DOI:
10.1039/B9AY00328B
(Paper)
Anal. Methods, 2010,
2, 1101-1105
Received
29th December 2009
, Accepted 23rd April 2010
First published on
28th May 2010
Abstract
An acid induced mixed-micelle mediated cloud point extraction procedure is developed for the separation and pre-concentration of platinum from digested road dust matrices. The mixed-micelle is formed from the micelle–micelle interaction of cetyltrimethylammonium bromide (CTAB) (cationic) and Triton X-100 (nonionic) surfactants. Nitric acid induces the clouding as well as phase separation in these mixed-micelles. In the presence of mixed acids (HCl and HNO3), platinum forms stable and extractable anionic species compared to palladium and rhodium, which provides the quantitative separation of platinum, from palladium and rhodium, by forming the hydrophobic ion-associated complex with quaternary ammonium head groups of mixed-micelles. This hydrophobic complex is subsequently pre-concentrated from a bulk aqueous phase into a small mixed-micelles rich phase, avoiding the addition of an external chelating agent. The potential oxide interference of Hf is eliminated effectively up to 1 mg L−1 level, enabling interference free determination of 195Pt using inductively coupled plasma mass spectrometry (ICP-MS). The parameters affecting the extraction process are optimized. Under the optimized conditions, the pre-concentration factor and detection limit are 13 and 10 pg g−1, respectively. The recoveries are in the range 92–99% at 100–200 pg mL−1 with relative standard deviation 2.0–3.5%. The accuracy of the procedure is evaluated by platinum determination in certified reference materials such as CCRMP PTC-1a Copper-Nickel sulfide concentrate and BCR 723 Road dust and the results agreed with the certified values with 95% confidence level, as well as being applied to road dust samples.
Introduction
Recently platinum has become a new anthropogenic pollutant because of its major emission from the catalytic converters fitted in automobiles.1–3 Moreover, the analysis of road dust matrices indicates its first emission levels.4–6 Its adverse effects on human health necessitate developing methods for its determination. Highly sensitive inductively coupled plasma mass spectrometry (ICP-MS) is used for platinum determination in road dust. However, this analysis is hampered by the presence of low levels of platinum and high levels of interfering elements.7,8 To alleviate these problems, cationic and anionic exchangers and tellurium precipitation have been used most frequently prior to its determination by ICP-MS.9–11 These methods are cumbersome and time consuming.
Over the last several years, cloud point extraction (CPE) has been recognized as a simultaneous matrix separation and analyte pre-concentration procedure using hydrophobic solubilizing sites of nonionic micelles.12–15 Only a few CPE procedures have been reported for the determination of precious metals16–21 and no procedure to the best of our knowledge has been reported without using a chelating agent. This is mainly due to the lack of hydrophilic solubilizing sites in the extracting nonionic micelles. Recently, the introduced mixed-micelles concept in CPE has been reported to overcome this limitation partially. Most of the reported mixed-micelles have been formed from the interaction of ionic monomers, below critical micelles concentration (CMC) with nonionic micelles and exhibit both hydrophobic and limited hydrophilic solubilizing properties through synergism. However, the limited hydrophilic solubilizing sites in mixed-micelles have been so far used for the extraction of bulky metal ions from aqueous solutions.22–28 To extract bare hydrophilic metal ions directly from aqueous solution the number of hydrophilic solubilizing sites need to be increased. This can be achieved by the addition of ionic surfactant above its CMC. However, it is expected that the cloud point temperature (CPT) of the system will increase due to the repulsions within and between ionic micelles and mixed-micelles and cause difficulty in achieving the clouding and phase separation process.29 These limitations prevent the analytical applicability of the mixed-micelles formed from the micelle-micelle interaction of ionic and nonionic surfactants. Hence, it is necessary to overcome these limitations to effectively utilize the hydrophilic solubilizing sites in the mixed-micelles, which are formed between the interactions of ionic micelles with nonionic micelles.
In this work, for the first time, we described an acid induced cloud point extraction of ultra trace levels of platinum using mixed-micelles formed by the interactions of Triton X-100 with cetyltrimethylammonium bromide (CTAB) micelles. The cloud point phase separation in this micelle–micelle interacting system has been induced by the addition of nitric acid. The stable hydrophilic anionic platinum species formed in HCl and HNO3 mixture react selectively with the positively charged ammonium head group of the mixed-micelles and forms the hydrophobic ion-associates. These are subsequently pre-concentrated into a small mixed-micelle rich phase, avoiding the addition of an external chelating agent. The accuracy of the procedure is verified by analyzing certified reference materials such as CCRMP PTC-1a Copper-Nickel sulfide concentrate and BCR 723 Road dust using ICP-MS.
Experimental
Instrumentation
Platinum concentrations in the dissolved mixed-micelle rich phases at ultra trace levels were determined by using an ICP-MS (VG Elemental Plasma Quad 3, Winsford, Cheshire, U.K) with a Meinhard concentric nebulizer with a Scott-type spray chamber. The optimized operating conditions are given in Table 1. All the matrices were digested using a CEM MARS (CEM, Matthews, NC, USA) microwave digester using a HP1500 vessel.
Table 1 Optimized operating parameters of ICP-MS
Plasma conditions |
|
Rf power/W |
1350 |
Coolant gas flow/L min−1 |
13.4 |
Auxiliary gas flow/L min−1 |
0.9 |
Nebulizer gas flow/L min−1 |
1.1 |
Sample uptake rate/mL min−1 |
0.6 |
Sampler cone, Ni/mm |
1 |
Skimmer cone, Ni/mm |
0.7 |
Mass spectrometer setting |
|
Analytical mass |
195Pt |
Internal standard |
115In |
Dwell time/ms |
100 |
Number of replicates |
3 |
Auto lens |
On |
Scanning mode |
Peak jump |
Reagents and standard solutions
All reagents were of analytical reagent grade (Merck, Darmstadt, Germany) unless otherwise stated. Sub-boiled HCl and HNO3 were prepared in our laboratory using quartz stills. Ultrapure water (18.2 MΩ cm) obtained from a Milli-Q water purification system (Millipore, Bedford, MA, USA) was used to prepare all the solutions. Suprapur grade hydrofluoric acid was used. The individual surfactant solutions of 10% m/v were prepared in water using Triton X-100 and CTAB (Sigma-Aldrich, Steinheim, Germany). Pt(IV) and Pd(II) stock solutions 1 mg mL−1 were prepared by dissolving the metals in minimum amount of aqua regia and evaporated to near dryness and finally taken in 10% HCl. Rh(III) stock solution (1 mg mL−1) was purchased from high-purity standards (Charleston, SC, USA). The working standards were prepared by subsequent dilutions of stocks. Indium (1 ng mL−1) prepared from 10 μg mL−1 stock solution was used as an internal standard. Methanol was used for dissolving the mixed-micelle rich phase. Stock standard solutions (1 mg mL−1) of analytes such as Na+, Cu2+, Ca+2, Mg+2, Ni2+, Co2+, Hg2+, Bi 3+, Hf4+, F−, PO43−, and SO42− used for interference study were prepared using their respective salts. All the polypropylene containers were cleaned with a mixture of 10% v/v nitric and hydrochloric acids and then with Milli-Q water. To minimize contamination risk, experiments were performed in a class-100 clean room. The road dust samples were collected in urban and rural areas.
About 200–500 mg of road dust samples and certified reference materials were weighed accurately and transferred into pre-cleaned high pressure Teflon vessels. To this 4 mL aqua regia and 2 mL HF were added and followed the procedure as described in an earlier work,18 except the evaporation step. The digested matrices were made up to 50 mL with Milli-Q water. Procedural blanks were also prepared in a similar manner.
Aliquots of 2–5 mL digested matrices were taken in cleaned polypropylene centrifuge tubes of 15 mL size. To these solutions, 0.5 mL of 10% m/v CTAB and 2.5 mL of 10% m/v Triton X-100 were added and made up to 10 mL using Milli-Q water. The solutions were mixed well for 5 min and then heated at 90 °C. After 20 min heating gravitational phase separation was obtained. The mixed-micelle rich phase was easily separated by decanting the bulk aqueous phase after cooling the solution in a “freezer at −4 °C” for 5 min. To reduce the viscosity, the mixed-micelle rich phase was dissolved in 1 mL of water containing 5% methanol and analyzed for platinum using ICP-MS. Procedural blanks were also prepared in a similar manner.
Results and discussion
Clouding phenomenon in cationic micelle and nonionic micelle interacting system
In nonionic surfactants clouding phenomenon is commonly observed, but it is very difficult in ionic surfactants because of the strong electrostatic repulsions between the charged head groups.30 Moreover, the clouding and phase separation can be achieved in charged micelles either by screening the electrostatic repulsion or by increasing the hydrophobicity with additives, that are reflected from the changes observed in their CPT.13,31 Therefore, the changes in CPT of Triton X-100 micelles with CTAB monomer and micelles were studied in presence of additives such as HCl and HNO3. Fig. 1 shows the CPT variation of 1% m/v Triton X-100 micelles with CTAB in the concentration range of 0–1% m/v in presence of 5 and 10% v/v HCl. Afterwards a similar study was made using 5% HCl with 5 and 10% HNO3, respectively using 1 and 2.5% Triton X-100. The results showed that the CPT of Triton X-100 in HCl with and without CTAB was the same up to CMC (0.035%), where only monomers were present. This indicates that the mixed-micelles formed by interaction of CTAB monomers and Triton X-100 micelles do not change the hydrophobic to hydrophilic character of Triton X-100 and hence maintained the constant CPT. Just above the CMC of CTAB, the CPT of Triton X-100 increased to 90 and 96 °C, respectively with 5 and 10% HCl at 0.12% CTAB. Above this concentration no clouding and phase separation was observed. This is mainly due to the strong repulsions within and between cationic micelles and mixed-micelles, which restricted mixed-micelles aggregation and its analytical applicability in the CPE procedure. Then the individual and mixed effect of HNO3 and Triton X-100 was studied in presence and absence of 5% HCl. The CPT of 1% and 2.5% Triton X-100 was almost constant up to 0.7% CTAB and a CPT of 82, and 78; and 60 and 58 °C were obtained respectively, with 5, and 10% HNO3 in presence of 5% HCl. Further increase in CTAB concentration caused increase with 5% HNO3 and decrease in CPT in case of 10% HNO3. The constant CPT up to 0.7% CTAB with HNO3 indicates that addition of CTAB did not change the hydrophobic and hydrophilic characters of mixed-micelles formed from either monomers or micelles of CTAB with Triton X-100 micelles. This is achieved mainly due to the effective interaction of nitrate ions with the positive head group of the mixed-micelles, which reduced significantly the repulsions between the positive head groups within and between the cationic micelles and mixed-micelles.32 The reduction in the CPT of mixed-micelles formed in presence of 2.5% Triton X-100 when compared to 1% Triton X-100 with CTAB in presence of HNO3 is due to increases in their hydrophobic nature. In the absence of HNO3, no phase separation was observed even in presence of 2.5% Triton X-100 with CTAB (>0.12%) and a minimum 0.5%, (v/v) HNO3 is necessary to achieve it. Hence, the addition of HNO3 favors the micelle–micelle interaction and induces the clouding and phase separation at low temperature compared to HCl alone.
 |
| Fig. 1 Cloud point temperature of nonionic micelles Triton X-100 (TX-100) and mixed-micelles formed from interaction of cationic monomer (<0.035% m/v CTAB) and micelles (>0.035% m/v CTAB) with nonionic micelles in presence of additives such as HCl and HNO3. | |
It is a well known fact that platinum group metals form stable anionic complexes with the chloride ions that prevents its complexation with other ligands.17,33 Hence, in the present procedure the extraction efficiency of mixed-micelles obtained from the monomer–micelles and micelle–micelle interaction of CTAB and Triton X-100 were studied. It was found that the micelle–micelle interaction is favorable for the quantitative extraction of platinum species in presence of mixed acids (HCl and HNO3). The parameters affecting the extraction process were studied and optimized by using 200 pg mL−1 Pt, Pd and Rh.
The type of acids used and its concentration play a very important role not only for clouding and phase separation in the mixed-micelles, but also in the dissolution of sample matrices as well as the formation of stable and selective extractable species. As discussed earlier (Fig. 1), with HCl alone, no phase separation was observed, which was achieved in presence of minimum 0.5% HNO3 alone added to higher concentrations of CTAB (>0.12%) in presence of Triton X-100 (2.5%). Hence, the effect of HNO3 and combined effect of 0.5% and 5% HNO3 with HCl and HF on the recoveries of Pt, Pd and Rh were studied in the range 0–10% v/v acids in presence of 0.5% m/v CTAB and 2.5% m/v Triton X-100. The results are shown in Fig. 2. The results showed that with HNO3, the recovery of Pt, Pd and Rh were 50, 25 and 10%, respectively. It indicates that HNO3 alone is insufficient to extract Pt, Pd and Rh species quantitatively. Hence, the combined effect of various HNO3 concentrations (0.5% and 5% shown in Fig. 2) with HCl and HF was studied. The recovery of Pt increased from 45 and 50 to 95% when HCl (>0.3% and >3%) was added to 0.5 and 5% HNO3 whereas the recovery of Pd and Rh was increased from 20 to 40 and 10 to 12%, respectively. It indicates quantitative extraction of Pt in presence of Pd and Rh, when the concentration ratio of HNO3 to HCl was in between 0.5 to 1.66 and the total acids concentration maintained at less than 15%. Therefore, a mixture of 5% v/v HCl and HNO3 was selected; hence the microwave digested solution can directly be used without the need for drying. The effect of HF was also studied in presence of 5% HCl and 5% HNO3. The results showed that the recovery of Pt was quantitative in the concentration range 0–10% HF.
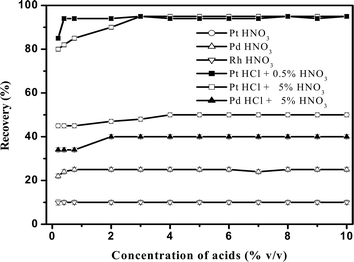 |
| Fig. 2 Effect of acids on the recovery of Pt, Pd and Rh in presence of 0.5% m/v CTAB, 2.5% m/v Triton X-100 and heated at 90 °C. The error bars indicate the standard deviation at each measurement (n = 4). | |
The concentrations of CTAB and Triton X-100 surfactants used in this study were optimized. Fig. 3 shows the effect of CTAB concentration in the range of 0–0.8% m/v on the recovery of platinum in presence of 2.5% m/v Triton X-100, 5% v/v HCl and HNO3. The results indicated that without CTAB, the clouding system acts as a nonionic surfactant based CPE and showed 5% recovery. By addition of CTAB up to CMC (0.035%) the monomers were responsible for the formation of mixed-micelles and showed only 25–30% recovery. This recovery was increased further by an increase in the concentration of CTAB above CMC and reached 95% at 0.3% concentration. By further increasing the concentration of CTAB, the recovery was constant up to a studied concentration (0.8%) of CTAB. The lower recovery at 0% CTAB is mainly due to the lack of electrostatic interacting sites in the extracting nonionic micelles. Quantitative recovery of platinum was obtained only with mixed-micelles formed from the micelles–micelles interaction of CTAB and Triton X-100. Hence, 0.5% m/v CTAB in the plateau region was selected.
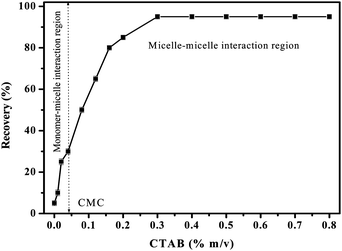 |
| Fig. 3 Effect of CTAB concentration on the recovery of Pt in presence of 5% v/v HCl and HNO3, 2.5% m/v Triton X-100 and heated at 90 °C. The error bars indicate the standard deviation at each measurement (n = 4). | |
The variation of platinum recovery with Triton X-100 in 0–4% m/v range, at 0.5% m/v CTAB was also studied. Without Triton X-100, only cationic micelles were present in the solution, which shows no clouding and phase separation because of the strong repulsion between the charged head groups. For achieving the clear cloud point phase separation, a minimum of 1% Triton X-100 was required in presence of 0.5% CTAB. At 1.5% Triton X-100, platinum recovery was 90%. The recovery increased with increase of Triton X-100 concentration up to 2%, and then reached the plateau region up to 4%. Hence, a 2.5% m/v Triton X-100 was selected.
Optimization of incubation temperature and time is necessary to study the ion-associated kinetics of inert platinum with mixed-micelles and their effective transfer into the micelles aggregates. Therefore, the effect of incubation temperature on recovery of platinum was studied in the range 70–100 °C (Fig. 1) using 30 min incubation time. The clouding temperature of the solution under optimized conditions was found to be 80 °C, whereas maximum extraction efficiency was achieved after 85 °C. Therefore, a 90 °C incubation temperature was selected. The optimization of incubation time was carried out between 10 to 120 min. The extraction of platinum is fast and efficient in all studied time intervals possibly due to the fast reactivity of stable anionic platinum species with positive mixed-micelles and stability of its ion-associates. Therefore, 20 min incubation time was selected. The amount of surfactants, incubation temperature and time decides the phase volume ratio (PVR) and pre-concentration factor (PCF). The PVR is the ratio of the final volume of the mixed-micelle rich phase to that of the aqueous phase and PCF is the ratio of the analyte concentration in the mixed-micelle rich phase to that of the initial aqueous phase. Under these conditions, the PVR and PCF were found to be 0.08 and 13, respectively.
Interferences study
In order to evaluate the performance of this procedure to various natural environmental matrices, the highest tolerability of various common interfering ions were studied along with Hf that interfere with 195Pt as 179Hf16O in ICP-MS measurements. The highest tolerable limits of various foreign ions spiked with 200 pg mL−1 Pt, by keeping the relative error between ±5% were found to be 1 g L−1 of Na+; 600 mg L−1 of Ca2+ and Mg2+; 200 mg L−1 of Cu2+ and Ni2+; 20 mg L−1 of Co2+ and Hg2+ and 2 mg L−1 of Bi3+ and 0.4 g L−1 of SO42−; 2 mg L−1 of F− and PO43−. In addition to the above, no interference was observed from Hf up to a concentration of 1 mg L−1 on 195Pt.
Analytical figures of merit
Under the optimized experimental conditions, the calibration curve was obtained by pre-concentrating the successively spiked ten standards of platinum in the range of 100–50,000 pg mL−1, respectively. The correlation coefficient was 0.9994. Quantifications have been performed by external calibration using aqueous standards prepared in 0.2% HCl. The limit of detection (LOD) calculated based on three times the standard deviation of ten measurements of procedural blanks was 10 pg g−1. The advantages of the proposed procedure were compared with the recently published nonionic surfactant based CPE procedures.16–19 The analytical capability of hydrophilic solubilizing sites of the mixed-micelles formed by the interaction of cationic micelles with nonionic micelles has been used effectively for the quantitative extraction of platinum. The mixed-micelles act as extracting agent for the extraction of platinum and avoid the addition of external chelating agents. Nitric acid induces the phase separation in the micelle–micelle interacting system and provides the quantitative extraction of platinum in presence of palladium and rhodium. The LOD obtained by the present procedure is comparable to an earlier reported procedure.17,18 This method also avoided the time consuming drying step commonly used in the microwave digestion procedure. Thus, the proposed procedure will be useful for determination of platinum in road dust over a wide concentration from sub-ppb to ppm range.
Validation of the procedure and analysis of real samples
The recovery studies of platinum were performed by spiking the digested PTC-1a Copper-Nickel sulfide concentrate and road dust sample collected from rural area in the range of 100–200 pg mL−1. The recoveries obtained are given in Table 2. The recoveries were between 92–99% in all studied matrices, which indicated that the studied matrices have no significant effect on the recoveries of platinum.
Table 2 Recovery of Pt from digest matrices using proposed procedure
Matrices |
Spiked/pg mL−1 |
Found/pg mL−1 (mean ± SD)a |
Recovery (%) |
Mean of four determination ± standard deviation.
|
Road dust sample rural area |
100 |
97 ± 3 |
97.0 ± 3. 1 |
200 |
197 ± 5 |
98.5 ± 2.5 |
PTC-1a Copper- Nickel sulfide concentrate |
100 |
92 ± 3 |
92.0 ± 3.5 |
200 |
193 ± 4 |
96.5 ± 2.0 |
The accuracy of the proposed procedure was validated by analyzing certified reference materials such as CCRMP PTC-1a Copper-Nickel sulfide and BCR 723 Road dust after microwave digestion. The values obtained are in good agreement with certified values based on student t-test at 95% confidence level. This procedure has also been applied to road dust samples along with certified reference materials and the results are given in Table 3. The platinum concentration obtained by the present procedure in real and certified reference materials were compared with the results obtained by reported CPE using the chelating agent. These results shows that there is a good agreement in the real as well as in certified reference material by both the procedures. The concentration of platinum in road dust sample obtained from urban area was approximately twice that of the sample collected from rural area, which indicates the anthropogenic affect of the catalytic converters.
Table 3 Analytical results for the determination of platinum in certified reference materials and real samples using the proposed mixed-micelle CPE procedure
Matrices |
Measured values/ng g−1 (mean ± ats(n)−1/2) |
Mixed-micelle-CPE |
Nonionic-CPE b |
Certified value/ng g−1 |
t
0.95 = 3.18, n = 4 and s = standard deviation.
Values are obtained from nonionic CPE procedure with chelating agent ref. 18.
Values are in μg g−1.
|
PTC-1a Copper-Nickel sulfide concentrate c |
2.76 ± 0.11 |
2.73 ± 0.13 |
2.72 ± 0.11 |
BCR 723 Road dust |
82.2 ± 1.7 |
83.0 ± 2.1 |
81.3 ± 3.3 |
Road dust sample rural area |
36.6 ± 1.9 |
37.5 ± 2.4 |
— |
Road dust sample urban area |
71.9 ± 2.4 |
72.7 ± 1.9 |
— |
Conclusions
A simple acid induced mixed-micelle CPE procedure has been demonstrated for the separation and pre-concentration of platinum from road dust prior to its determination by ICP-MS. Nitric acid induces not only the clouding and phase separation in the mixed-micelles formed by micelle–micelles interaction of CTAB and Triton X-100 surfactants, but also achieved the quantitative extraction of platinum in the presence of other platinum group metals. These mixed-micelles acts as extracting agent and avoids the addition of an external chelating agent commonly used in nonionic surfactant based CPE.
Acknowledgements
The authors are thankful to Dr J. Arunachalam, Head, CCCM, for his keen interest and consistent encouragement during this work and National Science Council (NSC) of the Republic of China for providing the PDF grant to Dr N. N. Meeravali.
References
- L. Bencs, K. Ravindra and R. V. Grieken, Spectrochim. Acta, Part B, 2003, 58, 1723–1755 CrossRef.
- V. F. Hodge and M. O. Stallard, Environ. Sci. Technol., 1986, 20, 1058–1060 CAS.
- R. R. Barefoot, Environ. Sci. Technol., 1997, 31, 309–314 CrossRef CAS.
- C. Wei and G. M. Morrison, Anal. Chim. Acta, 1994, 284, 587–592 CrossRef.
- P. Schramel, I. Wendler and S. Lusting, Fresenius J. Anal. Chem., 1995, 353, 115–118 CrossRef CAS.
- G. Kollensperger, S. Hann and G. Stingeder, J. Anal. At. Spectrom., 2000, 15, 1553–1557 RSC.
- L. A. Simpson, M. Thomsen, B. J. Alloway and A. Parker, J. Anal. At. Spectrom., 2001, 16, 1375–1380 RSC.
- M. Krachler, A. Alimonti, F. Petrucci, K. J. Irgolic, F. Forastiere and S. Caroli, Anal. Chim. Acta, 1998, 363, 1–10 CrossRef CAS.
- J. C. Ely, C. R. Neal, J. A. O'Neill Jr. and J. C. Jain, Chem. Geol., 1999, 157, 219–234 CrossRef CAS.
- D. G. Pearson and S. J. Woodland, Chem. Geol., 2000, 165, 87–107 CrossRef CAS.
- Z. Li and Y. Feng, J. Anal. At. Spectrom., 2006, 21, 90–93 RSC.
- R. P. Frankewich and W. L. Hinze, Anal. Chem., 1994, 66, 944–954 CrossRef CAS.
- S. Rubio and D. P. Bendito, Trends Anal. Chem., 2003, 22, 470–485 CrossRef CAS.
- C. D. Stalikas, Trends Anal. Chem., 2002, 21, 343–355 CrossRef CAS.
- C. B. Ojeda and F. S. Rojas, Anal. Bioanal. Chem., 2009, 394, 759–782 CrossRef CAS.
- M. A. M. da Silva, V. L. A. Frescure and A. J. Curtius, Spectrochim. Acta, Part B, 2001, 56, 1941–1949 CrossRef.
- K. Simitchiev, V. Stefanova, V. Kmetov, G. Andreev, N. Kovachev and A. Canals, J. Anal. At. Spectrom., 2008, 23, 717–726 RSC.
- N. N. Meeravali and S. J. Jiang, J. Anal. At. Spectrom., 2008, 23, 854–860 RSC.
- D. Rekha, P. R. Prasad and P. Chiranjeevi, Environ. Monit. Assess., 2007 DOI:10.1007/s10661-007-9837-5.
- K. Simitchiev, V. Stefanova, V. Kmetov, G. Andreev, A. Sanchez and A. Canals, Talanta, 2008, 77, 889–896 CrossRef CAS.
- M. Niemela, S. M. Huttunen, S. S. Gornostayev and P. Peramaki, Microchim. Acta, 2009, 166, 255–260 CrossRef.
- M. B. Gholivand, A. Babakhanian and E. Rafiee, Talanta, 2008, 76, 503–508 CrossRef CAS.
- N. Pourreza and M. Zareian, J. Hazard. Mater., 2009, 165, 1124–1127 CrossRef CAS.
- N. N. Meeravali and S. J. Jiang, J. Anal. At. Spectrom., 2008, 23, 1365–1371 RSC.
- T. Madrakian, A. Afkhami and A. Mousavi, Talanta, 2007, 71, 610–614 CrossRef CAS.
- E. K. Paleologos, A. G. Vlessidis, M. I. Karayannis and N. P. Evmiridis, Anal. Chim. Acta, 2003, 477, 223–231 CrossRef CAS.
- A. R. Zarei, Anal. Biochem., 2007, 369, 161–167 CrossRef CAS.
- D. L. Giokas, J. Antelo, E. K. Paleologos, F. Arce and M. I. Karayannis, J. Environ. Monit., 2002, 4, 505–510 Search PubMed.
- N. N. Meeravali and S. J. Jiang, J. Anal. At. Spectrom., 2008, 23, 555–560 RSC.
- T. Gu and P. A. G. Gomez, Colloids Surf., A, 1995, 104, 307–312 CrossRef CAS.
- W. N. Maclay, J. Colloid Sci., 1956, 11, 272–285 CrossRef CAS.
- J. Marra, J. Phys. Chem., 1986, 90, 2145–2150 CrossRef CAS.
- M. Balcerzak, Analyst, 1997, 122, 67R–74R RSC.
|
This journal is © The Royal Society of Chemistry 2010 |