DOI:
10.1039/C0AY00034E
(Paper)
Anal. Methods, 2010,
2, 714-721
Development of a validated HPLC-PAD-APCI/MS method for the identification and determination of iridoid glycosides in Lamiophlomis rotata
Received
18th January 2010
, Accepted 19th March 2010
First published on
23rd April 2010
Abstract
An HPLC-photodiode array detector (PAD)-APCI/MS method was developed for the identification of iridoid glycosides in Lamiophlomis rotata (Benth.) Kudo. The regularities of characteristic ions of iridoid glycosides obtained in APCI-MS were analyzed and used to deduce the structure of iridoid glycosides. Compared with the literature on ESI-MS, the deprotonated molecular ions were more outstanding in APCI-MS. Five major active constituents, namely, 8-O-acetylshanzhiside methyl ester, loganin, 8-deoxyshanzhiside, phloyoside II, and shanzhiside methyl ester, were simultaneously determined in ten samples by HPLC. A comprehensive validation of the method included tests of sensitivity, linearity, precision, and accuracy. The linear regressions were acquired with r > 0.999. The precision was evaluated by intra- and inter-day assays, after which relative standard deviation (R.S.D.) values were reported within 2.5%. The recovery studies for the quantified compounds were observed in the range of 95.6-101.2% with R.S.D. values less than 2.1%. The overall procedure may be suitable for the qualitative and quantitative analyses of iridoid glycosides in L. rotata and its preparations.
1 Introduction
Lamiophlomis rotata (Benth.) Kudo (LR) is a perennial herb from the Labiatae family, which grows wild in the eastern Qinghai-Tibet Plateau of China. For thousands of years, the aerial parts and the roots of LR have been used as traditional drugs by the Tibetan, Mongolian, and Na–Xi nations because of the herb's beneficial effects on hemostasis and pain alleviation.1 Since 2005, Herba L. rotata has been recorded in the Pharmacopoeia of P.R. China, and preparations made of this plant have been used extensively and frequently.2 Iridoid glycosides and flavonoids are two main groups of compounds found in this plant.3–6 Flavonoids have been regarded as the analgesic activity ingredients of LR. The contents of total flavonoid and luteolin have been set as the quality specification of this plant and its preparation,2 and five flavonoids in this plant have already been simultaneously determined by CE.7 Recently, an increasing number of studies have shown that the iridoid glycosides are responsible for the hemostatic bioactivity.8,9 Therefore, the quantitation and quantification analysis of iridoid glycosides is necessary to control the quality of L. rotata.
ESI seems to be the preferred ionization method in the majority of these applications. This is probably because its application scope, in terms of physico-chemical character of the compound under investigation, is broader than that of APCI. Consequently, on starting the development of a new method, ESI ionization of the compound(s) and the intended internal standard is often investigated first. Only when ESI fails, APCI or other less common approaches, such as atmospheric pressure photoionization, are tried. Iridoid glycosides have been frequently analyzed by HPLC/MS with ESI as the electro-ionization source.10–13 In this study, an HPLC-PAD-APCI/MS method was developed for the identification of iridoid glycosides in L. rotata for the first time. Five major active constituents, namely, 8-O-acetylshanzhiside methyl ester (1), loganin (2), 8-dehydroxy shanzhiside (3), phloyoside II (4), and shanzhiside methyl ester (5), were simultaneously determined in ten samples. Their structures are shown in Fig. 1.
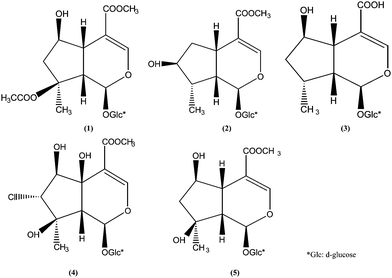 |
| Fig. 1 Structures of five marker compounds isolated and identified from L. rotata: 8-O-acetyl shanzhiside methyl ester (1), loganin (2), 8-deoxyshanzhiside (3), phloyoside II (4), and shanzhiside methyl ester (5). | |
2 Experimental
2.1 Materials and reagents
Samples of L. rotata were collected from different habitats in the Qinghai-Tibet Plateau of China in different years. These herbs were authenticated by Professor Zhigang Ma (Pharmacy College of Lanzhou University, Lanzhou, PR China). Voucher specimens were deposited at the Herbarium Center of the Pharmacy College of Lanzhou University. Specifications of the samples evaluated in the present study are shown in Table 1.
Table 1 The specifics and contents of five constituents of Lamiophlomis rotata in natural habit
No. |
Part of plant and year of harvest |
Growth site |
Content of five constituents (%) |
1 |
2 |
3 |
4 |
5 |
01 |
Aerial parts (2003) |
Maqv, Gansu |
1.19 ± 0.01 |
0.26 ± 0.00 |
1.02 ± 0.01 |
0.21 ± 0.00 |
0.85 ± 0.01 |
02 |
Aerial parts (2004) |
Maqv, Gansu |
1.95 ± 0.02 |
0.35 ± 0.01 |
0.85 ± 0.01 |
0.19 ± 0.00 |
1.23 ± 0.02 |
03 |
Aerial parts (2005) |
Maqv, Gansu |
1.54 ± 0.02 |
0.32 ± 0.00 |
0.74 ± 0.00 |
0.15 ± 0.00 |
1.01 ± 0.01 |
04 |
Aerial parts (2007) |
Maqv, Gansu |
1.04 ± 0.01 |
0.21 ± 0.00 |
0.63 ± 0.00 |
0.13 ± 0.00 |
0.82 ± 0.01 |
05 |
Aerial parts (2006) |
Linzhi, Xizang |
0.92 ± 0.01 |
0.15 ± 0.00 |
0.65 ± 0.00 |
0.04 ± 0.00 |
0.74 ± 0.00 |
06 |
Aerial parts (2007) |
Deqin, Yunnan |
1.39 ± 0.03 |
0.31 ± 0.01 |
0.72 ± 0.01 |
0.07 ± 0.00 |
0.56 ± 0.00 |
07 |
Aerial parts (2007) |
Linzhi, Xizang |
1.09 ± 0.01 |
0.22 ± 0.00 |
0.71 ± 0.01 |
0.07 ± 0.00 |
0.91 ± 0.01 |
08 |
Root (2003) |
Maqv, Gansu |
0.73 ± 0.00 |
0.10 ± 0.00 |
0.55 ± 0.00 |
0.03 ± 0.00 |
0.66 ± 0.01 |
09 |
Root (2004) |
Maqv, Gansu |
0.67 ± 0.00 |
0.09 ± 0.00 |
0.43 ± 0.00 |
0.02 ± 0.00 |
0.56 ± 0.00 |
10 |
Root (2007) |
Deqin, Yunnan |
0.72 ± 0.01 |
0.08 ± 0.00 |
0.46 ± 0.00 |
0.02 ± 0.00 |
0.53 ± 0.00 |
We used HPLC grade acetonitrile (Burdic & Jackson, Honeywell International Inc., USA), HPLC grade water obtained from a deionized water treatment system (Millipore, Bedford, MA, USA), and analytical grade acetic acid (Huada, Guangdong, China) for the preparation of the mobile phase. Meanwhile, we used analytical grade methanol (Huada, Guangdong, China) for the sample preparation. The reference compounds 1–5 were extracted, isolated, and purified from fresh L. rotata (No. 3) in our laboratory. The purified compounds were identified by APCI/MS and 1H NMR, 13C NMR spectrometric techniques, in accordance with procedures detailed in past studies.6 The purities were found to be greater than 98% based on the percentage of the total peak area using HPLC analysis.
2.2 HPLC system and conditions
Experiments were performed on a Waters apparatus (two solvent delivery systems, model 600; and a diode-array detector, model 996). On-line UV spectra were recorded from 200 to 400 nm. Data acquisition and quantification were then performed using Millenium 2.10 version software (Waters). A symmetry reversed-phase column (C18, 4.6 mm × 150 mm; particle size 5 μm, Waters, made in Ireland) was employed for the separation of the LR samples. The mobile phase consisted of acetonitrile (A) and deionized water (acetic acid adjusting pH to 5.50, B), using a gradient program of 6% A in 0–13 min, 6–14% A in 13–15 min, 14% A in 15–40 min, and 14–6% A in 40–45 min. The flow rate was 1.0 ml min−1, and column temperature was maintained at 35 °C. The detection wavelength was set at 238 nm for acquiring chromatograms. The representative HPLC chromatogram of standard compounds and samples (No. 3) are shown in Fig. 2.
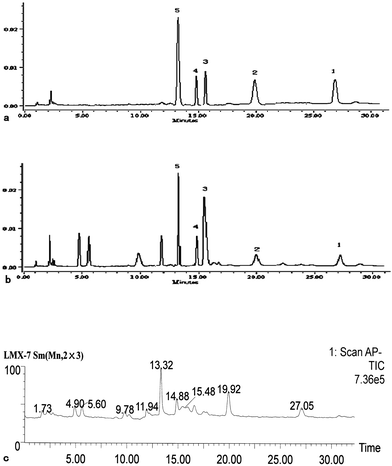 |
| Fig. 2 LC–UV chromatogram and the TICs of standard mixture and LR extract. Chromatographic conditions are described in the experimental section: (a and b) LC–UV chromatogram of the standard mixture and the LR extract recorded at 238 nm, respectively; (c) TIC of LR extracts (1) 8-O-acetylshanzhiside methyl ester; (2) loganin, (3) 8-deoxyshanzhiside; (4) phloyoside II; and (5): shanzhiside methyl ester. | |
2.3 LC-MS system and conditions
For APCI/MS analysis, all experiments were carried out in the negative ion mode using a ZMD-400 micromass spectrometer (Waters, USA) fitted with an APCI source. We used the Microsoft Windows-NT-based software LCQ Navigator to control the instrument and conduct data acquisition and processing. The vaporizer temperature was set at 450 °C, capillary temperature at 100 °C, corona at 30 eV, fragmentor voltage at 3.52 kV, cone voltage at 30 V, and electron multiplier voltage at 650 V. High purity nitrogen was used for the sheath and auxiliary gases at 2.6 exp-5 mbar and 3.1 mL min−1, respectively. We also conducted qualitative analysis in the APCI-MS scan mode (m/z 100–600). A representative HPLC chromatogram of the sample (No. 3) is shown in Fig. 2.
For the assay of the five analytes in the L. rotata herb, the standard stock solutions of 8-O- acetylshanzhiside methyl ester, loganin, 8-deoxyshanzhiside, phloyoside II, and shanzhiside methyl ester were prepared in 5.0 ml methanol for each analyte. These were then diluted with methanol to appropriate concentrations for the establishment of calibration curves. All of the standard solutions were kept at 4 °C.
The dried powders of the L. rotata herb samples (0.5 g, 0.2 mm) were accurately weighed and extracted with 25 ml 50% methanol at 60 °C for 30 min. The loss weight of the solution should be supplemented with 50% methanol and the extraction was filtered through a 0.45 μm membrane prior to HPLC injection. An aliquot of 20 μl-solution was injected in triplicate for HPLC analysis. All samples were prepared for analysis in triplicate.
2.5 Linearity
An aliquot of 20 μl-solution for each calibration standard solution was injected in triplicate for HPLC analysis. The calibration curve was constructed by plotting the peak areas versus the concentration for each analyte.
2.6 Limits of detection and quantification
The stock solutions mentioned above were diluted to a series of appropriate concentrations with methanol, and aliquots of the diluted solutions were subjected to HPLC analysis. The limits of detection (LOD) and limits of quantification (LOQ) for each analyte were determined under the present chromatographic conditions at a signal-to-noise ratio (S/N) of about 3 and 10, respectively.
2.7 Precision
The intra- and inter-day variations were chosen to deduce the precision of the developed method. For the intra-day variability test, the assays were carried out on six replicate samples (No. 3) that were extracted and analyzed in the same day. The inter-day variations were determined for three consecutive days. The quantity of each ingredient presented in this herbal sample was determined from the corresponding calibration curve, and the variations were expressed by the relative standard deviation (R.S.D.).
2.8 Accuracy
Recovery was used to further evaluate the accuracy of the method. The mixed standard solution were added to the L. rotata samples, which contents have been determined. The resultant samples were extracted and then analyzed by HPLC using the previously described procedure. The standard solutions were prepared at three different concentration levels (low, medium, and high) within the concentration range of the calibration curve. Three replicates were performed for the test.
3 Result and discussion
3.1 Optimization of the extraction procedure
Using the peak area of 8-O-acetylshanzhiside methyl ester in HPLC as the evaluation criterion, the extraction method, extraction solvent, and extraction time were investigated in an effort to optimize the extraction procedure. Every sample was prepared with 0.5 g root of LR and extracted with 25 ml solution. First, heat-extraction (30 min, 60 °C) and heat-refluxing (30 min) were compared with ultrasonic extraction (30 min, 490 W). The results showed the peak areas of 8-O-acetylshanzhiside methyl ester were 57286, 57648 and 46782 respectively. So the heat-extraction was simple and more effective. Second, different solvents including 20% methanol, 50% methanol, and 100% methanol were used with heat-extraction to evaluate the efficiency of the solvent extraction. The results showed the peak areas of 8-O-acetylshanzhiside methyl ester were 31508, 57236 and 55555 respectively. So the 50% methanol was selected as the most suitable extraction solvent. Finally, the influence of extraction time on the efficiency of extraction was also optimized. The results showed the peak areas of 8-O-acetylshanzhiside methyl ester in extraction prepared by heat-extraction with 50% methanol for 10, 30, and 60 min were 46258, 57553 and 57168 respectively. At the same time, there was no obvious difference between the results when the extraction was performed 1, 2 or 3 times on a sample under these conditions. In summary, heat-extraction of the samples in 50% methanol at 60 °C for 30 min and one time gave the best extraction efficiency. The mild nature of this extraction method could be attributed to the good water-solubility of iridoid glycosides in L. rotata.
3.2 Choice of detection wavelength for HPLC-DAD analysis
A typical feature of iridoids is the partly hydrogenated cis-fused cyclopenta-[c] pyran system, which arises from the intramolecular acetalization of a 1,5-cyclopentandialdehyde moiety and is usually stabilized by acetalization or esterification at C-4.14–16 Most of the iridoid glycosides with a carboxyl group at C-4 have a large conjugated system and demonstrate strong absorption ability around 230–240 nm (Fig. 3 and 4). Under the optimized HPLC conditions mentioned in the experimental section, 238 nm best represents the profile of the major constituents.
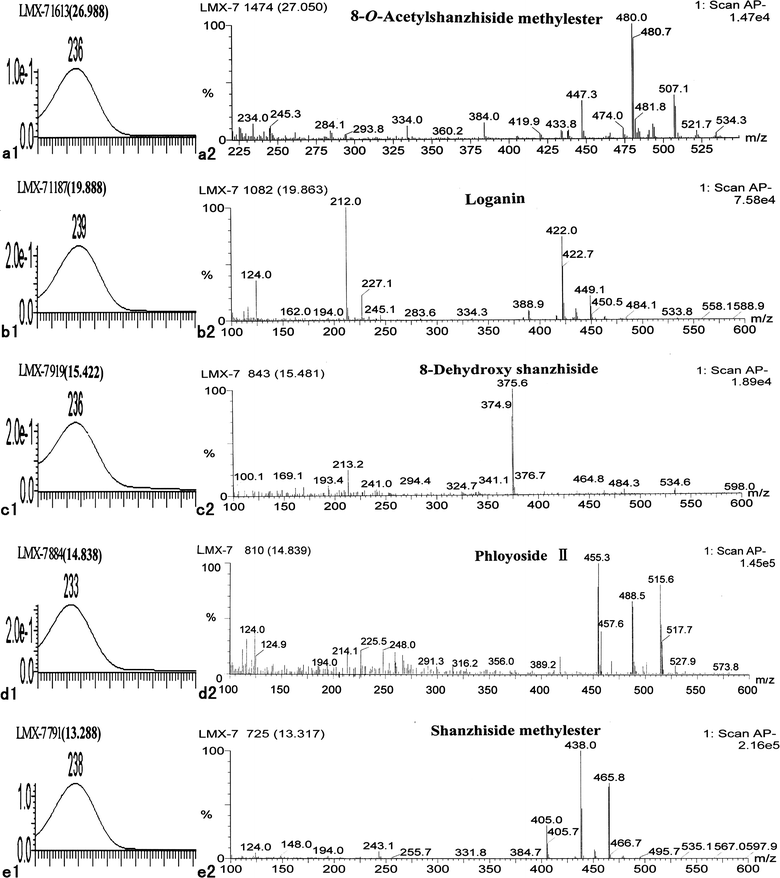 |
| Fig. 3 UV spectra and negative ion APCI mass spectra of five standard iridoid glycosides in LR: 8-O-acetylshanzhiside methyl ester (a1, a2), loganin (b1, b2), 8-deoxyshanzhiside (c1 c2), phloyoside II (d1, d2), and shanzhiside methyl ester (e1, e2). | |
3.3 Optimization of separation conditions
We optimized the chromatographic conditions in order to obtain chromatograms with a better resolution of adjacent peaks within a shorter period of time. The resolutions of these compounds were tested and compared with different reversed phase conditions using a variety of analytical columns, such as Symmetry C18 (5 μm, 150 mm × 4.6 mm; i.d.), Diamonsil TM C18 (5 μm, 250 mm × 4.6 mm i.d.), and Shim-packVP-ODS (5 μm, 150 mm × 4.6 mm i.d.). Different pH levels of the mobile phase were also optimized (Table 2). The carboxyl group at C-4 in most iridoid glycosides of L. rotata was esterified. However, the carboxyl group was free in 8-deoxyshanzhiside (3), a new compound isolated from L. rotata with a structure identified by X-ray.7 As the pH of the mobile phase was adjusted from 7.0 to 2.5, the retention time of 8-deoxyshanzhiside varied from 4.5 min to 20.5 min. At the same time, this change was not obvious in the other four compounds. As a result, a Symmetry C18 (5 μm, 150 mm × 4.6 mm; i.d.) column with acetonitrile and acetic acid aqueous solution (pH = 5.50) as the mobile phase was chosen as the preferred chromatographic conditions. Hence, gradient elution was applied. It was also suggested that the separation was better when the column temperature was set at 35 °C rather than at 20, 25, and 40 °C.
Table 2 UV absorption peaks and MS data in negative ion mode of compounds observed from the extracts of LR
Retention Time |
Name |
M.W. |
m/z |
UV λmax/nm |
[M − 1]− [M − 1 + 33]− [M − 1 + 60]− [M − 162]− [M − 162 − 59]− |
Schismoside or one of its isomers.
|
27.086 |
8-O-Acetylshanzhiside methyl ester |
448 |
447 |
480 |
507 |
286 |
227 |
236 |
19.863 |
Loganin |
390 |
389 |
422 |
449 |
228 |
169 |
239 |
15.481 |
8-Deoxyshanzhiside |
376 |
376 |
— |
— |
214 |
155 |
236 |
14.839 |
Phloyoside II |
456 |
455 |
488 |
516 |
294 |
235 |
233 |
13.317 |
Shanzhiside methyl ester |
406 |
405 |
438 |
466 |
244 |
185 |
238 |
11.924 |
Penstemoside |
406 |
405 |
438 |
465 |
244 |
185 |
238 |
9.779 |
Schismosidea |
422 |
421 |
455 |
482 |
260 |
201 |
237 |
5.617 |
Schismosidea |
422 |
421 |
455 |
482 |
— |
— |
237 |
4.939 |
Phloyoside I |
438 |
437 |
470 |
497 |
276 |
217 |
231 |
3.4 HPLC-MS identification of major iridoid glycosides in L. rotata
The major iridoid glycosides 8-O-acetylshanzhiside methyl ester (1), loganin (2), 8-deoxyshanzhiside (3), phloyoside II (4), and shanzhiside methyl ester (5) were identified using a standard mixture. For compounds 1, 2, 3, and 5, the ion fragments of [M − H]−, [M − H + 33]−, and [M − H + 60]− were outstanding and concomitant in the negative ion mass spectra (Fig. 3). The ion fragments of [M − H + 60]− could correspond to the formation of an adduct of M with the acetate anion [M + CH3COO]−. For 8-deoxyshanzhiside (5), the main ions in the MS spectra were [M − H]−, and there was no adduction of M with the acetate anion. Therefore, the outstanding and concomitant appearance of [M − H]−, [M − H + 33]−, and [M − H + 60]− could be regarded as the characteristic ion pattern that will distinguish the iridoid glycosides, which has an ester group or a carboxyl group at the C-4 position in the acetic acid buffer. In other words, iridoid glycosides with an ester group at C-4 more easily produce these three concomitant ions fragments, while the iridoid glycosides with a free carboxyl group at C-4 only produce the molecular ion [M − H]−, when acetic acid buffer was present in the negative MS mode. This observation is useful in deducing the molecular weight and the substituent group at C-4 in the identification of unknown iridoid glycosides.
According to the existing literature, ESI is more frequently used as the ionization method in the HPLC-MS analysis of iridoid glycosides; the adduction of M with the acetate anion [M − H + CH3COOH]− or the formate anion [M + HCOO]− has also been frequently observed.11–13 However, in the MS spectra of standard iridoid glycosides with an ester group at C-4, only [M + HCOO]− could be seen and the deprotonated molecular ions were either very poor or nonexistent.10 Thus, APCI may be more suitable for analyzing this kind of iridoid glycoside in HPLC/MS.
From Fig. 3, we can see two kinds of ion fragmentions in these five standard iridoid glycosides: [M − Glu]− corresponding to the loss of neutral Glu; and [M − Glu − COOCH3]− or [M − Glu − COOH]− corresponding to the sequential loss of Glu and –COOCH3 or –COOH accompanied by transfer of a proton. The UV absorption peaks and MS data in the negative ion mode of standard iridoid glycosides observed from the extracts of LR are shown in Table 2.
3.5 Deduction of unknown iridoid glycosides in L. rotata
As described above, 8-O-acetylshanzhiside methyl ester, loganin, 8-deoxyshanzhiside, phloyoside II, and shanzhiside methyl ester have been identified through the comparison with standards; however, there are still four iridoid glycosides (retention time 11.924, 9.779, 5.617, and 4.939) that remain unidentified in LR. (Fig. 4)
The UV spectra of these four compounds correspond to the characteristic profile of iridoid glycoside, and only had a strong absorption between 230–240 nm (Fig. 4). An analysis of the mass spectral data (Fig. 4) for peaks of these four compounds reveals the outstanding and concomitant appearances of [M − H]−, [M − H + 33]−, and [M − H + 60]− just as in 8-O-acetylshanzhiside methyl ester, loganin, phloyoside II, and shanzhiside methyl ester, respectively. The UV absorption peaks and MS data in the negative ion mode of these compounds are shown in Table 2. They should be the iridoid glycosides with an ester group at the C-4 position. The UV spectra and mass spectral of the peak with a retention time of 11.924 min and that of shanzhiside methyl ester are exactly alike. Even the proportions of the signal intensities of [M − H]−, [M − H + 33]−, and [M − H + 60]− are the same. In all iridoid glycosides isolated and identified from LR, penstemoside is the only isomer of shanzhiside methyl ester.6 Comparing the structure of these two compounds, only the hydroxy group at C-8 in shanzhiside methyl ester is removed to C-5 in penstemoside. Thus, the peak with a retention time of 11.924 min should be penstemoside (6) (Fig. 5). The UV spectra and mass spectra of the two compounds with retention times of 9.761 min and 5.599 min are also exactly alike. Obviously, they should be another pair of isomeric iridoid glycosides. The appearance of [M − H]−, [M − H + 33]−, and [M − H + 60]− ions reveals that the molecular weight of these two compounds should be 422. In all isolated and identified iridoid glycosides from LR, only schismoside has an M.W. of 422.8 Therefore, these two compounds should be schismoside and an isomer with an unknown structure (7, 8) (Fig. 5). The mass spectra of the peaks with a retention time of 4.939 min has a strong peak of molecular ion [M − H]− at m/z 437.0, a weak peak of [M − H + 33]− 470.0, and an even weaker peak of [M − H + 60]− 497.0. Thus, the molecular weight of this compound may be 438, which is the same as that of phloyoside I (9) as reported from LR.9 (Fig. 5).
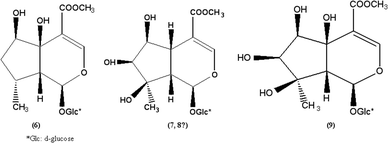 |
| Fig. 5 Structures of four iridoid glycosides deduced from L. rotata, Penstemoside (6), Schismoside (7), isomer of Schismoside with uncertain conformation (8), and Phloyoside I (9). | |
3.6 Method validation
The suitability of the developed method for its intended use can be concluded from several analytical parameters. All the compounds showed good linearity (r > 0.999) within a particular concentration range. Typical equations for the calibration are shown in Table 3. The data of LOD (based on a 20 μl injection) and LOQ for each investigated compounds are found to be below 3.5 and 11.8 ng, indicating that the method is sensitive for the quantitative evaluation of major constituents in L. rotata. Table 4 indicates that the intra- and inter-day R.S.D. values of the five compounds are all less than 2.5%, showing good precision of the developed method. Accuracy has also been determined in recovery experiments. As seen in Table 5, all the results are within the usually required recovery range of 100 ± 5%, with the maximum deviation reached for compound 3 (recovery at low spike: 95.6%) and compound 2 (recovery at high spike: 101.2%), respectively. Relative standard deviations below 2.1% indicate the method's accuracy. Considering the results, the method is considered to have good precision and accuracy.
Table 3 Calibration, linearity and limits of five iridoid glycosides (n = 6)
Iridoid glycosides |
Regression equation |
R
|
Linear range/μg ml−1 |
LOD/ng |
LOQ/ng |
8-O-Acetylshanzhiside methyl ester |
A = 23461 × C − 3507.1 |
0.9998 |
2.7073–111.0 |
3.2 |
11.6 |
Loganin |
A = 24657 × C − 1234.5 |
0.9991 |
2.6240–112.2 |
2.7 |
9.8 |
8-Deoxyshanzhiside |
A = 26275 × C − 1099.6 |
0.9991 |
2.8427–116.6 |
3.5 |
11.8 |
Phloyoside II |
A = 18528 × C − 1448.3 |
0.9992 |
2.7614–113.2 |
3.3 |
11.5 |
Shanzhiside methyl ester |
A = 22874 × C − 1351.4 |
0.9997 |
3.3535–113.2 |
2.8 |
9.4 |
Table 4 Intra- and inter-day precision of the five major constituents in Lamiophlomis rotata
Compound |
Intra-day (n = 6) |
Inter-day (n = 6) |
Day1 |
Day 2 |
Day3 |
|
Calculated content (%) a |
R.S.D. (%) |
Calculated content (%) a |
R.S.D. (%) |
Calculated content (%) a |
R.S.D. (%) |
Calculated content (%) a |
R.S.D. (%) |
Data were gram constituents per 100 g drug herb.
|
1 |
1.54 ± 0.02 |
1.3 |
1.51 ± 0.02 |
1.2 |
1.51 ± 0.02 |
1.4 |
1.51 ± 0.02 |
1.5 |
2 |
0.32 ± 0.00 |
2.0 |
0.33 ± 0.00 |
2.1 |
0.31 ± 0.00 |
2.2 |
0.31 ± 0.00 |
2.3 |
3 |
0.74 ± 0.01 |
1.6 |
0.76 ± 0.01 |
1.8 |
0.74 ± 0.01 |
1.8 |
0.74 ± 0.01 |
1.9 |
4 |
0.15 ± 0.00 |
2.2 |
0.14 ± 0.00 |
2.3 |
0.14 ± 0.00 |
2.4 |
0.14 ± 0.00 |
2.5 |
5 |
1.01 ± 0.01 |
1.4 |
1.00 ± 0.01 |
1.3 |
1.00 ± 0.01 |
1.5 |
1.00 ± 0.01 |
1.6 |
Table 5 Recoveries of the five major constituents in Lamiophlomis rotata (n = 3)
Compound |
Added/mg ml−1 |
Detected/mg ml−1a |
Recovery (%)b |
R.S.D. (%) |
Calculated by subtracting the total amount after spiking from the amount in the herb before spiking. Data is the mean result of three experiments.
Calculated as detected amount/added amount × 100%. Data is the mean result of three experiments.
|
1 |
0.005 |
0.0049 ± 0.0001 |
97.1 |
1.8 |
0.010 |
0.0096 ± 0.0002 |
96.1 |
2.1 |
0.020 |
0.0194 ± 0.0002 |
96.8 |
0.8 |
2 |
0.023 |
0.0226 ± 0.0013 |
98.3 |
1.6 |
0.046 |
0.0449 ± 0.0011 |
97.5 |
1.4 |
0.092 |
0.0931 ± 0.0021 |
101.2 |
0.9 |
3 |
0.010 |
0.0096 ± 0.0012 |
95.6 |
1.1 |
0.020 |
0.0191 ± 0.0021 |
95.7 |
2.1 |
0.040 |
0.0388 ± 0.0019 |
97.1 |
0.5 |
4 |
0.008 |
0.0079 ± 0.0001 |
98.4 |
0.8 |
0.016 |
0.0157 ± 0.0012 |
98.1 |
0.7 |
0.032 |
0.0317 ± 0.0020 |
99.2 |
0.4 |
5 |
0.05 |
0.0488 ± 0.0001 |
97.5 |
1.1 |
0.010 |
0.0096 ± 0.0002 |
96.4 |
1.3 |
0.020 |
0.0197 ± 0.0003 |
98.3 |
0.9 |
3.7 Application
The developed analytical method was also utilized to simultaneously analyze the 5 constituents in the 10 natural samples of L. rotata. Data regarding the contents calculated with external standard method are summarized in Table 1, which also shows the mean values of three parallel determinations. Peaks in the chromatograms were identified by comparing the retention times and UV spectra with those of the standards. Retention time parameters for 1–5 were 27.01, 19.87, 15.41, 14.84, and 13.29 min, respectively. There were remarkable differences among the contents of the five constituents in all samples. For example, 8-O-acetylshanzhiside methyl ester was the most dominant constituent, and its contents varied from 0.67 to 1.95%. At the same time, it can be seen that the contents of iridoid glycosides in the aerial parts were higher than in the root. Digging of the root of LR in some place has induced damage on plateau vegetation and has also resulted in desertification. From the result of this study, authors would like to suggest that the root of LR should be removed from the source of LR in the Pharmacopoeia of P.R. China.
4. Conclusion
In summary, a rapid analytical method for iridoid glycosides in Lamiophlomis rotata samples was developed by HPLC-PAD-APCI/MS. The regularities of characteristic ions of iridoid glycosides in APCI-MS were obtained and used to deduce the structure of unknown iridoid glycosides successfully. At the same time, the LC-PAD was used to quantify the iridoid glycosides in samples resulting in a protocol which is simple, sensitive, rapid and economical. This method can easily be conducted in laboratories with limited facilities such as factory laboratories and monitoring centers in China.
Acknowledgements
The authors wish to express their thanks to the financial support provided by the Gansu Province Research and Development Foundation (No.2GS054-A43-014-14) and by the Chinese Tradition Medicine Generalization Foundation of PLA (No.2006032001).
References
-
Editorial Board of China Herbal, China Herbal; Shanghai Science and Technology Press: Shanghai, 1999, p.6074-6075 Search PubMed.
-
The State Pharmacopoeia Commission of PR China, Pharmacopoeia of the People's Republic of China, vol. I, 2005 ed., Chemical Industry Press, Beijing, 2005, p. 152 Search PubMed.
- C. Z. Zhang, C. Li and S. L. Feng, Phytochemistry, 1991, 30, 4156–4157 CrossRef CAS.
- J. H. Yi, X. P. Huang and Y. Cheng, Acta Pharm. Sin, 1997, 5, 357–360 Search PubMed.
- J. J. Tan, C. H. Tan, M. Li, S. H. Jiang and D. Y. Zhu, Helv. Chim. Acta, 2007, 90, 143–148 CrossRef CAS.
- M. X. Li, Z. P. Jia, Z. D. Hu, R. X. Zhang and X. Tian, Carbohydr. Res., 2008, 343, 561–565 CrossRef CAS.
- M. N. Luo, H. W. Lu, H. Q. Ma, L. Zhao, X. Liu and S. X. Jiang, J. Pharm. Biomed. Anal., 2007, 44, 881–886 CrossRef CAS.
- M. X. Li, Z. P. Jia, Z. D. Hu, R. X. Zhang, T. Shen, H. X. Zhang and J. Wang, Phytother. Res., 2008, 22, 759–765 CrossRef CAS.
- M. X. Li, R. X. Zhang, Z. P. Jia, J. Sheng, J. G. Qiu and J. Wang, Phytother. Res., 2009, 23, 816–822 CrossRef CAS.
- L. L. Ren, X. Y. Xue, F. F. Zhang, Y. C. Wang, Y. F. Liu, C. M. Li and X. M. Liang, Rapid Commun. Mass Spectrom., 2007, 21, 3039–3050 CrossRef CAS.
- Y. Song, S. L. Li, M. H. Wu, H. J. Li and P. Li, Anal. Chim. Acta, 2006, 564, 211–216 CrossRef CAS.
- X. M. Liang, Y. Jin, Y. P. Wang, G. W. Jin, Q. Fu and Y. S. Xiao, J. Chromatogr., A, 2009, 1216, 2033–2044 CrossRef CAS.
- M. Yang, J. H. Sun, Z. Q. Lu, G. T. Chen, S. H. Guan, X. Liu, B. H. Jiang, M. Ye and D. A. Guo, J. Chromatogr., A, 2009, 1216, 2045–2062 CrossRef CAS.
- L. J. EI-Nagger and J. L. Beal, J. Nat. Prod., 1980, 43, 649–707 CrossRef CAS.
- C. A. Boros and F. R. Stemrmitz, J. Nat. Prod., 1990, 53, 1055–1132 CAS.
- C. A. Boros and F. R. Stemrmitz, J. Nat. Prod., 1991, 54, 1173–1132 CrossRef CAS.
|
This journal is © The Royal Society of Chemistry 2010 |
Click here to see how this site uses Cookies. View our privacy policy here.