DOI:
10.1039/B9AY00289H
(Paper)
Anal. Methods, 2010,
2, 354-358
Received
10th December 2009
, Accepted 21st January 2010
First published on
2nd February 2010
Abstract
Methylmalonic acid (MMA) in human serum is an important biomarker of methylmalonic acidemias. In this work, a simple and fast method based on microwave-assisted derivatization (MAD) followed by gas chromatography-mass spectrometry (GC-MS) was developed for the determination of methylmalonic acid in serum. In the proposed method, the MMA was extracted from serum and rapidly derivatized with bis(trimethylsilyl)trifluoroacetamide under microwave irradiation, finally the derivative was analyzed by GC-MS. The experimental parameters were investigated and the method validations were studied. The optimal conditions were obtained: 50 μL of bis(trimethylsilyl)trifluoroacetamide plus 1% trimethylchlorosilane (BSTFA-TMCS (99
:
1)) as derivatization regent, microwave power of 400 W and irradiation time of 2 min. The proposed method provided satisfactory precision (RSD 2.3–4.5%), wide linear range (0.021–5 mM), good linearity (R2 >0.999), and good recovery (96–101.3%). The proposed method was successfully applied to the analysis of MMA in serum samples. The experimental results showed that the proposed method was a simple, rapid, and efficient technique for the quantitative determination of MMA in serum.
1 Introduction
Methylmalonic academia is one of the most common autosomal recessive organic acidemias, which is cause by a deficiency of the activity of L-methylmalonyl-CoA mutase or cobalamin coenzyme deficiency. Attributed to that, methylmalonic acid (MMA) in the serum or plasma of the patients accumulates and the concentration of it becomes obviously higher than that under normal conditions. Monitoring of methylmalonic acid in plasma is useful in the screening, treatment and management of methylmalonic acidemia. Therefore, it is important to develop a simple, rapid and accurate method to analyze MMA in biological samples for the diagnosis of methylmalonic acidemia.
A number of analytical techniques, most of which are based on mass spectrometry, including gas chromatography-mass spectrometry (GC-MS), liquid chromatography-tandem mass spectrometry (LC-MS), tandem mass spectrometry(MS-MS) and so on, have been developed for the determination of MMA.1–5 Due to its high accuracy and sensitivity, GC-MS is more suitable for profile analysis of serum samples or plasma samples.6–10 So far, GC-MS has been widely applied to analyzing the organic acids and amino acids in serum and urine.11,12 As we know, for the GC-MS analysis of organic acids, a prior derivatization step is required, because of their high polarity and poor chromatographic properties. In general, the derivatization is performed by heating under dry conditions, which is tedious and time-consuming (about 60 min). Recently, Lankmayr and co-workers have demonstrated that microwave irradiation could remarkably accelerate the derivatization of carbonyls with O-(2,3,4,5,6-pentafluorobenzyl)hydroxylamine.13 This technique has been successfully applied to the analysis of 2,5-hexanedione, urine diuretics and glucocorticoids in human urine, chlorophenols in ash, glucose and isoleucine and 17α-hydroxyprogesterone in blood samples.14–19 In the present work, microwave-assisted derivatization followed by GC-MS was developed for the fast determination of MMA in the serum samples. The conditions of microwave-assisted derivatization and the method validation were studied.
2 Experimental
2.1 Chemicals, calibration solutions, and human serum samples
Bis(trimethylsilyl)trifluoroacetamide plus 1% trimethylchlorosilane (BSTFA-TMCS 99
:
1), and methylmalonic acid (MMA), were purchased from Sigma (St. Louis, MO, USA). Mandelic acid (used as an Internal Standard (IS)), HPLC grade methanol and analytical grade acetone were purchased from Shanghai Chemical Regent Company (Shanghai, China). A microwave chemical reactor was purchased from Nanjing Lingjiang Development Co. Ltd (Nanjing, China).
Standard stock solutions of methylmalonic acid (20 mM) was made by dissolving MMA in acetone and stored at −4 °C. Working solutions with the concentrations from 0.005 to 8 mM were prepared by diluting the 20 mM stock solution with acetone. IS solution (10 mM) was made by dissolving mandelic acid in acetone and stored at −4 °C. Blood samples were obtained from Jiangxi Phenix Hospital (Shangrao, China). The whole blood samples were collected in BD Vacutainer™ SST tubes, allowed to clot at 4 °C for 1 h, and centrifuged at 3000 rpm for 10 min at room temperature. The serum was aliquotted into Eppendorf tubes, and stored at −80 °C until use.
An HP 6890 GC system, coupled to a 5973 MSD quadrupole mass spectrometer was used in the electron impact (EI) mode. The extracted compounds were separated on an HP-5MS capillary column (30 m × 0.25 mm × 0.25 μm). A 1 μl volume of the sample was injected into the split mode with the ratio of 10
:
1. The oven temperature program was as follows. The initial temperature was 70 °C, and held for 3 min; then increased to 280 °C at a rate of 10 °C min−1, and 280 °C was maintained for 3 min. The injection temperature was 250 °C. Helium (99.999%) was used as the carrier gas with a flow rate of 1.0 ml min−1. The ionizing energy was 70 eV. All data were obtained by collecting the full-scan mass spectra within the scan range of 40–350 amu. The quadrupole temperature, transfer line temperature and MS source temperature were 150 °C, 280 °C and 230 °C, respectively.
2.3 Optimization of the microwave-assisted derivatization conditions
The microwave-assisted derivatization (MAD) parameters: the amount of derivatization agent, microwave power and irradiation time can affect the MMA derivatization efficiency, so these parameters were investigated in the study. A standard solution with a MMA concentration of 0.1 mM was used for the optimization of MAD. To begin with, the amount of derivatization agent was studied. A volume of 50 μl of the MMA solution was introduced into a 2 ml screw-capped vial. The four different volumes of BSTFA-TMCS (99
:
1) (20 μl, 40 μl, 50 μl, 60 μl) were tested under a power of 400 W and an irradiation of 4 min of the microwave parameters. Subsequently, microwave power with 200 W, 300 W, 400 W, and 500 W, and irradiation time of 1 min, 2 min, 3 min, and 5 min, were also tested. Following derivatization, 1.0 μl of derivatization products was sampled for GC-MS analysis.
In order to compare the results between microwave-assisted derivatization and conventional derivatization, the derivatization of MMA was also performed by using a conventional heating method (120 °C for 1.0 h). The calibration solution with a MMA concentration of 0.1 mM (50 μl) and 50 μl BSTFA-TMCS (99
:
1) were introduced into another vial. Instead of microwave heating, the solution was heated at 120 °C for 60 min in a dry heating block. After the derivatization, 1.0 μl was taken to be analyzed under the same conditions as described above.
2.4 Validation of the method
The optimum conditions were applied to the investigation of the linear range, precision, recovery, and detection limit of the present method. The linear range was determined by analyzing MMA at concentrations ranging from 0.005 to 8 mM by the proposed method. Five replicate measurements of calibration solution (0.1 mM) were carried out and peak area ratio of MMA to IS by each measurement was obtained. Precision was assessed by calculating the RSD of the observed values. The recovery was investigated by adding 50 μl of calibration solution (0.5 mM) to a 0.1 ml serum sample containing known concentrations of MMA. Five replicated measurements for each sample were performed. The recoveries were obtained by comparing real values of the added MMA with calculated values. The limit of detection was studied by five replicated measurements of the calibration solution with low concentration of 5μM. The limit of detection was calculated on the basis of a signal to noise ratio of 3.
2.5 Quantitative analysis of methylmalonic acid in serum samples
For each serum sample, 100 μl of serum was added into Eppendorf tubes and 50 μl of 0.1 mM IS solution was added to all sample tubes. To precipitate proteins, 0.8 ml of ice-cold methanol and 100 μl deionized water were added to the mixture. The mixture was vortex-mixed for 5 min and ultrasonicated on ice for 10 min, and then centrifuged at 12000 rpm for 10 min at 4 °C. Then 800 μl of supernatant was introduced into a 2 ml screw-capped vial, and evaporated to dryness under a stream of nitrogen at 50 °C. Finally, 50 μl acetone and 50 μl BSTFA-TMCS (99
:
1) were introduced into the vial and the residue was reacted with BSTFA-TMCS at the optimal conditions of microwave power of 400 W and irradiation time of 2 min. 1.0 μl of the derivatives was taken for the GC-MS analysis.
3 Results and discussion
3.1 Optimization of microwave-assisted derivation
The important parameters including derivatization agent amount, microwave power and irradiation time were studied. At first, in order to determine the optimal volume of BSTFA, different volumes (20, 40, 50 and 60 μl) were applied to the derivatization of MMA. The obtained results were compared and shown in Fig. 1. The results indicated that the increase of the peak areas of the derivative could be observed with the increase of volume of BSTFA from 20 to 50 μl. However, the peak areas of the derivative did not increase when volume of BSTFA increased from 50 μl to 60 μl. Thus, we chose 50 μl as the optimal volume of BSTFA. The effect of microwave power and irradiation time on MMA derivatization efficiency is shown in Fig. 2. It can be seen that the best derivatization efficiency was obtained at a microwave power of 400 W and an irradiation time of 2 min. Based on these results, a 50 μl BSTFA-TMCS (99
:
1), microwave power of 400W and irradiation time of 2 min were selected as the optimum silylation conditions, and were used in the following work.
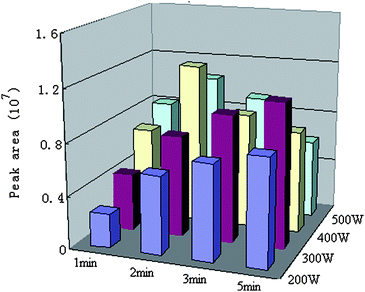 |
| Fig. 2 The effect of microwave power and irradiation time on the yields of MMA silylation. | |
In order to compare the results obtained by microwave-assisted derivatization and conventional derivatization, MMA derivatization was also performed by using conventional heating method (120 °C for 60 min). The peak area (1.23 × 107) of the MMA derivative obtained using microwave-assisted derivatization method was very close to that (1.28 × 107) obtained by conventional heating derivatization method. This shows that microwave-assisted derivatization can observably accelerate the MMA derivatization reaction, and shorten analysis time.
3.2 Validation of the method
In the work, the method validations were investigated. To obtain the method linearity, the standard solutions from 0.005 to 8 mM with 200 μM IS were analyzed by MAD followed by GC-MS. The obtained linear range for MMA was 0.021–5 mM, the calibration curve for MMA was: Y= 14.703X − 0.2547 (Y is the peak area ratio of MMA and IS, and X is the MMA concentration (mM), with a correlation coefficient (for R2) of 0.9991. This suggests that the proposed method has a good linearity. The precision of the assay was expressed by relative standard deviation (RSD) values. Five replicate analyses of the 0.1 mM calibration solution were measured by the same analytical procedure. The RSD value of 3.2% shows that the method also has good precision. In order to estimate recoveries, the added methylmalonic acid amount was calculated by using the linear equations. Recoveries obtained by comparing the real values with calculated values was 96%. The detection limit was calculated on the basis of S/N = 3 and the obtained value was 0.81 μM. Under normal conditions, the concentration of MMA in blood is very low (<0.2 mM), but it becomes significantly elevated in MMA-positive newborns (220 ∼ 2900 mM).20 This shows that the proposed method has the required sensitivity for fast screening of the methylmalonic acidemia.
The results for the linear range, detection limit, recovery and precision demonstrated that the proposed method was a reliable and sensitive method for the analysis of MMA in blood samples.
3.3 Quantitative analysis of methylmalonic acid in serum samples
To further demonstrate the proposed method, it was applied to the fast analysis of MMA in real serum samples. The artificial specimens were prepared by spiking three levels of MMA concentrations (0.02, 0.1, 2 mM) into three same serum specimen (0.1 mL) with IS (0.1 mM). MMA in the sample was extracted, derivatized and analyzed by GC-MS according to the method described in the Experiment Section. Four replicated measurements for each sample were performed. Fig. 3 shows the total ion chromatogram of (a) a calibration solution, (b) an artificial serum sample and (c) a control serum sample by MAD-GC-MS. The retention times of methylmalonic acid trimethylsilyl derivatives and IS trimethylsilyl derivatives are 9.743 and 13.441 min, respectively. In a control serum sample, the MAA was not detected. This is the same with the result in the literature.21Based on the linear equation of Y= 14.703X − 0.2547, the methylmalonic acid concentration obtained by the proposed method are 20.3, 101.6 and 2005.2 μM, respectively. The calculated RSD values were 4.1%, 2.3%, 4.5%, respectively. Recovery was also investigated by adding 50 μl of calibration solution (0.1 mM) and 50 μl of IS solution (0.1 mM) to three artificial serum sample containing known concentrations of MMA (20.3, 101.6 and 2005.2 μM). The recoveries were 99.8%, 101.3%, 98.6%, respectively. This shows that the proposed method is reliable for the fast analysis of MMA in serum samples. As the derivatization time is only 2 min, the whole analysis time is much shortened. The experimental results show that the proposed method is a simple, rapid and reliable technique for the analysis of MMA.
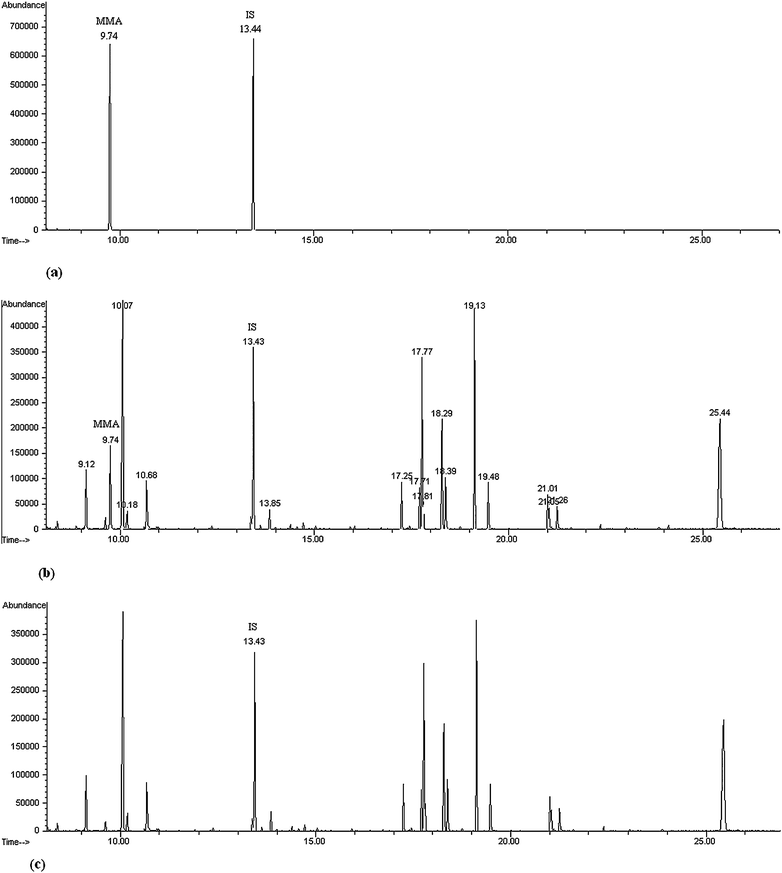 |
| Fig. 3 Total ion chromatogram of a calibration solution (50 μl of calibration solution (0.5 mM) and 50 μl of IS solution (0.2 mM)) (a), an artificial serum sample (0.1 ml serum sample containing 50 μl of calibration solution (0.1 mM) and 50 μl of IS solution (0.1 mM)) (b) and a control serum sample (0.1 ml serum sample containing 50 μl of IS solution (0.1 mM)) (c) by the MAD-GC-MS. | |
4 Conclusions
In this work, we successfully developed a microwave-assisted derivatization followed by GC-MS for the fast analysis of MMA in serum. Compared with a conventional heating derivatization method, the MMA derivatization time could be greatly reduced using the microwave assisted derivatization method. The proposed method has a wide linear range, low detection limit, good precision and good recovery. The proposed method was a rapid, simple and sensitive technique for the analysis of MMA in serum samples, and it also has potential as a clinical tool for diagnosis of methylmalonic acidemia.
Acknowledgements
This research was financially supported by the Research Program of Science and Technology from Education Department of Jiangxi Province, China (No.:GJJ08525).
References
- H. J. Blom, A. van Rooij and M. Hogeveen, Clin. Chem. Lab. Med., 2007, 45, 645–650 CrossRef CAS.
- M. Wajner, D. M. Coelho, R. Ingrassia, A. B. D. Oliveira, E. N. B. Busanello, K. Raymond, R. F. Pires, C. F. M. Souza, R. Giugliani and C. R. Vargas, Clin. Chim. Acta, 2009, 400, 77–81 CrossRef CAS.
- A. Schmedes and I. Brandslund, Clin. Chem., 2006, 52, 754–757 CrossRef CAS.
- Windelberg, O. Arseth, G. Kvalheim and P. M. Ueland, Clin. Chem., 2005, 51, 2103–2109 CrossRef.
- M. Yazdanpanah, P. C. Chan, J. Evrovski, A. Romaschin and D. E. C. Cole, Clin. Biochem., 2003, 36, 617–620 CrossRef CAS.
- C. H. Deng, N. Li and X. M. Zhang, Rapid Commun. Mass Spectrom., 2004, 18, 2558–2564 CrossRef CAS.
- C. H. Deng, W. Zhang, J. Zhang and X. M. Zhang, J. Chromatogr., B: Anal. Technol. Biomed. Life Sci., 2004, 805, 235–240 CrossRef CAS.
- R. Y. Xue, Z. X. Lin, C. H. Deng, L. Dong, T. T. Liu, J. Y. Wang and X. Z. Shen, Rapid Commun. Mass Spectrom., 2008, 22, 3061–3068 CrossRef CAS.
- R.y. Xue, L. Dong, S. Zhang, C. H. Deng, T. T. Liu, J. Wang and X. Z. Shen, Rapid Commun. Mass Spectrom., 2008, 22, 1181–1186 CrossRef CAS.
- S. Petr, H. Petr and Z. Helena, Anal. Chem., 2008, 80, 5776–5782 CrossRef CAS.
- N. Li, C. H. Deng and X. M. Zhang, J. Sep. Sci., 2007, 30, 266–271 CrossRef CAS.
- C. H. Deng, X. Y. Yin, L. J. Zhang and X. M. Zhang, Rapid Commun. Mass Spectrom., 2005, 19, 2227–2234 CrossRef CAS.
- S. Strassnig, T. Wenzl and E. P. Lankmayr, J. Chromatogr., A, 2000, 891, 267–273 CrossRef CAS.
- S. Strassnig, M. Gfrerer and E. P. Lankmayr, J. Chromatogr., B: Anal. Technol. Biomed. Life Sci., 2004, 813, 151–158 CrossRef CAS.
- L. Amendola, C. Colamonici, M. Mazzarino and F. Botre, Anal. Chim. Acta, 2003, 475, 125–136 CrossRef CAS.
- L. Amendola, F. Garribba and F. Botre, Anal. Chim. Acta, 2003, 489, 233–243 CrossRef CAS.
- M. Ramil Criado, S. Pombo da Torre, I. Rodrı'guez Pereiro and R. Cela Torrijos, J. Chromatogr., A, 2004, 1024, 155–163 CrossRef CAS.
- R. Y. Xue, S. Zhang, C. H. Deng, L. Dong, T. T. Liu, J. Y. Wang, H. Y. Wu, J. X. Gu and X.Z., Rapid Commun. Mass Spectrom., 2008, 22, 245–252 CrossRef CAS.
- C. H. Deng, J. Ji, L. J. Zhang and X. M. Zhang, Rapid Commun. Mass Spectrom., 2005, 19, 647–653 CrossRef CAS.
- F. Wang and L. S. Han, Journal of Clinical Pediatrics, 2008, 26, 724–727 Search PubMed.
- X. L. Hou and N. Qian, Chin J Contemp Pediatr., 2005, 7, 183–185 Search PubMed.
|
This journal is © The Royal Society of Chemistry 2010 |
Click here to see how this site uses Cookies. View our privacy policy here.