolved in 5 ml of tetrahydrofuran (THF). This polymer solution was poured into a glass Petri dish and was rotated horizontally onto a flat surface to get an even distribution of polymer over the Petri dish. The polymer solution was covered with a glass lid to allow slow and controlled evaporation of the solvent and was kept overnight. Two pieces of 2 × 2 cm2 dimensions were cut from this PVC membrane of even thickness (0.2 mm) with a pair of scissors and stored at 4 °C until use.
Immobilization of polyphenol oxidase onto the PVC membrane
Polyphenol oxidase was immobilized onto the PVC membrane through physisorption after BSA glutaraldehyde cross linking.22 Polyphenol oxidase (40 U) and BSA (50 mg) were dissolved in 250 μl distilled water. 25 μl of this enzyme solution and 12.5 μl of 2.5% (w/v) of glutaraldehyde in distilled water were mixed rapidly and placed on a 4 cm2 portion of PVC membrane (Inner membrane). A further 4 cm2 portion of PVC membrane (Outer membrane) was then placed on the enzyme membrane and two glass slides were used to compress both the membrane under mild finger pressure for 5 min. The resulting laminate was then washed with buffer solution (0.1 M sodium phosphate buffer, pH 7.0)
The PVC membrane with and without enzyme was subjected to scanning electron microscopy (SEM) at ‘Sophisticated Analytical Instrumentation Facility’ (DST), AIIMS, New Delhi to confirm the immobilization.
Construction and response measurement of amperometric polyphenol biosensor
An amperometric biosensor for measurement of polyphenols was prepared by mounting PVC membrane bound PPO onto a Pt electrode (diameter 2 mm and length 2 cm) with parafilm to construct a working electrode. This working electrode along with Ag/AgCl as reference and Cu wire as auxiliary electrode were connected to a three terminal electrometer (Make: Keithley Japan Model 6517A/E). To test the activity of biosensor, all three electrodes were dipped into a 25 ml beaker containing 4 ml reaction mixture [1.0 ml of 10 mM L-DOPA and 3.0 ml of 0.1 M sodium phosphate buffer pH 7.0] and kept in a temperature controlled water bath. The electrodes were polarized at different potential (in volts) and current (mA) was measured in electrometer. The current was also measured at varying concentration of L-DOPA in a sodium phosphate buffer (0.1 M, pH 7.0). The working principle of this biosensor is as follows. L-DOPA is oxidized into its corresponding o-quinone by the PVC membrane bound PPO. Then L-DOPA was regenerated through electrochemical reduction of o-quinone, thus forming a bioelectrocatalytic amplification cycle and thereby generating electrons, which were passed to the Pt electrode from solution through pores of the PVC membrane23 (Fig. 1).
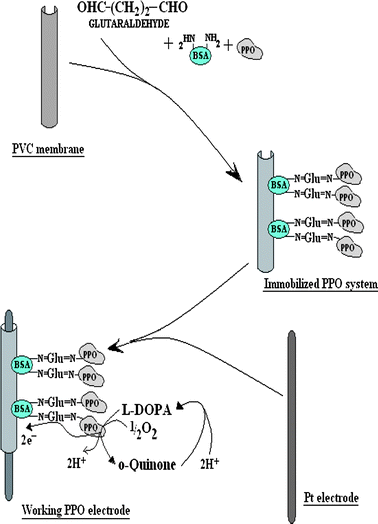 |
| Fig. 1 Scheme of chemical reactions involved in immobilization of polyphenol oxidase (PPO) onto polyvinyl chloride (PVC) membrane after bovine serum albumin (BSA)–glutaraldehyde (Glu) crosslinking and response measurement of the enzyme electrode. | |
Study of kinetic properties of immobilized PPO
Various kinetic properties of the PVC membrane bound enzyme such as optimum pH, incubation temperature, response time and effect of substrate (L-DOPA) concentration were studied amperometrically to determine the optimum working conditions of the biosensor.
Amperometric determination of polyphenols in tea, alcoholic beverages and waste water samples
The biosensor was employed for determination of polyphenols in five commercial brands of tea leaves, alcoholic beverages and four water samples. Samples of tea leaves were prepared by boiling 5 g of dried tea leaves into 20 ml of distilled water and filtering it through a sieve. Polyphenol level in tea leaves, alcoholic beverages and water samples was determined by the biosensor in the same manner as described above for its response measurement under its optimal working conditions except that L-DOPA was replaced by these sample. The current (mA) was recorded and the amount of polyphenols was extrapolated from standard curve between L-DOPA concentrations and current (mA) prepared under optimal working conditions.
Results and discussion
Immobilization of polyphenol oxidase onto the PVC membrane
Polyphenol oxidase was extracted from ripened banana fruit and immobilized onto the PVC membrane through BSA–glutaraldehyde cross-linking. One –CHO group of glutaraldehyde linked to the –NH2 group of the enzyme and another –CHO group bound to the –NH2 group of BSA which provided a more physical stable complex than attained by physical aggregation (Fig. 1). In addition, BSA was used to reinforce the compact ester structure of enzymes leading to increased size and stability which prevented leakage of enzymes from the pores of the laminated PVC membrane. SEM of PVC membrane bound to PPO shows the big clusters of immobilized enzyme which were absent in the PVC membrane alone (Fig. 2), confirming the immobilization of enzyme.
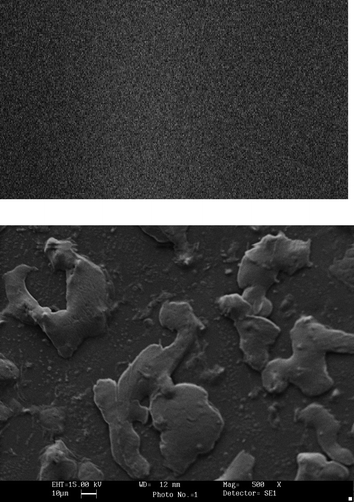 |
| Fig. 2 SEM pictures of (top) a polyvinyl chloride (PVC) membrane and (bottom) polyphenol oxidases immobilized on the PVC membrane at magnification of 500×. | |
Construction of the polyphenol biosensor
A method is described for construction of an amperometric polyphenol biosensor based on the PVC membrane bound PPO. As the current generated was maximum at +0.4V, it was selected for standardization of working conditions of the sensor for the polyphenol biosensor. The optimum working conditions of this biosensor were as follows:
Optimum pH
The effect of pH of reaction buffer on the biosensor response was studied in the range pH 6.0 to 8.5 using 0.1 M sodium phosphate buffer. The biosensor response was increased as the pH was increased up to pH 7.5 after which it started decreasing (Fig. 3). The optimum pH of the immobilized enzyme (pH 7.5) was higher than that of the free form (pH 7.0) but also comparable to that immobilized on different supports, e.g. nafion membrane (pH 6.8),23 gelatin24 and polypyrrole layer [pH 7.0].25
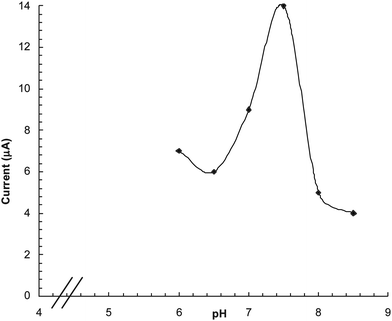 |
| Fig. 3 Effect of pH on response of polyphenol biosensor based PVC bound polyphenol oxidase. | |
Optimum incubation temperature
The optimum temperature for the biosensor was 35 °C (Fig. 4) which is higher than that of the free enzyme (30 °C) and that immobilized onto the gelatin and glutaraldehyde (2.5%) bound polyphenol oxidase24 but lower than that immobilized onto the polypyrrole layer.25 The sensor showed an optimum response at 30 s (Fig. 5), hence in subsequent studies the current was measured within this time.
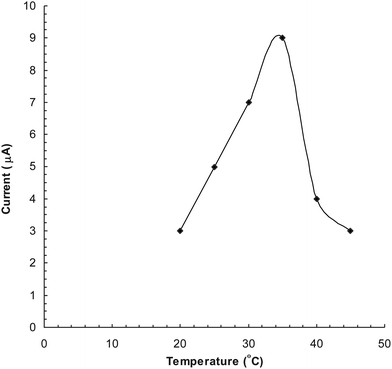 |
| Fig. 4 Effect of temperature on response of polyphenol biosensor based PVC bound polyphenol oxidase. | |
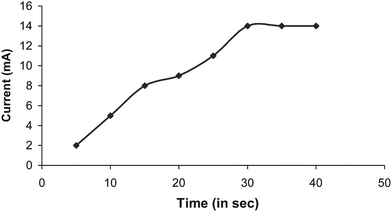 |
| Fig. 5 Time course study for response measurement of polyphenol biosensor based PVC bound polyphenol oxidase. | |
Effect of substrate concentration
To study the effects of substrate concentration on biosensor response, the concentration of L-DOPA was varied from 1.25 μM to 20 μM. A hyperbolic relationship was observed between L-DOPA concentration range versus current (in μA) (Fig. 6). Km for L-DOPA, as calculated from the Lineweaver–Burke plot, was 55.5 μM (Fig. 7), which is lower than that of the Eastman and nafion membrane bound enzyme (257 and 170 μM respectively).23 Imax was 25 μA min−1 ml−1, which is higher than that of earlier ones.23 The following criteria were studied to evaluate the performance of this biosensor:
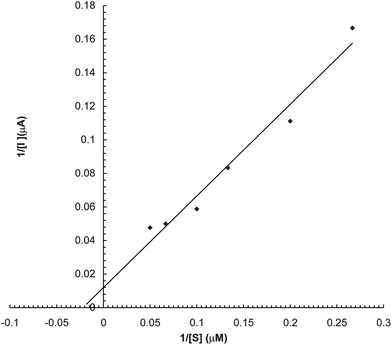 |
| Fig. 7 Lineweaver–Burke plot for the effect of L-DOPA conc. on response of polyphenol biosensor based on PVC bound enzyme. | |
Linearity
There was a linear relationship between L-DOPA concentration ranging from 1.25 × 10−6 M to 1 × 10−5 M and current (mA) (Fig. 6), which is better than the earlier biosensor based on polyethersulfone membrane [9.09 × 10−6–138 × 10−6 M]15 and artificial membrane [1 × 10−2–8 × 10−3M]26 but comparable to those based on Fe3O4 MNPs coated carbon nanotube nanocomposites on a glassy carbon electrode (1 × 10−8–3.9 × 10−5M)27 and gelatin membrane (1–20 μM)28 and higher than that of ferric oxide nanoparticles and the chitosan nanocomposite bound enzyme (8.3 × 10−8–7 × 10−5M).17
Detection limit
The detection limit of the biosensor was 7.5 × 10−7 M, which is comparable to earlier biosensors (6.23 × 10−7 M)17 but higher than ferric oxide nanoparticles and the chitosan nanocomposite bound enzyme sensor (2.5 × 10−8 M)27 and the nafion membrane bound tyrosinase (1.5 × 10−8 M).
Recovery
The % recovery of added L-DOPA in tea leaf extract (2.5 μM and 5 μM) by the present sensor was 86.48 ± 0.35 and 93.50 ± 0.28 respectively (Table 1).
Table 1 Analytical recovery of added L-DOPA in the tea, as measured by the polyphenol biosensor based on PVC membrane bound polyphenol oxidase
L-DOPA Added (μM) |
Polyphenols Found (μM) |
% Recovery |
— |
1.2 |
— |
2.5 |
3.2 |
86.37 ± 0.35 |
5 |
5.8 |
93.50 ± 0.28 |
Precision
To check the reproducibility and reliability of the present method, the polyphenol in the five tea samples was estimated six times on a single day (within batch) and again after storage at −20 °C for one week (between batch). The results showed that determinations were consistent and within and between coefficient of variance (CV) were <0.82% and <1.65% respectively (Table 2) showing the good reproducibility and reliability of the method.
Table 2 Within and between assay coefficients of variation (CV) for determination of polyphenols in the tea samples as measured by the polyphenol biosensor based on PVC membrane bound polyphenol oxidase
(n) |
L-DOPA (μM) |
CV (%) |
Within assay (5) |
1.21 ± 0.01 |
0.82 |
Between assay (5) |
1.21 ± 0.02 |
1.65 |
Reusability and storage
The enzyme electrode was reused 200 times over a period of 180 days when stored at 4–8 °C in glycerol solution 50% (v/v) (Fig. 8). This stability of the PVC membrane bound enzyme was higher than earlier sensors.29–32
A comparison of kinetic properties of various membrane based polyphenol biosensors is summarized in Table 3 which shows the superiority of the present PVC membrane based biosensor over other biosensors regarding sensitivity, stability and response time.
Table 3 A comparison of various membrane based polyphenol biosensors
Parameter |
Nistor et al., 1999 |
Climent et al., 2001 |
Abhijith et al. 2007 |
Tembe et al. 2008 |
Present |
Mode of measurement |
Current |
Current |
Dissolved O2 |
Current |
Current |
Support for Immobilization |
Nafion membrane |
Polyethersulfone membrane |
Cellophane membrane |
Eggshell membrane |
PVC membrane |
Method of Immobilization |
Entrapment |
Adsorption |
Cross-linking |
Cross-linking |
Physisorption |
Optimum pH |
4.9 |
7.0 |
7.0 |
5.0–6.5 |
7.5 |
Optimum temp. |
NR |
25 °C |
30 °C |
20–45 °C |
35 °C |
Minimum detection limit |
1.5 × 10−8 M |
9.09 × 10−6 M |
1 × 10−2 M |
2.5 × 10−5M |
7.5 × 10−7M |
Linearity |
3 × 10−8–1 × 10−4 M |
9.09 × 10−6 M–138 × 10−6 M |
1 × 10−2–8 × 10−3 M |
5 × 10−5 M–25 × 10−5 M |
1.25 × 10−6 M–2 × 10−5 M |
Stability |
42 days |
37 days |
30 days |
6 months |
2 months |
Km |
17.5 × 10−5 M |
NR |
NR |
67 × 10−5 M |
5.55 × 10−5 M |
Response time |
1 min. |
2 min. |
5 min. |
1 min. |
30 s |
Determination of polyphenols in tea leaves, alcoholic beverages and water samples
Polyphenol level as measured by the present sensor ranged from 4–8.4 μM in tea leaves, 1.9–3.0 in alcoholic beverages and 0.7–7.6 μM in waste water samples (Table 4).
Table 4 Polyphenol level in different brands of tea, alcoholic beverages and water samples as measured by the polyphenol biosensor based on PVC membrane bound polyphenol oxidase
Biological materials |
Polyphenol level (mM) |
Tea |
a) Tata tea |
4.9 |
b) Red label |
4.9 |
c) Darjelling |
4.7 |
d) Taj |
4.0 |
e) Local tea |
8.4 |
2. Alcoholic Beverages |
a) Vodka |
2.9 |
b) Royal challenge |
2.5 |
c) Beer |
3.0 |
d) Whisky |
2.2 |
e) Local |
1.9 |
3. Water |
|
a) Well |
0.076 |
b) Canal |
0.060 |
c) Tap |
0.079 |
d) Distilled |
0 |
Conclusion
A method is described for a PVC membrane based polyphenol biosensor with improved sensitivity, stability and response time compared to other membrane based biosensors.
References
-
D. Barcelo in Environmental Analysis-Techniques, Applications and Quality Assurance, Elsevier, Amsterdam, Ed., 1993 Search PubMed.
- M. V. Reddy, G. R. Blackburn, C. A. Schreiner, M. A. Mehlman and C. R. Makerer, Environ. Health Perspect., 1989, 82, 253–258 CrossRef CAS.
- J. W. Yager, D. A. Eastmond, M. L. Robertson, W. M. Paradisina and M. T. Smith, Cancer Res., 1990, 15, 393–399.
- J. I. Brodfuehrer, D. E. Chapman, T. J. Wilkea and G. Powis, Drug Metab. Dispos. 18, 1990, 18, 20–27 Search PubMed.
- F. Bosch, G. Font and J. Mares, Analyst, 1987, 112, 1335–1337 RSC.
- W. A. MacCrehan and J. M. Brown-Thomas, Anal. Chem., 1987, 59, 477–479 CrossRef CAS.
- E. Delage, G. Bohuon, A. Baron and J.-F. Drilleau, J. Chromatogr., A, 1991, 555, 125–136 CrossRef CAS.
- T. A. Berger and J. F. Deye, J. Chromatogr. Sci., 1991, 29, 54–59 CAS.
- C. Brage and K. Sjöström, J. Chromatogr., A, 1991, 538, 303–310 CrossRef CAS.
- Y. Hoshika and N. Murayam, Analyst, 1983, 108, 984–990 RSC.
- F. Mazzei, F. Botrwe, M. Lanzi, G. Lorenti, F. Procelli and C. Botre, Sens. Actuators, B, 1992, 7, 427–430 CrossRef.
- D. M. Goldberg, E. Tsang, A. Karumanchiri, E. P. Diamemdis, G. Someas and E. Ng, Anal. Chem., 1996, 68, 1688–1694 CrossRef CAS.
- S. A. S. S. Gommes and M. J. F. Rebelo, Sensors, 2003, 3, 166–175 CrossRef.
- S. G. Burton, Catal. Today, 1994, 22, 459–487 CrossRef CAS.
- P. V. Climent, M. L. M. Serralheiro and M. J. F. Rebelo, Pure Appl. Chem., 2001, 73, 1993–1999 CrossRef CAS.
- S. Wang, Y. Tan, D. Zhao and G. Liu, Biosens. Bioelectron., 2008, 23, 1781–1787 CrossRef CAS.
- B. X. Gu, C. X. Xu, G. P. Zhu, S. Q. Liu, L. Y. Chen and X. S. Li, J. Phys. Chem. B, 2009, 113, 377–381 CrossRef CAS.
- Y. Tan, X. Guo, J. Zhanga and J. Kan, Biosens. Bioelectron., 2010, 25, 1681–1687 CrossRef CAS.
- J. Narang, M. Bhambi, Minakshi and C. S. Pundir, Anal. Lett., 2010, 43, 1–10 CrossRef CAS.
-
O. H. Lowry, N. J. Rosebrough, A. L. Farr and R. J. Randall, 1951, 193, pp. 265–275.
- R. R. Reddy, C. Anju and B. Enakshi, Biosens. Bioelectron., 2001, 16, 313–317 CrossRef CAS.
- I. Ben Rejeb, F. Arduini, A. Amine, M. Gargouri and G. Palleschi, Anal. Chim. Acta, 2007, 594, 1–8 CrossRef.
- C. Nistor, J. Emnéus, L. Gorton and A. Ciacu, Anal. Chim. Acta, 1999, 387, 309–326 CrossRef CAS.
- E. Akyllmaz, S. H. Baysal and E. DinÇkaya, Int. J. Environ. Anal. Chem., 2007, 87, 755–761 CrossRef.
- A. E. Böyükbayram, S. Kıralp, L. Toppare and Y. Yağ, Bioelectrochemistry, 2006, 69, 164–171 CrossRef.
- K. S. Abhijith, P. V. Sujith Kumar, M. A. Kumar and M. S. Thakur, Anal. Bioanal. Chem., 2007, 389, 2227–2234 CrossRef CAS.
- Y. Cheng, Y. Liu, J. Huang, K. Li, Y. Xian, W. Zhang and L. Jin, Electrochim. Acta, 2009, 54, 2588–2594 CrossRef CAS.
- E. Asav, E. Yorganci and E. Akyilmaz, Talanta, 2009, 78, 553–556 CrossRef CAS.
- Y. D. de Albuquerque and L. F. Ferreira, Anal. Chim. Acta, 2007, 596, 210–221 CrossRef CAS.
- N. Li, M. H. Xue, H. Yao and J. J. Zhu, Anal. Bioanal. Chem., 2005, 383, 1127–1132 CrossRef CAS.
- J. Li, L. S. Chia, N. K. Goh and S. N. Tan, Anal. Chim. Acta, 1998, 362, 203–211 CrossRef CAS.
- P. Dantoni, S. H. P. Serrano, A. M. Oliveira Brett and I. G. R. Gutz, Anal. Chim. Acta, 1998, 366, 137–145 CrossRef CAS.
|
This journal is © The Royal Society of Chemistry 2010 |
Click here to see how this site uses Cookies. View our privacy policy here.