DOI:
10.1039/C0AY00150C
(Paper)
Anal. Methods, 2010,
2, 1095-1100
Received
2nd March 2010
, Accepted 10th May 2010
First published on
10th June 2010
Abstract
Electrochemical behavior of nitrobenzene (NB) at a bismuth-film modified carbon paste electrode (BiF/CPE) in the presence of cetyltrimethylammonium bromide (CTAB) was investigated by square wave voltammetry. The electrochemical response of NB was apparently improved by CTAB due to the enhanced accumulation of NB at the electrode surface. Operational parameters including the deposition potential and time of bismuth film, pH of solutions and concentration of CTAB were investigated and optimized. Under optimized conditions, the cathodic peak current was proportional to the concentration of NB in the range of 1.0 × 10−6 to 1.0 × 10−4 mol L−1 with the detection limit of 8.3 × 10−7 mol L−1. The proposed BiF/CPE revealed good stability and reproducibility for rapid, simple and sensitive analysis of NB.
1. Introduction
Nitrobenzene (NB) is a priority pollutant and widely exists in water, soil and sediments from a variety of industrial activities. Even at low concentrations, it presents high risk to the environment and poses a great threat to human health. If the concentration of NB in waste exceeds 2 mg L−1, the waste can be declared hazardous.1–3 So the degradation and detection of NB are significant in public security and environmental protection, which also promote the development of detecting techniques for NB. Several techniques have been developed for the determination of NB, including high-performance liquid chromatography,4 gas chromatography,5 and UV-visible spectrophotometry.6 However, expensive equipment, time-consuming and complicated operations restrict their extensive application. Therefore, a sensitive, rapid and cheap method for the analysis of NB is in high demand.
Electrochemical methods have long been recognized as powerful techniques for inorganic and organic compound detection because they are highly sensitive, cheap, simple and convenient.7–9 Cathodic electrochemical detection of inorganic as well as organic compounds at different electrodes has been studied extensively, essentially at mercury electrodes (e.g. mercury-film electrode, hanging mercury drop electrode, and dropping mercury electrode).10 Nevertheless, due to the toxicity of mercury, its use seems to be constrained. Since the year 2000, bismuth-film electrodes (BiFEs), as an attractive alternative to the mercury electrode, have been employed in anodic or potentiometric stripping analysis for trace metal ions (e.g. the determination of Cd(II), Pb(II), Zn(II), Tl(I), In(III) by anodic stripping voltammetry and Cr(VI), Ni(II), Co(II) by adsorptive cathodic stripping voltammetry).11–14 Furthermore, due to the high hydrogen evolution overpotential, the bismuth electrode has been used for cathodic electrochemiluminescence studies in aqueous solutions.15 However, few reports have been focused on the application of BiFE in the cathodic electrochemical determination of organic compounds.16–18
Surfactants are a kind of amphiphilic ions or molecules with a hydrophilic head compatible with water on one side and long hydrophobic tail compatible with oil on the other side, which have been widely used in electrochemistry and electroanalytical chemistry.19–21 It is well known that surfactants play an important role in electrode reactions, not only in solubilizing organic compounds but also in providing specific orientation of the molecules at the electrode interface. These molecules give rise to adsorbed layers of varying thickness monolayers, bilayers or multilayers of a complex structure, which may alter the overvoltage of electrode and influence the rate of electron transfer.22–24 For example, Hu's group has successfully employed surfactants for the analysis of some biomolecules in their works.25,26 Arancibia et al. have reported the effect of sodium dodecyl sulfate on the sensitivity of the determination of lead in the presence of morin-5′-sulfonic acid with adsorption stripping voltammetry.27 Recently, the enhancement of cetyltrimethylammonium bromide (CTAB) in the electrochemical determination of chlorogenic acid has also been reported.28 These results indicate that the electrochemical responses of analytes were remarkably enhanced by surfactants.
In this work, a simple, rapid and sensitive electrochemical procedure for the determination of NB at an ex-situ plated bismuth-film modified carbon paste electrode (BiF/CPE) in the presence of CTAB was proposed. The electrochemical behavior of NB at BiF/CPE in the presence of CTAB was investigated by square wave voltammetry (SWV) and linear sweep voltammetry. The proposed method was applied to the determination of NB in tap water.
2. Experimental
2.1. Apparatus
All the electrochemical measurements were performed with a CHI 842B electrochemical workstation (Shanghai, China) in a conventional three-electrode configuration. The BiF/CPE was employed as the working electrode (i.d. 3.0 mm). A platinum wire and a saturated calomel electrode (SCE) were used as the auxiliary electrode and the reference electrode, respectively.
2.2. Reagent and solutions
NB, CTAB, and graphite power (spectroscopic grade) were purchased from Sinopharm Chemical Reagent Co. Ltd. (Shanghai, China). Paraffin oil was obtained from Sangon Co. Ltd. (Shanghai, China). Unless otherwise stated, other reagents were of analytical grade and used as received. Doubly-distilled water was used throughout the experiments. A 0.10 mol L−1 stock solution of NB was prepared by dissolving appropriate nitrobenzene in anhydrous ethanol. The Bi(III) stock solution was prepared by dissolving Bi(NO3)3·5H2O in 0.5% (v/v) HNO3. 0.10 mol L−1 phosphate buffer solution (PBS, pH 5.5) prepared from KH2PO4 and K2HPO4·3H2O was used as the supporting electrolyte.
A carbon paste electrode (CPE) was prepared by thoroughly hand-mixing graphite powder with paraffin oil at a ratio of 3.5
:
1 (w/w) in an agate mortar. The mixture was homogenized for 30 min and then firmly packed into a glass tube (i.d. 3.0 mm) as a piston-driven carbon paste electrode holder. The electric contact was established via a copper rod. Prior to use, the surface of CPE was smoothed on a piece of weighing paper. The new surface can be obtained by extruding ca. 0.5 mm of carbon paste out of the electrode holder and smoothed with a piece of weighing paper.
Bismuth-film was formed by ex-situ deposition on the prepared CPE. The ex-situ preparation of the bismuth-film was performed in an acetate buffer solution (0.10 mol L−1, pH 4.5), in the presence of dissolved oxygen, containing 5.0 mg L−1 of Bi(III) at −1.0 V vs. SCE for 60 s while stirring the plating solution. Once the bismuth-film was plated, the electrode was gently rinsed with doubly-distilled water for 5 s and placed in the fresh measurement solution. To remove the bismuth film, an electrochemical step was carried out by applying a potential of +0.5 V for 15 s in an acidic solution of 0.5 mol L−1 HNO3, after which the deposition of a fresh film was performed.
2.4. Electrochemical determination of NB
Voltammetric determinations of NB were carried out in 0.10 mol L−1 PBS (pH 5.5) containing 2 × 10−5 mol L−1 CTAB and appropriate amount of NB. After 30 s open-circuit accumulation, SWV curve was recorded in the potential range from −0.4 to −1.0 V at BiF/CPE with an increment potential of 0.004 V, an amplitude of 0.025 V and a frequency of 15 Hz. All the measurements were carried out at room temperature.
3. Results and discussion
3.1. Electrochemical behavior of NB at BiF/CPE and CPE
SWV was employed in the potential range from −0.4 to −1.0 V for investigating the electrochemical behavior of NB. Square wave voltammograms of NB at BiF/CPE and bare CPE in 0.10 mol L−1 PBS with and without 2.0 × 10−5 mol L−1 CTAB are shown in Fig. 1. As illustrated in Fig. 1, the BiF/CPE (curve A) exhibits a lower background signal than that of bare CPE (curve C) in the negative potential range. When 1.0 × 10−4 mol L−1 NB was added into the buffer solution, a well-defined SWV peak was obtained at both BiF/CPE (curve E) and bare CPE (curve D). According to the literature,29 the cathodic peak arose from the reduction of the nitro group of NB. However, the peak current of NB at BiF/CPE was 80% higher than that observed at bare CPE, and the peak potential shifted to a less negative value. These results suggest that the electrochemical reactions of NB at both electrodes are probably similar, while the electron transfer kinetics of NB on BiF/CPE was higher than that on bare CPE.30
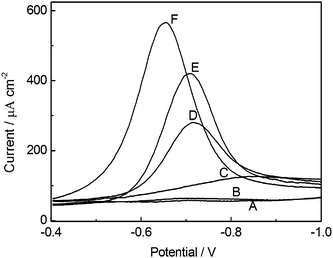 |
| Fig. 1 SWV response of NB in 0.10 mol L−1 PBS (pH 5.5). A) 0 mol L−1 CTAB at BiF/CPE; B) 2.0 × 10−5 mol L−1 CTAB at BiF/CPE; C) 0 mol L−1 CTAB at CPE; D) 1.0 × 10−4 mol L−1 NB at CPE; E) 1.0 × 10−4 mol L−1 NB at BiF/CPE; F) 1.0 × 10−4 mol L−1 NB + 2.0 × 10−5 mol L−1 CTAB at BiF/CPE. SWV parameters: increment potential 0.004 V; amplitude 0.025 V; frequency 15 Hz; quiet time 30 s. Bismuth plating conditions: 0.10 mol L−1 acetate buffer (pH 4.5) containing 5.0 mg L−1 Bi(III) at −1.0 V during 60 s. | |
Moreover, it is apparent that the peak current of NB at BiF/CPE (curve F) was improved when CTAB was added into the buffer solution. The peak current of NB at BiF/CPE, in the presence of 2.0 × 10−5 mol L−1 CTAB, was 43% higher than that obtained in the absence of CTAB, and the cathodic peak potential was 160 mV less negative than that obtained in the absence of CTAB. This may be attributed to the adsorption of the surfactant onto electrode surface, which changes the overpotential of the electrode and influences the electron transfer rate.31 The results indicate that CTAB plays an important role in the electrochemical reduction of NB and can improve the sensitivity for voltammetric determination of NB at BiF/CPE.
3.2. Optimization of the preparation of the BiF/CPE
BiFEs ex-situ deposited from a separate plating solution have found a wide range of applications in direct cathodic electrochemical determination because of its wide negative potential window and good electrochemical behavior (low background, high hydrogen overpotential, limited oxygen interference).11,32 For better application in voltammetric determination of NB using BiF/CPE, parameters influencing the quality of bismuth film were carefully optimized by varying the deposition time and deposition potential for bismuth film preparation in 0.10 mol L−1 acetate buffer (pH 4.5) containing 5.0 mg L−1 Bi(III). The results are shown in Fig. 2. The deposition potential was examined in the potential window between −0.4 and −1.4 V with 60 s deposition time. As can be seen from Fig. 2a, SWV peak current of NB gradually increased while the deposition potential shifting from −0.4 to −1.0 V, whereas at more negative deposition potentials the signal attenuated. The reason may be that at more negative potential the process of Bi(III) reduction is faster and the obtained film is generally thicker, more non-adhesive and mechanically unstable, often without contact to the support.17 And the effect of deposition time under a deposition potential of −1.0 V was also investigated as another important parameter influencing the quality of the bismuth film, showing an increase in SWV peak currents up to 60 s and a decrease for longer deposition time (Fig. 2b). At longer deposition time, a decrease of the signal was probably due to a thicker bismuth film which is usually less stable accompanied with its lower conductivity.30 Thus, the preparation of bismuth film was conducted at −1.0 V for 60 s in 0.10 mol L−1 acetate buffer (pH 4.5). Under these conditions, the BiF/CPE exhibited favorably electrochemical performance for NB determination.
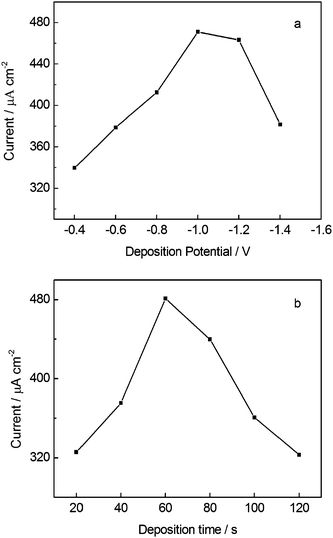 |
| Fig. 2 Effect of plating conditions of bismuth film on the peak current of NB. (a) deposition potential with 60 s deposition time, (b) deposition time with the deposition potential at −1.0 V. Other conditions are the same as in Fig. 1. | |
3.3. Effect of pH on the voltammetric response of NB
The effect of pH on the peak current and peak potential of NB at BiF/CPE in the presence of 2.0 × 10−5 mol L−1 CTAB was investigated in the pH range from 4.0 to 8.0. As can be seen in the Fig. 3, the peak potential (Epc) for NB became more negative with the increase of pH in a roughly linear manner. The plot of Epcversus pH was linear with the equation of best fit being: −Epc = 0.46 + 0.030 pH (R = 0.9973). This indicates that the electrochemical process involves proton transfer and the concentration of proton affects the rate of reaction.33
 |
| Fig. 3 Influence of pH on the peak current (●) and peak potential (○) of 1.0 × 10−4 mol L−1 NB at BiF/CPE in the presence of 2.0 × 10−5 mol L−1 CTAB. Other conditions are the same as in Fig. 1. | |
The effect of pH on the peak current is also demonstrated in Fig. 3. It was found that the peak current of NB gradually increased with the increase of pH from 4.0 to 5.5 and attained a maximum value at pH 5.5. Nevertheless, the current of NB remarkably decreased when pH was further increased. Therefore, a phosphate buffer of pH 5.5 was chosen as the supporting electrolyte for the subsequent experiments.
3.4. Effect of CTAB on the peak current of NB
The electrochemical reduction of NB at BiF/CPE was studied in PBS with different concentrations of CTAB in the range of 0–5.0 × 10−5 mol L−1. The influence of CTAB concentration on the SWV response of NB is shown in Fig. 4. As can be observed in Fig. 4, the cathodic peak current of NB obviously increased with increasing the concentration of CTAB from 0 to 2.0 × 10−5 mol L−1, however, the peak current of NB remained almost constant when the concentration of CTAB was higher than 2.0 × 10−5 mol L−1. The reasons may be that cationic surfactant CTAB might weakly adsorb onto the BiF/CPE and increase the concentration of NB on the electrode surface, which would help NB approach to the electrode surface and facilitate its cathodic electrochemical detection. Further increase of CTAB concentration did not increase the amount of NB at the electrode surface due to surface saturation, and the peak current remained constant.34Fig. 4 also shows that the cathodic peak potential of NB substantially shifted positive with CTAB concentration up to 2.0 × 10−5 mol L−1 and slightly shifted for higher CTAB concentration. Thus, 2.0 × 10−5 mol L−1 of CTAB in the analytic solution was selected for the voltammetric determination of NB.
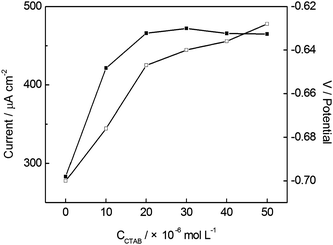 |
| Fig. 4 Effect of CTAB concentration on the SWV peak current (■) and peak potential (□) of 1.0 × 10−4 mol L−1 NB at BiF/CPE in 0.10 mol L−1 PBS (pH 5.5). Other conditions are the same as in Fig. 1. | |
3.5. Effect of the scan rate on the voltammetric response of NB
The voltammetric response of 1.0 × 10−4 mol L−1 NB on BiF/CPE at different scan rate over the range of 20–400 mV s−1 was tested, as shown in Fig. 5. The cathodic peak current (ipc) of NB varied linearly with the square root of scan rate (v1/2), and the regression equation is ipc(μA) = −19.81 + 4.84v1/2(mV s−1) with correlation coefficient of 0.9983, following the Ramdles-Sevick Equation: ipc = 2.687 × 105AD1/2n3/2v1/2C, where A is the electrode area, D is the diffusion coefficient of molecule in solution, v is the scan rate, C is the analyte concentration in the bulk solution.35 In addition, a negative potential shift with increasing scan rate was also observed (Fig. 5). These results indicate that the reduction of NB is a diffusion-controlled irreversible electrochemical process, which may include two steps: the first one involving the diffusion of NB from the bulk, the second one involving the diffusion inside the layer formed by the surfactant.
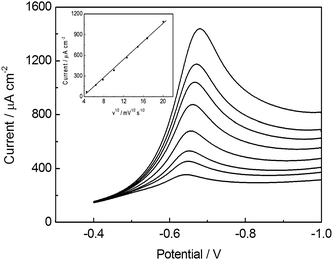 |
| Fig. 5 Linear sweep voltammograms of 1.0 × 10−4 mol L−1 NB obtained at BiF/CPE in 0.10 mol L−1 PBS (pH 5.5) containing 2.0 × 10−5 mol L−1 CTAB at different scan rates: 20, 40, 60, 100, 160, 220, 280, 400 (mV s−1). Inset is the calibrated plot of peak current vs. v1/2. Other conditions are the same as in Fig. 1. | |
The effect of accumulation time on the peak current was also studied. The result shows that the peak current hardly changed with increasing the accumulation time, indicating no further adsorptive characteristics at BiF/CPE.
3.6. Interferences
Some anions and metal ions were investigated to evaluate the possible interference with the determination of NB. The results suggest that 500-fold NO3−, SO42−, CO32− and PO43− (concentration ratios) had no significant influence on the signal of 1.0 × 10−4 mol L−1 NB. It was also found that 200-fold Mg2+, 100-fold Mn2+, 20-fold Zn2+, 5-fold Pb2+ and Cd2+ produced negligible contributions to the signal of NB. In addition, some organic compounds were also tested. The results indicate that no significant interference was observed in the presence of 100-fold toluene and aminophenol.
3.7. Calibration curve, limit of detection and reproducibility
A series of square wave voltammograms for increasing the concentration of NB obtained at an ex-situ plated BiF/CPE are illustrated in Fig. 6. The calibration curves exhibits excellent linearity with the concentration in the range of 1.0 × 10−6–1.0 × 10−4 mol L−1 for NB with correlation coefficient of 0.9980. The sensitivity was 2.89 × 105 μA L mol−1. The limit of detection was 8.3 × 10−7 mol L−1 based on threefold standard deviation of a blank. The response characteristics of NB obtained by the proposed method are compared with those obtained by other electrochemical methods, and the results are summarized in Table 1. In comparison with some other voltammetric methods of NB detection, the proposed electrochemical method is sensitive for the determination of NB.
Table 1 Comparison of the performance of the modified electrode proposed in this work with other modified electrodes reported in literatures for the detection of NB
Modified electrode |
Linear range/mol L−1 |
LOD/mol L−1 |
Correlation coefficient |
Ref. |
OMC/DDAB composite modified electrode |
2.0 × 10−5∼2.9 × 10−3 |
1.0 × 10−5 |
0.9990 |
36
|
hanging mercury drop electrode |
1.47 × 10−5∼1.0 × 10−3 |
5.0 × 10−6 |
0.9995 |
37
|
10% C60 modified carbon paste electrode |
5.0 × 10−5∼6.0 × 10−3 |
3.0 × 10−5 |
0.9998 |
38
|
BiF/CPE |
1.0 × 10−6∼1.0 × 10−4 |
8.3 × 10−7 |
0.9980 |
This work |
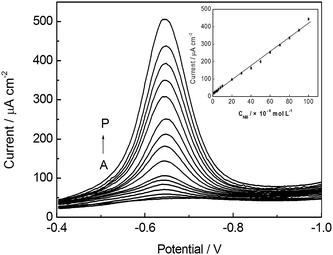 |
| Fig. 6 Square wave voltammograms obtained at an ex-situ plated BiF/CPE in 0.10 mol L−1 PBS (pH 5.5) containing 2.0 × 10−5 mol L−1 CTAB and different concentration of NB. From (A) to (P): blank, 1.0, 2.0, 4.0, 6.0; 8.0; 10; 20, 30, 40, 50, 60, 70, 80, 90, 100 (× 10−6 mol L−1). Other conditions are the same as in Fig. 1. | |
The reproducibility of BiF/CPE was examined by detecting the cathodic peak current of 1.0 × 10−4 mol L−1 NB in 0.10 mol L−1 PBS (pH 5.5) containing 2.0 × 10−5 mol L−1 CTAB. When fifteen replicate measurements were performed at the same BiF/CPE and CPE, the relative standard deviation (RSD) of peak currents were 2.67% and 4.60%, respectively. In addition, when six consecutive measurements were carried out each time at a fresh BiF/CPE, the RSD was 4.1%. No mechanical or any electrochemical pretreatment of the substrate CPE surface prior to re-plating a new bismuth film was applied. The results indicate that BiF/CPE has an acceptable reproducibility for monitoring NB.
In order to further demonstrate the practicality of the proposed method, recovery tests were carried out by adding a certain amount of NB into tap water samples which were collected from our laboratory. The concentration of NB was determined using the standard addition method. Results are listed in Table 2. The recoveries suggest that the method is effective for the determination of NB.
Table 2 Results of determination of NB in tap water sample
Sample |
Found/mol L−1 |
Standard added/mol L−1 |
Found after added/mol L−1a |
Recovery (%) |
mean of three determinations.
|
Tap water 1 |
0 |
1.0 × 10−5 |
0.971 × 10−5 |
97.1 |
Tap water 2 |
0 |
1.0 × 10−5 |
1.026 × 10−5 |
102.6 |
4. Conclusions
In this paper, voltammetric determination of NB at BiF/CPE in the presence of surfactant CTAB was described. The sufficiently wide negative potential window made the bismuth film electrode a potentially suitable electrode for application in the field of cathodic electrochemical detection of NB. The bismuth film electrode revealed an advantageous electrochemical performance which compared to that of the bare CPE. The voltammetric response of BiF/CPE to NB was apparently improved by CTAB. The proposed method for detecting NB is simple, sensitive, and electrochemically renewable surface together with high stability and reproducibility.
Acknowledgements
This research is supported by the National Natural Science Foundation of China (No. 20805044, 10804067), Leading Academic Discipline Project of Shanghai Municipal Education Commission (J50102) and the State Key Laboratory of Electroanalytical Chemistry, Changchun Institute of Applied Chemistry, Chinese Academy of Sciences, China (No. 2009005).
References
- Y. Nishikawa and T. Okumura, Anal. Chim. Acta, 1995, 312, 45–55 CrossRef CAS.
- Y. P. Li, H. B. Cao, C. M. Liu and Y. Zhang, J. Hazard. Mater., 2007, 148, 158–163 CrossRef CAS.
- P. S. Majumder and S. K. Gupta, Water Res., 2003, 37, 4331–4336 CrossRef CAS.
- S. P. Wang and H. J. Chen, J. Chromatogr., A, 2002, 979, 439–446 CrossRef CAS.
- S. F. Patil and S. T. Lonkar, J. Chromatogr., A, 1994, 688, 189–199 CrossRef CAS.
- Y. Chen, K. Liang, H. Xun and S. Y. Wang, Capital Normal University Transaction (Nature Science), 1998, 19 Search PubMed.
-
J. Wang, Analytical electrochemistry, 3rd ed., Wiley-VCH, New York, 2006 Search PubMed.
- S. Zhu, W. Niu, H. Li, S. Han and G. Xu, Talanta, 2009, 79, 1441–1445 CrossRef CAS.
- F. Li, J. Song, D. Gao, Q. Zhang, D. Han and L. Niu, Talanta, 2009, 79, 845–850 CrossRef.
- A. Economuou and P. R. Fielden, Analyst, 2003, 128, 205–212 RSC.
- J. Wang, J. Lu, S. B. Hocevar and P. A. M. Farias, Anal. Chem., 2000, 72, 3218–3222 CrossRef CAS.
- J. Wang, J. Lu, Ü. A. Kirgöz, S. B. Hocevar and B. Ogorevc, Anal. Chim. Acta, 2001, 434, 29–34 CrossRef CAS.
- A. Economou, TrAC, Trends Anal. Chem., 2005, 24, 334–340 CrossRef CAS.
- A. Krolicka and A. Bobrowski, Electrochem. Commun., 2004, 6, 99–104 CrossRef CAS.
- L. Hu, H. Li, S. Zhu, L. Fan, L. Shi, X. Liu and G. Xu, Chem. Commun., 2007, 4146–4148 RSC.
- B. Claux and O. Vittori, Electroanalysis, 2007, 19, 2243–2246 CrossRef CAS.
- V. Guzsvany, M. Kadar, F. Gaal, L. Bjelica and K. Toth, Electroanalysis, 2006, 18, 1363–1371 CrossRef CAS.
- D. Shan, J. Zhang, H. G. Xue, Y. C. Zhang, S. Cosnier and S. N. Ding, Biosens. Bioelectron., 2009, 24, 3671–3676 CrossRef CAS.
- G. Xu, H. L. Pang, B. Xu, S. Dong and K. Y. Wong, Analyst, 2005, 130, 541–544 RSC.
- N. F. Atta, S. A. Darwish, S. E. Khalil and A. Galal, Talanta, 2007, 72, 1438–1445 CrossRef CAS.
- C. Li, Colloids Surf., B, 2007, 55, 77–83 CrossRef CAS.
- Q. He, X. P. Dang, C. G. Hu and S. S. Hu, Colloids Surf., B, 2004, 35, 93–98 CrossRef CAS.
- G. J. Yang, X. L. Qu, M. Shen, C. Y. Wang, Q. S. Qu and X. Y. Hu, Sens. Actuators, B, 2007, 128, 258–265 CrossRef.
- J. B. Raoof, M. A. Karimi, S. R. Hosseini and S. Mangelizadeh, J. Electroanal. Chem., 2010, 63, 833–838.
- Q. He, X. Dang, C. Hu and S. Hu, Colloids Surf., B, 2004, 35, 93–98 CrossRef CAS.
- P. Xie, X. Chen, F. Wang, C. Hu and S. Hu, Colloids Surf., B, 2006, 48, 17–23 CrossRef CAS.
- V. Arancibia, E. Nagles and S. Cornejo, Talanta, 2009, 80, 184–188 CrossRef CAS.
- T. A.d. Araújo, J. C. Cardoso, A. M. J. Barbosa and V. S. Ferreira, Colloids Surf., B, 2009, 73, 408–414 CrossRef.
- E. A. Hutton, B. Ogorevc and M. R. Smyth, Electroanalysis, 2004, 16, 1616–1621 CrossRef CAS.
- B. Nigovic, B. Simunic and S. Hocevar, Electrochim. Acta, 2009, 54, 5678–5683 CrossRef CAS.
- A. P. dos Reis, C. R. T. Tarley, N. Maniasso and L. T. Kubota, Talanta, 2005, 67, 829–835 CrossRef CAS.
- E. A. Hutton, B. Ogorevc, S. B. Hocevar, F. Weldon, M. R. Smyth and J. Wang, Electrochem. Commun., 2001, 3, 707–711 CrossRef CAS.
- H. K. Zhang, S. X. Liang and S. J. Liu, Anal. Bioanal. Chem., 2007, 387, 1511–1516 CrossRef CAS.
- D. Dua, X. Ye, J. Zhang and D. Liu, Electrochim. Acta, 2008, 53, 4478–4484 CrossRef.
-
A. J. Bard, L. R. Faulkner, Electrochemical Methods, 2nd ed, Wiley, New York, 2001 Search PubMed.
- B. Qi, F. Lin, J. Bai, L. Liu and L. Guo, Mater. Lett., 2008, 62, 3670–3672 CrossRef CAS.
- S. X. Liang, H. K. Zhang and D. Lu, Environ. Monit. Assess., 2007, 129, 331–337 CrossRef CAS.
- H. Qian, J. Ye and L. Jin, Anal. Lett., 1997, 30, 367–381 CAS.
|
This journal is © The Royal Society of Chemistry 2010 |