DOI:
10.1039/B9AY00324J
(Paper)
Anal. Methods, 2010,
2, 707-713
Received
23rd December 2009
, Accepted 15th March 2010
First published on
15th April 2010
Abstract
Benzophenone (BP)-type UV (ultraviolet) filters, especially 2-hydroxy-4-methoxybenzophenone (2OH–4MeO–BP), are widely used in the U.S., to protect the skin and hair from UV irradiation. Despite human exposures to UV filters through the dermal application of products containing sunscreen agents, few studies have examined the occurrence of UV filters in humans. Thus far, few sensitive methods are available for the determination of 2OH–4MeO–BP in human urine. Furthermore, methods for the determination of other BP derivatives, including 2,4-dihydroxybenzophenone (2,4OH–BP), which is formed from 2OH–4MeO–BP via metabolic activities of the cytochrome P450 enzymes, have not been available. In this study, we have developed a method for the analysis of five BP derivatives: 2OH–4MeO–BP, 2,4OH–BP, 2,2′-dihydroxy-4-methoxybenzophenone (2,2′OH–4MeO–BP), 2,2′,4,4′-tetrahydroxybenzophenone (2,2′,4,4′OH–BP), and 4-hydroxybenzophenone (4OH–BP) in human urine, using liquid–liquid extraction and liquid chromatograph (LC)-tandem mass spectrometer (MS/MS) analysis. The instrumental calibration range for each of the BP derivatives ranged from 0.05 to 100 ng ml−1, and showed excellent linearity (r > 0.99). The respective limits of detection (LODs) and limits of quantification (LOQs) were determined to be 0.082 and 0.28 ng ml−1 for both 2,4OH–BP and 4OH–BP; 0.13 and 0.44 ng ml−1 for 2,2′OH–4MeO–BP; and 0.28 and 0.90 ng ml−1 for both 2OH–4MeO–BP and 2,2′,4,4′OH–BP. Recoveries of BP derivatives through the entire analytical procedure were between 85.2 and 99.6%. The coefficients of variation (CVs) of five replicate analyses within 1 day, and across 5 days, were respectively 1.4 and 3.7% for 2,4OH–BP; 1.7 and 3.0% for 2OH–4MeO–BP; and 2.8 and 4.5% for 4OH–BP. When BP derivatives were determined in urine samples from 23 U.S. (Albany, New York) and 22 Japanese (Matsuyama, Ehime) volunteers, higher concentrations of 2,4OH–BP, 2OH–4MeO–BP, and 4OH–BP were found in samples collected from females in the Albany cohort, probably reflecting great usage by U.S. females. The urine sample from a known sunscreen user contained very high concentrations of 2,4OH–BP and 2OH–4MeO–BP. 2,2′,4,4′OH–BP and 2,2′OH–4MeO–BP were not detected in any of the urine samples analyzed. Our results indicate considerable exposure to highly estrogenic 2,4OH–BP and 2OH–4MeO–BP by females in the U.S., and suggest the need for further studies on potential health effects.
1. Introduction
For the protection of skin and hair from ultraviolet (UV) irradiation, organic chemicals that can absorb the UV radiation and attenuate the negative effects have been used in a variety of personal care products; those chemicals are commonly referred to as UV filters. UV filters enter the aquatic environment either directly, via wash-off from skin and clothes during water recreational activities, or indirectly, via discharges of sewage and swimming pool waters. UV filters have been detected in environmental matrices such as water, soil, and sediment samples.1 A few investigations on experimental animals have indicated that some organic UV filters possess significant estrogenic effects.2
In the U.S., 2-hydroxy-4-methoxybenzophenone (2OH–4MeO–BP or BP-3), a benzophenone (BP) derivative, is widely used in a variety of cosmetic products as a UV filter, and it is also used as a UV stabilizer in plastic surface coatings, to prevent photodegradation of polymers or foods.3 Human exposures to 2OH–4MeO–BP can be through the dermal and oral routes. When sunscreen lotion containing 2OH–4MeO–BP was applied to human skin, significant amounts of this chemical penetrated the epidermal barrier, and 1–2% of the amount applied on the skin was absorbed in the bloodstream within 10 h.4 In addition, 2OH–4MeO–BP has been shown to penetrate human skin more readily than do other sunscreen agents.5,6 Further, 2OH–4MeO–BP has shown weak estrogen-like activity in vitro and in vivo2,7–10 and antiandrogenic activity in vitro;10,11 thus it is a potential endocrine-disrupting compound.
It has been reported that, in rats and piglets, 2OH–4MeO–BP is metabolized to 2,4-dihydroxybenzophenone (2,4OH–BP or BP-1) and 2,2′-dihydroxy-4-methoxybenzophenone (2,2′OH–4MeO–BP or BP-8), compounds that are also used in cosmetic products as sunscreen agents12–15 (see Fig. 1). Interestingly, there is evidence that 2,4OH–BP possesses an estrogenic activity higher than that of 2OH–4MeO–BP,7–9,13,16 and it can also display antiandrogenic activity8in vitro. Additive estrogenic effects of 2,4OH–BP and 2OH–4MeO–BP, on pS2-gene transcription in MCF-7 cells, have been reported.17 In addition, other BP derivatives, such as 2,2′,4,4′-tetrahydroxybenzophenone (2,2′,4,4′OH–BP or BP-2) and 4-hydroxybenzophenone (4OH–BP), have been shown to have estrogenic activities higher than that of 2OH–4MeO–BP.7,9 Nevertheless, information regarding occurrence of BP derivatives in humans is scarce, except for 2OH–4MeO–BP.
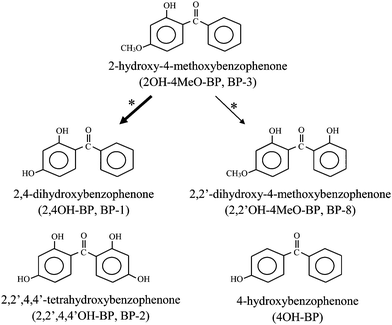 |
| Fig. 1 Chemical structures of the benzophenone-type UV filters analyzed in this study. *Metabolic pathway of BP-3 was suggested from some experimental studies (The thickness of arrows indicates that BP-1 is mainly formed from BP-3). | |
2OH–4MeO–BP has been recently analyzed in water,18–20 fish,19 and human urine,21–24 using liquid chromatograph (LC) - UV detection, gas chromatograph (GC) - mass spectrometer (MS) and LC - tandem mass spectrometer (MS/MS). Jeon et al.25 detected 2OH–4MeO–BP, 2,4OH–BP, and 2,2′OH–4MeO–BP in water, soil, and sediment samples using GC–MS. Felix et al.26 measured 2OH–4MeO–BP, 2,4OH–BP, and 2,2′OH–4MeO–BP in urine from a person using sunscreen product that contained 2OH–4MeO–BP; 2OH–4MeO–BP was detected in the urine, while 2,4OH–BP was not resolved adequately, and no peak was found for 2,2′OH–4MeO–BP. Thus, more selective and sensitive analytical methods are needed for the determination of BP derivatives in human specimens.1
In this study, we have developed a method for the determination of five BP derivatives, namely, 2OH–4MeO–BP; 2,4OH–BP; 2,2′OH–4MeO–BP; 2,2′,4,4′OH–BP; and 4OH–BP, in human urine, using LC-MS/MS. The chemical structures of the five BP derivatives are shown in Fig. 1. We believe that urine is a useful matrix for the biomonitoring of human exposure to BP derivatives, for the following reasons: polar metabolites are readily excreted in urine, concentrations of the polar compounds are generally higher in urine than in other biological matrices, and collection of urine is relatively easier than is the case for other matrices.
2. Experimental
2.1. Chemicals and reagents
2OH–4MeO–BP (purity: 98%), 2,4OH–BP (purity: 99%), 2,2′OH–4MeO–BP (purity: 98%), 2,2′,4,4′OH–BP (purity: 97%), 4OH–BP (purity: 98%), deuterated bisphenol-A (d16-BPA), 4-methylumbelliferone, 4-methylumbelliferyl β-glucuronide, and β-glucuronidase/sulfatase (Type HP-2, from Helix pomatia, ≥100,000 units/ml glucuronidase and ≤7,500 units/ml sulfatase) were purchased from Sigma-Aldrich (St. Louis, MO). Analytical grade methanol (MeOH), methyl tert-butyl ether (MTBE), and ammonium acetate were obtained from Mallinckrodt Baker Inc. (Phillipsburg, NJ), and ethyl acetate was from Honeywell International Inc. (Morristown, NJ). Deionized (DI) water was generated with a NANOpure Diamond ultrapure water system (Barnstead International, Dubuque, IA), and had a resistance of 18.2 MΩ cm.
2.2. Standard solution
Stock solutions of BP derivatives, d16-BPA, 4-methylumbelliferone, and 4-methylumbelliferyl β-glucuronide were prepared at 1 mg ml−1, using MeOH, and were then stored at −20 °C in a dark room. The calibration standards, ranging in concentration from 0.05 to 100 ng ml−1, were prepared from the stock solution through dilution with MeOH, whenever a new batch of samples was injected into the LC-MS/MS (i.e., every 5 days). Solution at 10 ng ml−1 and 500 ng ml−1 of d16-BPA, 50 ng ml−1 (283.82 nM) of 4-methylumbelliferone, and 10 μg ml−1 (28.385 μM) of 4-methylumbelliferyl β-glucuronide were made from each of the stock solutions, to check recoveries and deconjugation efficiencies. As described later, 100 ng (10 μl of 10 ppm solution) of 4-methylumbelliferyl β-glucuronide was spiked into each urine sample, and the final volume prior to LC-MS/MS analysis was set at 1 ml. The molarity of 4-methylumbelliferone deconjugated from 4-methylumbelliferyl β-glucuronide was calculated as 283.85 nM.
2.3. Sample collection
In this study, we analyzed 52 urine samples (50–200 ml) that were stored at −20 °C at the New York State Department of Health (NYSDOH); the samples had been collected during February-March 2005 from two cohorts: scientists, students, and office workers at Wadsworth Center of NYSDOH, located in Albany, New York, USA (male: n = 14 [24–63 years old], female: n = 6 [23–45 years old]); and a similar group of subjects at Center for Marine Environmental Studies (CMES), Ehime University, located in Matsuyama, Japan (male: n = 19 [22–55 years old], female: n = 13 [21–36 years old]). Information on use of sunscreen was not available for these 52 subjects. We also obtained urine samples (50 ml) from three NYSDOH scientists in September 2009: a sunscreen user (male; 47 years old) and two non-sunscreen users (male; 52 years old, female; 48 years old). Each sample was collected in a 50-ml polypropylene (PP) conical tube and stored at −20 °C until analysis. The samples were devoid of any personal identifiers. Institutional Review Board approvals were obtained from NYSDOH for the analysis of urine samples.
Urine samples were removed from the −20 °C freezer and thawed overnight at 4 °C. A 500-μl or 100-μl (for a sunscreen user) aliquot of urine was transferred into a 15-ml PP tube, using a tip ejector variable volume micropipetter. Then, 10 ng (20 μl of 500 ppb solution) of d16-BPA and 100 ng (10 μl of 10 ppm solution) of 4-methylumbelliferyl β-glucuronide were spiked as internal and deconjugation standards, respectively. After gentle mixing, 300 μl of β-glucuronidase/sulfatase buffer, which contains 2 μl enzyme and 1 ml of 1.0 M ammonium acetate, was added. After vortex mixing, the sample was incubated overnight at 37 °C. Then, 3 ml of 50% MTBE/ethyl acetate was added, shaken for 30 min using a reciprocating shaker, and centrifuged at 3,000 g for 2 min. The organic phase, containing BP derivatives, was transferred into a new 15-ml PP tube, and the aqueous phase was extracted one more time with 3 ml of 50% MTBE/ethyl acetate. The organic phases were combined, evaporated to near-dryness using N2, and redissolved with 1 ml of MeOH. After sonication for 10 s and centrifugation at 3,000 g for 2 min, the solution was transferred to a 1.5-ml amber vial for LC-MS/MS analysis.
An API 2000 electrospray triple quadrupole mass spectrometer (ESI-MS/MS; Applied Biosystems, Foster City, CA) equipped with an Agilent 1100 Series HPLC system (Agilent Technologies Inc., Santa Clara, CA) was used for the measurement of BP derivatives. The negative ion multiple reaction monitoring (MRM) mode was used, and the MRM transitions monitored were 227>211 (confirmation ion: 183) for 2OH–4MeO–BP, 213>91 (65) for 2,4OH–BP, 243>93 (108) for 2,2′OH–4MeO–BP, 245>91 (109) for 2,2′,4,4′OH–BP, and 197>92 (120) for 4OH–BP. Nitrogen was used as both curtain and collision gas. MS/MS parameters were optimized for each BP derivative, by infusion of 1 μg ml−1-standard solution. The optimized MS/MS parameters for BP derivatives are summarized in Table 1. The MRM transitions monitored for d16-BPA and 4-methylumbelliferone were 241>142 and 175>119, respectively.
|
2OH-4MeO-BP |
24OH-BP |
2,2′OH-4MeO-BP |
2,2′,4,4′OH-BP |
4OH–BP |
Curtain Gas (CUR) |
10 |
10 |
20 |
15 |
10 |
Collision Gas (CAD) |
6 |
7 |
7 |
7 |
7 |
IonSpray Voltage (IS) |
−4500 |
−4000 |
−4000 |
−4000 |
−4000 |
Temp (TEM) |
400 |
400 |
400 |
400 |
400 |
Ion Source Gas 1 (GS1) |
50 |
50 |
50 |
50 |
50 |
Ion Source Gas 2 (GS2) |
60 |
60 |
60 |
60 |
60 |
Declustering Potential (DP) |
−25 |
−30 |
−25 |
−25 |
−30 |
Focusing Potential (FP) |
−400 |
−400 |
−300 |
−400 |
−400 |
Entrance Potential (EP) |
−10 |
−10 |
−9 |
−9 |
−10 |
Collision Energy (CE) |
−30 |
−40 |
−40 |
−40 |
−40 |
Collision Cell Exit Potential (CXP) |
−7 |
−5 |
−5 |
−5 |
−5 |
Ten microlitres of urine extract were injected onto a Thermo BETASIL C18 (100 mm length × 2.1 mm internal diameter, 5 μm particle diameter) chromatographic column serially connected with a guard column (20 × 2.1 mm, 5 μm; Thermo Electron Co., Bellefonte, PA), at a flow rate of 300 μl min−1. The mobile phase was MeOH (solvent A) and deionized water (solvent B); the gradient parameters are shown in Table 2.
Table 2 HPLC gradient parameters optimized for analysis of benzophenone derivatives
2.7. Data analysis
The analytes were quantified from an external calibration curve prepared at concentrations ranging from 0.05 to 100 ng ml−1, when the retention times of BP derivatives in urine matched those of the standards within ±0.05 min. If concentrations of BP derivatives in urine were above 100 ng ml−1, the sample was re-analyzed using a smaller volume (100 μl). In this study, the urine sample from one sunscreen user had to be re-analyzed for 2OH–4MeO–BP and 2,4OH-BP due to high concentration (i.e., further dilutions were needed). Data processing was performed with the Analyst 1.4.1 software package. Statistical analyses were conducted with Statistica V. 06J (StatSoft Inc., Tulsa, OK). The statistical significance of differences in concentrations of BP derivatives, between the 20 U.S. male and female samples collected in 2005, was evaluated by Mann-Whitney U test. Samples with concentrations less than the limit of detection (LOD) were treated as the LOD value, for the statistical analysis. A p value <0.05 was considered significant.
3. Results and discussion
3.1. Instrumental calibration and limit of detection
Calibration standards injected at eight different concentrations, ranging from 0.05 to 100 ng ml−1, for each of the BP derivatives showed excellent linearity (r > 0.99). When 10 μl of 0.05 ng ml−1 (i.e., 0.5 pg of 2,4OH–BP and 4OH–BP) or 10 μl of 0.1 ng ml−1 (i.e., 1.0 pg of 2OH–4MeO–BP, 2,2′OH–4MeO–BP, and 2,2′,4,4′OH–BP) standard was injected, the signal to noise (S/N) ratio was 3.0 for 2,4OH–BP and 2,2′,4,4′OH–BP; 3.3 for 4OH–BP and 2OH–4MeO–BP; and 5.0 for 2,2′OH–4MeO–BP. Thus, the instrumental detection limits were in the range of 0.5–1.0 pg. The LOD and LOQ values for the analytical method were determined based on the standard deviations of six replicate analyses, using the lowest calibration standard, i.e., 0.05 ng ml−1 for 2,4OH–BP and 4OH–BP, and 0.1 ng ml−1 for 2OH–4MeO–BP, 2,2′OH–4MeO–BP, and 2,2′,4,4′OH–BP. The LOD and LOQ values were calculated as 3S and 10S, respectively, where S is the standard deviation. The calculated LOD and LOQ values were, respectively, 0.041 and 0.14 ng ml−1 for both 2,4OH–BP and 4OH–BP; 0.067 and 0.22 ng ml−1 for 2,2′OH–4MeO–BP; and 0.14 and 0.45 ng ml−1 for both 2OH–4MeO–BP and 2,2′,4,4′OH–BP. Because of the 2–fold dilution of the sample in the analytical procedure, the actual LOD and LOQ values for urine samples analyzed in this study were 0.082 and 0.28 ng ml−1 for both 2,4OH–BP and 4OH–BP; 0.13 and 0.44 ng ml−1 for 2,2′OH–4MeO–BP; and 0.28 and 0.90 ng ml−1 for both 2OH–4MeO–BP and 2,2′,4,4′OH–BP.
3.2. Analytical accuracy and precision
A recovery test was conducted, through spiking of two concentrations (1.0 and 10 ppb) of each of five BP derivatives into a urine sample that had been found not to contain any of the target compounds. The spiked urine sample was passed through the entire analytical procedure. The recoveries of five BP derivatives in urine were between 85.2 and 99.6%, and the coefficients of variation (CVs) of triplicate analyses were between 0.53 and 5.9% (Table 3). In addition, variations in the responses of BP derivatives within a day and between 5 days were checked by replicate analyses of a U.S. urine sample collected in 2005 that had been found to contain 2,4OH–BP, 2OH–4MeO–BP, and 4OH–BP. Respective CVs of five replicates within a day and between 5 days were 1.4 and 3.7% for 2,4OH–BP, 1.7 and 3.0% for 2OH–4MeO–BP, and 2.8 and 4.5% for 4OH–BP (Table 4). These results suggest that the analytical method developed for the analysis of five BP derivatives in urine provides adequate accuracy and precision.
Table 3 Recoveries (n = 3) of benzophenone (BP) derivatives through the entire analytical procedure, after spiking of either of two concentrations (1.0 and 10 ppb) of BP derivatives into a urine sample
|
Added amount/ng ml−1 |
Mean value |
(SD) |
Recovery (%) |
CV (%) |
2,2′,4,4′OH-BP |
0 |
<LOD |
|
|
|
1 |
0.946 |
(0.02) |
94.6 |
2.1 |
10 |
9.16 |
(0.1) |
91.6 |
1.1 |
2,2′OH-4MeO-BP |
0 |
<LOD |
|
|
|
1 |
0.852 |
(0.05) |
85.2 |
5.9 |
10 |
8.56 |
(0.24) |
85.6 |
2.8 |
2,4OH-BP |
0 |
<LOD |
|
|
|
1 |
0.991 |
(0.01) |
99.1 |
1.0 |
10 |
9.84 |
(0.25) |
98.4 |
2.5 |
2OH-4MeO-BP |
0 |
<LOD |
|
|
|
1 |
0.873 |
(0.02) |
87.3 |
2.3 |
10 |
8.78 |
(0.1) |
87.8 |
1.1 |
4OH-BP |
0 |
<LOD |
|
|
|
1 |
0.996 |
(0.01) |
99.6 |
1.0 |
10 |
9.52 |
(0.05) |
95.2 |
0.53 |
Table 4 Within-day and between-day variations in determinations of 2,4OH–BP, 2OH–4MeO–BP, and 4OH–BP, by replicate analyses of a urine sample
|
Within-day precision (n = 5) |
Between-day precision (1 run per day for 5 days) |
2,4OH-BP |
2OH-4MeO-BP |
4OH-BP |
2,4OH-BP |
2OH-4MeO-BP |
4OH–BP |
1 |
4.9 |
7.1 |
0.82 |
4.6 |
7.3 |
0.76 |
2 |
4.9 |
7.2 |
0.79 |
4.9 |
6.8 |
0.77 |
3 |
4.9 |
7.0 |
0.78 |
4.5 |
6.8 |
0.76 |
4 |
4.8 |
7.0 |
0.82 |
4.6 |
7.0 |
0.84 |
5 |
4.9 |
7.2 |
0.78 |
4.4 |
6.8 |
0.76 |
Average |
4.9 |
7.1 |
0.80 |
4.6 |
6.9 |
0.78 |
SD |
0.067 |
0.12 |
0.023 |
0.17 |
0.21 |
0.035 |
CV (%) |
1.4 |
1.7 |
2.8 |
3.7 |
3.0 |
4.5 |
When BP derivatives were analyzed in 55 urine samples from the U.S. and Japan, d16-BPA was used as an internal standard, as reported in earlier studies on 2OH–4MeO–BP in urine; 13C12-BPA has been used in those studies.21–23 Recoveries of d16-BPA spiked into urine samples, in our study, were between 78.5 and 96.0%. Deconjugation efficiencies, as estimated from the concentrations of 4-methylumbelliferone, were nearly 100%. No BP derivatives were detected in procedural blanks, which consisted of solvents and reagents passed through the entire analytical procedure.
3.3. Benzophenone-type UV filters in human urine
Typical chromatograms of BP derivatives detected in human urine and standard solutions are shown in Fig. 2. 2,2′,4,4′OH–BP and 2,2′OH–4MeO–BP were not detected in any of the urine samples analyzed. Detection frequencies of 2,4OH–BP, 2OH–4MeO–BP, and 4OH–BP in urine samples collected in February-March 2005 are shown in Fig. 3.
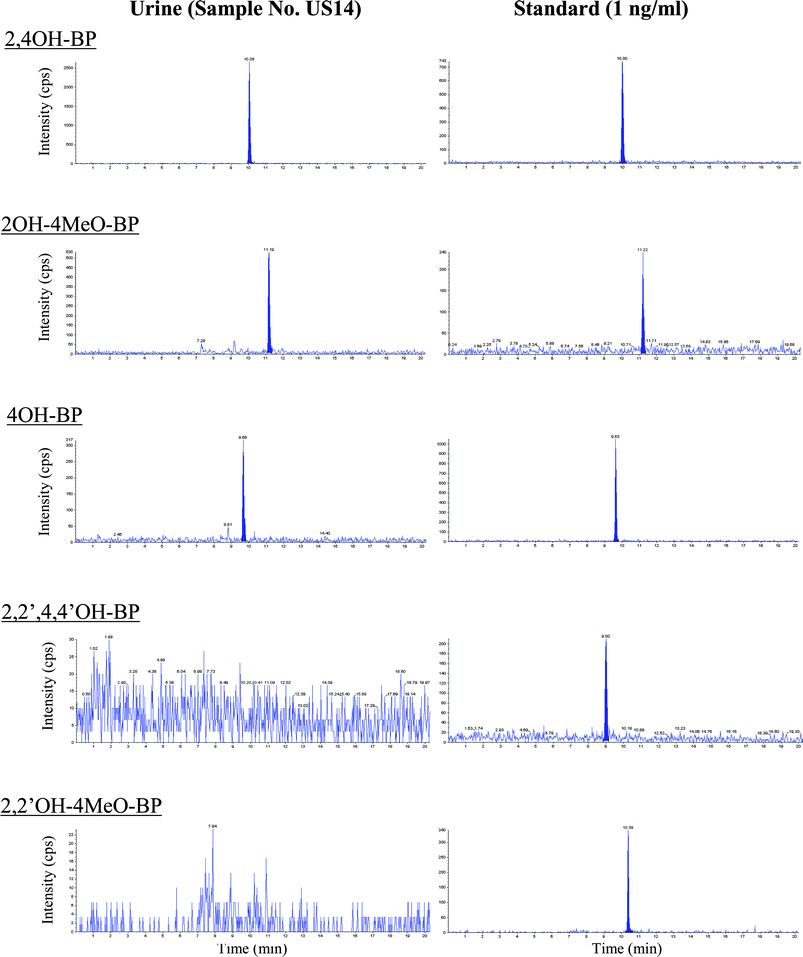 |
| Fig. 2 Chromatograms of BP derivatives detected in human urine and in a standard solution. | |
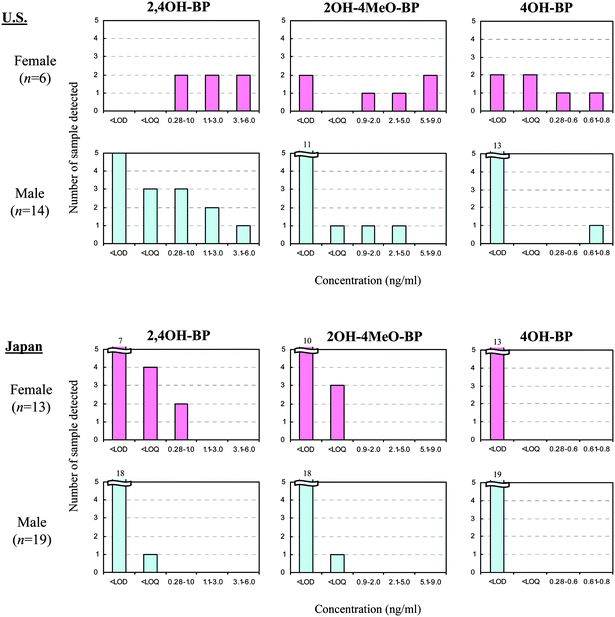 |
| Fig. 3 Detection frequencies of BP derivatives in urine samples collected from subjects in Albany, New York and in Matsuyama, Japan, during February-March 2005. | |
2,4OH–BP, 2OH–4MeO–BP, and 4OH–BP were respectively detected in 100%, 66.7%, and 33.3% of the urine samples from females, and in 42.6%, 21.4%, and 7.1% of the urine samples from males, in Albany, New York (Fig. 3). Concentrations of BP derivatives in urine samples from females were significantly higher than those in males (p<0.05). This suggests higher exposures in females than in males and can be attributed to higher usage of both sunscreen products and also other cosmetic products containing UV filters by females. Among 32 Japanese urine samples analyzed, only 2,4OH–BP was found in only two samples from females (15.4%); no samples from males had any detectable concentrations (Fig. 3). These results indicate that the U.S. females are exposed to relatively high concentrations of BP derivatives. Although published data for 2,4OH–BP and 4OH–BP are not available, geometric mean concentration of 2OH–4MeO–BP in 2517 urine samples collected from the U.S. general population (≥ 6 years of age), as part of the 2003–2004 National Health and Nutrition Examination Survey, was 22.9 ng ml−1 (range: 0.4–21700 ng ml−1).23 Concentrations of this BP derivative in females were significantly higher than in males in that study, indicating probable differences in the use of personal care products containing 2OH–4MeO–BP.23 Concentrations of 2OH–4MeO–BP in all of the urine samples from Albany that were analyzed in the present study were lower than the U.S. mean value of 22.9 ng ml−1 reported earlier.23 This difference could be ascribable in part to seasonal variations in personal exposure to BP derivatives. Wolff et al.27 measured concentrations of 2OH–4MeO–BP in urine collected from 90 girls (6.4–9.2 years old) in New York City (NY), Cincinnati (OH), and northern California. 2OH–4MeO–BP concentrations ranged from <0.2 to 26700 ng ml−1 and were significantly higher in samples collected in summer than in other seasons.27 We analyzed BP derivatives in urine collected from a male sunscreen user and two non-sunscreen users, in September 2009, and found notably high concentrations of 2OH–4MeO–BP and 2,4OH–BP in urine from the sunscreen user (Table 5), indicating that sunscreen products are one of the major exposure sources of BP derivatives in humans.
Table 5 Concentrations (ng ml−1) of BP derivatives in urine collected from a sunsceen user and two non-sunsceen users in September 2009 (Albany, NY)
|
2OH-4MeO-BP |
2,4OH-BP |
4OH-BP |
2,2′OH-4MeO-BP |
2,2′,4,4′OH–BP |
Numbers in parentheses denote age (in years).
|
Sunscreen user
|
Male (47)a |
330 |
250 |
1.53 |
<LOD |
<LOD |
Non user
|
Male (52)a |
<LOD |
0.39 |
<LOD |
<LOD |
<LOD |
Female (48)a |
<LOD |
0.33 |
0.37 |
<LOD |
<LOD |
Concentrations of 2,4OH–BP in urine were comparable to those of 2OH–4MeO–BP (Fig. 3 and Table 5). This observation can be attributed to the formation of 2,4OH–BP from 2OH–4MeO–BP via metabolic activities of cytochrome P450 enzymes, although humans can also be exposed to 2,4OH–BP directly, through the use of personal care products; the latter BP derivative is also present in some U.S. cosmetics, although the frequency of use of this compound is much lower than that of 2OH–4MeO–BP.28 In a skin absorption study,29 sunscreen lotion containing 2OH–4MeO–BP was applied to the skin of three female volunteers; the major metabolite in the urine was found to be 2,4OH–BP. It has also been reported that 2OH–4MeO–BP is metabolized principally to 2,4OH–BP, in rats12–14 and piglets.15 There is evidence that 2,4OH–BP possesses higher estrogenic activity than does 2OH–4MeO–BP,7–9,13,16 indicating that hydroxylated intermediates (such as 2,4OH–BP) can be potent xenoestrogens. Oral administration of 2OH–4MeO–BP to rats showed that the metabolite, 2,4OH–BP, decreased much more slowly in rat blood than did its parent compound, indicating that the metabolites can be retained in the body for a long time.14 4OH–BP detected in the present study could have been formed through oxidative metabolism of BP, but information on the use of 4OH–BP in personal care products, and evidence of its formation from 2OH–4MeO–BP and 2,4OH–BP are lacking. BP has been reported to be metabolized to 4OH–BP, following incubation with rat hepatocytes30 and in an in vivo study.14 The hydroxylated metabolite acted as a weak xenoestrogen on MCF-7 cells.30
4. Conclusions
We have developed a reliable LC-MS/MS method for the measurement of five BP derivatives, namely, 2OH–4MeO–BP, 2,4OH–BP, 2,2′OH–4MeO–BP, 2,2′,4,4′OH–BP, and 4OH–BP, in human urine. 2OH–4MeO–BP, 2,4OH–BP, and 4OH–BP were detected in the samples analyzed; concentrations of 2,4OH–BP were comparable to those of 2OH–4MeO–BP. Concentrations of the remaining two analytes, 2,2′OH–4MeO–BP and 2,2′,4,4′OH–BP, were below the limit of detection in all of the samples analyzed. Considering that elevated concentrations of 2OH–4MeO–BP and 2,4OH–BP found in the urine from a known sunscreen user sampled in late summer (September 2009) and considering that 2,4OH–BP possesses a higher estrogenic activity than does 2OH–4MeO–BP, comprehensive investigations on human exposures to not only 2OH–4MeO–BP but also its metabolite, 2,4OH–BP, are needed, especially for females and during the summer months.
Acknowledgements
This study was funded by a biomonitoring grant (1U38EH000464-01) from the Center for Disease Control and Prevention (CDC), Atlanta, GA and was supported by ‘Global COE Program’ and Grants-in-Aid for Scientific Research (S) (No. 20221003) from the Ministry of Education, Culture, Sports, Science and Technology, Japan (MEXT) and Japan Society for the Promotion of Science (JSPS). We thank all the donors in NYSDOH, Wadsworth Center, Albany, NY, USA and CMES, Ehime University, Matsuyama, Japan, for their provision of urine samples.
References
- D. L. Giokas, A. Salvador and A. Chisvert, Trends Anal. Chem., 2007, 26, 360–374 CrossRef CAS.
- M. Schlumpf, B. Cotton, M. Conscience, V. Haller, B. Steinmann and W. Lichtensteiger, Environ. Health Perspect., 2001, 109, 239–244 CAS.
- FDA Department of Health and Human Services, 21 CER Parts 310, 352, 700 and 740, RIN 0910-AAOI, Final Monograph, Federal Register, 1999, Vol. 64, No. 98, p. 27666.
- C. G. J. Hayden, M. S. Roberts and H. A. E. Benson, Lancet, 1997, 350, 863–864 CrossRef CAS.
- R. Jiang, M. S. Roberts, D. M. Collins and H. A. E. Benson, Br. J. Clin. Pharmacol., 1999, 48, 635–637 CrossRef CAS.
- N. R. Janjua, B. Mogensen, A.-M. Andersson, J. H. Petersen, M. Henriksen, N. E. Skakkebæk and H. C. Wulf, J. Invest. Dermatol., 2004, 123, 57–61 CrossRef CAS.
- K. Morohoshi, H. Yamamoto, R. Kamata, F. Shiraishi, T. Koda and M. Morita, Toxicol. in Vitro, 2005, 19, 457–469 CrossRef CAS.
- T. Suzuki, S. Kitamura, R. Khota, K. Sugihara, N. Fujimoto and S. Ohta, Toxicol. Appl. Pharmacol., 2005, 203, 9–17 CrossRef CAS.
- Y. Kawamura, Y. Ogawa, T. Nishimura, Y. Kikuchi, J. Nishikawa, T. Nishihara and K. Tanamoto, J. Health Sci., 2003, 49, 205–212 CrossRef CAS.
- R. H. M. M. Schreurs, E. Sonneveld, J. H. J. Jansen, W. Seinen and B. Van der Burg, Toxicol. Sci., 2004, 83, 264–272 CrossRef.
- R. Ma, B. Cotton, W. Lichtensteiger and M. Schlumpf, Toxicol. Sci., 2003, 74, 43–50 CrossRef CAS.
- C. S. Okereke, M. S. Abdel-Rhaman and M. A. Friedman, Toxicol. Lett., 1994, 73, 113–122 CrossRef CAS.
- Y. Nakagawa and T. Suzuki, Chem.-Biol. Interact., 2002, 139, 115–128 CrossRef CAS.
- H. Jeon, S. N. Sarma, Y. Kim and J. Ryu, Toxicology, 2008, 248, 89–95 CrossRef CAS.
- S. Kasichayanula, J. D. House, T. Wang and X. Gu, J. Chromatogr., B: Anal. Technol. Biomed. Life Sci., 2005, 822, 271–277 CrossRef CAS.
- S. Takatori, Y. Kitagawa, H. Oda, G. Miwa, J. Nishikawa, T. Nishihara, H. Nakazawa and S. Hori, J. Health Sci., 2003, 49, 91–98 CrossRef CAS.
- M. Heneweer, M. Muusse, M. Van den Burg and J. T. Sanderson, Toxicol. Appl. Pharmacol., 2005, 208, 170–177 CrossRef CAS.
- B. J. Vanderford, R. A. Pearson, D. J. Rexing and S. A. Snyder, Anal. Chem., 2003, 75, 6265–6274 CrossRef CAS.
- M. E. Balmer, H. Buser, M. D. Müller and T. Poiger, Environ. Sci. Technol., 2005, 39, 953–962 CrossRef CAS.
- G. A. Loraine and M. E. Pettigrove, Environ. Sci. Technol., 2006, 40, 687–695 CrossRef.
- X. Ye, Z. Kuklenyik, L. L. Needham and A. M. Calafat, Anal. Chem., 2005, 77, 5407–5413 CrossRef CAS.
- X. Ye, Z. Kuklenyik, L. L. Needham and A. M. Calafat, Anal. Bioanal. Chem., 2005, 383, 638–644 CrossRef CAS.
- A. M. Calafat, L. Y. Wong, X. Ye, J. A. Reidy and L. L. Needham, Environ. Health Perspect., 2008, 116, 893–897 CAS.
- L. Vidal, A. Chisvert, A. Canals and A. Salvador, J. Chromatogr., A, 2007, 1174, 95–103 CrossRef CAS.
- H. Jeon, Y. Chung and J. Ryu, J. Chromatogr., A, 2006, 1131, 192–202 CrossRef CAS.
- T. Felix, B. J. Hall and J. S. Brodbelt, Anal. Chim. Acta, 1998, 371, 195–203 CrossRef CAS.
- M. S. Wolff, S. L. Teitelbaum, G. Windham, S. M. Pinney, J. A. Britton, C. Chelimo, J. Godbold, F. Biro, L. H. Kushi, C. M. Pfeiffer and A. M. Calafat, Environ. Health Perspect., 2006, 115, 116–121 CrossRef.
- Environmental Working Group. Skin Deep Cosmetic Safety Database. http://www.cosmeticsdatabase.com/wordsearch.php%3Fquery%3Dbenzophenone, available on December 2009.
- V. Sarveiya, S. Risk and H. A. E. Benson, J. Chromatogr., B: Anal. Technol. Biomed. Life Sci., 2004, 803, 225–231 CrossRef CAS.
- Y. Nakagawa, T. Suzuki and S. Tayama, Toxicology, 2000, 156, 27–36 CrossRef CAS.
|
This journal is © The Royal Society of Chemistry 2010 |
Click here to see how this site uses Cookies. View our privacy policy here.