DOI:
10.1039/B9AY00239A
(Paper)
Anal. Methods, 2010,
2, 470-478
Comparison of extraction methods for the removal of volatile organic compounds (VOCs) present in sorbents used for human scent evidence collection
Received
30th October 2009
, Accepted 28th January 2010
First published on
23rd February 2010
Abstract
Sorbent materials used for the collection of human scent for investigative purposes have been shown to contain a variety of compounds reported as components of human scent. Many of the sorbents evaluated were biologically sterile, and it has been demonstrated that biologically sterile does not equate to “analytically clean” and the possibility exists for collected odor samples to be altered through interactions with the volatile organic compounds (VOCs) present initially on the sorbent material. The primary purpose of the study conducted was to evaluate various processes that can be applied to the sorbent materials to attain analytical cleanliness and eliminate the possibility of contamination of collected human odor samples. The chemical methods evaluated included Supercritical Fluid Extraction (SFE), Subcritical Water Extraction (SWE), traditional Soxhlet extraction, and autoclaving of sorbents. Optimization of the extraction parameters evaluated included temperature, pressure and extraction time, and the use of chemical modifiers such as methanol and water. Overall, SFE proved to be the most efficient method for producing sorbent materials free of human scent compounds.
Introduction
The use of human scent evidence as an investigative tool in several recent court cases has demonstrated its value to the field of forensic science. For a scent identification to be admitted as scientific evidence in a United States (US) court of law it must satisfy the Kelly/Frye rules of evidence. The Kelly rule, resulting from the People v. Kelly,1 applies to new scientific techniques, especially in cases involving novel devices or processes. An important US court ruling pertaining to canines and human scent was a Kelly hearing conducted in late 2004, prior to the prosecution of the State of California V. Benigno Salcido,2 an attempted murder case. In its ruling the court agreed with the People and ruled that human scent discrimination by canine can be admitted into court as evidence if the person utilizing the technique used the correct scientific procedures and the methods used by the dog handler are reliable. The task of optimizing the methods used for the collection of human scent play a key role in maintaining the reliability of this investigative technique.
Currently there is no uniform human scent evidence collection method among the various countries which utilize this technique. All collection variations utilize a sorbent as a medium for the collection of human scent, yet the materials used have not been previously optimized or standardized among the law enforcement community. Each agency uses a different type of absorbent medium varying in sterility to collect human scent evidence. For example, the FBI uses Johnson & Johnson sterile gauze while the Dutch National Police utilize King's Cotton which is a non-sterile material.3 Previous analysis of human scent through both canine and instrumental methods vary in the type of materials used and provide no scientific reasoning for the choice of collection material employed. Some canine human scent research refers to a type of “odor collecting cloth”4 yet does not specify the composition of the material. Other canine work has utilized t-shirts5 and handkerchiefs6 for odor collection with no specification on composition. Cotton is the most widely reported type of material used for human odor collection for both canines7,8 and instruments9 nevertheless no mention of the percent composition or purity of the fabrics are listed.
The majority of absorbers used for the collection of human scent in the law enforcement community are composed of varying compositions of cotton. Cotton is a type of natural fiber from the cellulose chemistry branch.10 Cellulose polymers are made of long, linear chains of glucose units without cross-linkages between the adjacent polymer chains.11 Natural fiber products are considerably more complex than man-made fibers and exhibit a greater diversity in surface chemistry, which may be exploited to optimize the absorptive potential of the textile gauze. Cellulose fibers (cottons) have a large number of hydroxyl groups, resulting in increased absorbency and affiliation for polar species via hydrogen-bonding (Fig. 1).10
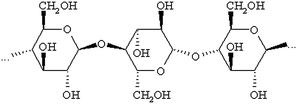 |
| Fig. 1 Natural Cotton (Cellulose) Structure. | |
Despite sterilization through common techniques such as autoclaving and gamma radiation, headspace analysis of sterile gauze pads has revealed the presence of numerous volatile organic compounds (VOCs). Biologically sterile has been shown not to equate to analytically clean,12 and consequently the collection medium may prove to be a limiting factor for the instrumental analysis of human scent profiles and thus an optimization of these materials is required. Table 1 illustrates the types of compounds commonly extracted from the various textile materials after the solid phase microextraction-gas chromatography mass spectrometry (SPME-GC-MS) analysis. It is important to highlight that some of the commercial brands under study have undergone initial sterilization methods before their distribution. However, the amount of chemical contamination observed after extraction procedures reinforces the idea that biologically sterile does not correlate to a significant chemical cleanliness.
Table 1 Compounds Present in Headspace of Sorbent Materials
Canines have shown the natural ability to discriminate between odors in the presence of a high background, whereas instrumental analysis typically requires a significantly lower background. For purposes of this study as well as other studies by the authors,12–15human scent is described as the volatile organic compounds present in the headspace of a collected odor sample. However, these compounds may not be utilized by canines for their human odor associations, but 71.43% of the compounds detected utilizing this method have been shown to have sufficient volatility for detection by canines.16 The analytical cleanness of the absorbers used for the collection of human scent for instrumental evaluation is of importance because many of the compounds present in the headspace of biologically sterile gauze have been previously reported to be components of human scent,12including various aldehydes, alcohols, and alkanes.13,14,15 For this study and previous studies by the authors, human scent is defined as the most abundant volatile organic compounds identified to be in the headspace above scent samples; however, other substances that have a relatively low volatility or are present in low concentrations may make contributions to human odor.
The popularity of supercritical fluids for pressurized extraction methods is due in large part to their unique density and solvent properties, which are very similar to those of a liquid. Appropriate control of parameters such as temperature and pressure enables the solvation power of a supercritical fluid to be adjusted. Supercritical fluids have gas-like diffusivity, low viscosity and zero surface tension; these distinctive properties allow supercritical fluids to quickly penetrate into complex sample matrices. In contrast to traditional extraction methods such as Soxhlet and steam distillations, SFE eliminates the use and costs associated with solvent disposal as well as the immediate exposure of laboratory personnel to toxic and flammable solvents.17–22
The main challenge with SFE is to adjust the best operational extraction parameters for the compounds of interest within a particular sample matrix. Critical parameters, which are important in conducting an extraction by SFE, include pressure, temperature, density, flow rate, and the use of modifiers. The fact that CO2 is relatively non-polar limits the solvating power for polar analytes. Thus, the addition of modifiers has become relatively common when performing SFE extractions.23 Modifiers, a small amount of polar organic solvent added to the supercritical CO2, are added for two major reasons. One, modifiers are added when the solubilities of target analytes are not sufficient to yield reasonable extraction rates. Two, modifiers can be added to interact with the sample and thus enhance the rate of kinetic/desorption process.24
Subcritical water extraction (SWE), also known as hot water extraction, high temperature water extraction, or superheated water extraction has also emerged as a useful extraction technique based on the use of water as the extraction solvent (critical point of water, 374 °C and 3306 psi). In the subcritical state, the polarity of water can be dramatically lowered by increasing the temperature. This reduced polarity can in turn be beneficial for the efficient removal of intermediate or low polarity compounds. The polarity can be measured in terms of the dielectric constant as a function of temperature. Unlike SFE procedures where parameters such as pressure and temperature are heavily responsible for extraction selectivity, the use of water for the extraction of polar and non-polar organics depends primarily on the extraction temperature keeping in mind the needed pressure to maintain the water solvent flow in the liquid state.25
The purpose of this paper was to evaluate the application of Supercritical Fluid Extraction (SFE) as a method of completely removing human scent VOCs from adsorbents utilized for the collection of human scent. A parallel study of the effect of steam sterilization and Subcritical Water Extraction of absorbers was also examined. The extraction parameters evaluated included: temperature, pressure and extraction time, and the use of chemical modifiers such as methanol, chloroform and water. The modifier effects demonstrated a direct dependency on extraction efficiency with modifier identity. Soxhlet extraction was also conducted using both chloroform and methanol to compare traditional extraction techniques to SFE.
Methods and materials
Materials
DUKAL Sterile Gauze Sponges 2 × 2 in, 8-Ply (Dukal Corporation, Hauppauge, NY), Johnson & Johnson Sterile Small Pads 2 × 2 in (Johnson & Johnson Consumer Company, Inc, Skillman, NJ), Eckerd Sterile Pads (Eckerd Drug Company, Clearwater, FL), Nexcare Sterile Pads (3M Health Care, St. Paul, MN), Kings Cotton, Hungarian, and Polish Absorbers (Dutch National Police). Extraction solvents: supercritical grade carbon dioxide (Airgas, Radnor, PA). HPLC grade methanol (Fischer Scientific, Pittsburgh, PA), chloroform (Fischer Scientific, Pittsburgh, PA), and deionized water. Vials utilized were 10 mL clear screw top glass vials with PTFE/Silicone septa (Supelco, Bellefonte, PA) and the solid phase micro-extraction fibers used were Divinylbenzene/Carboxen on PDMS fibers (Supelco, Bellefonte, PA).
SFC grade carbon dioxide (Airgas, Radnor, PA) was pressurized by an ISCO Model 260D Syringe Pump attached to an ISCO SFX 2–10 Supercritical Fluid Extractor (ISCO, Lincoln, Nebraska). For the development of the optimal SFE conditions DUKAL brand gauze was used exclusively as the control sorbent material, as its composition was 100% cotton whereas other materials studied ranged in their composition. All extractions were conducted in triplicate. A piece of gauze weighing ∼0.36 g was placed inside a 10 mL SFE cell (ISCO, Lincoln, Nebraska). During the evaluation of the addition of modifiers on extraction efficiency, a modifier was added directly to the extraction cell by pipeting 500 μL onto the gauze pad in the extraction cell. The extraction temperature (36 °C, 130 °C, and 150 °C) within the cell was controlled through use of an ISCO SFE Temperature Controller contained within the SFE Extractor, and an ISCO Restrictor Temperature Controller was used to maintain temperatures during the dynamic aspect of the extractions. The restrictor used had an internal diameter of 2 mm and its temperature was maintained at 120 °C during the dynamic extraction step at a fixed volume flow of 1.5 ml min−1. The effect of various pressures on the extraction efficiencies was also evaluated at pressures that included both 2500 and 4500 psi. Various static/dynamic time combinations were evaluated including 30/10 min and 45/10 min. Extraction analytes were not collected or evaluated post extraction. After the extractions the gauzes were removed from the extraction cell, placed inside a 10 mL vial (Supelco, Bellefonte, PA), and headspace analysis was then conducted using SPME-GC-MS as described later in the text. To evaluate the ruggedness of the optimal SFE conditions, varying sizes and types of materials were run through the SFE and the amount of modifier was scaled-up based on a weight ratio of 500 μL to ∼0.36 g.
Subcritical water extractions were performed using an Isco Model 260D Syringe pump as the source of constant flow mode to pump the water in the system through a 10 mL SFE plastic extraction vessel placed in a SFX 2–10 Supercritical Fluid extractor (ISCO, Lincoln, Nebraska). All extractions were conducted in triplicate. To perform the extractions, the extractor valve was closed while the water supply valve was opened to fill the pump. Water was then allowed to pump through ∼0.36 g of Dukal gauze material placed in the extraction vessel at a low flow rate to fill the cell. The system outlet valve remained closed in order to perform 30 min of continuous static flow of water solvent through the loaded sample at the evaluated pressures (1000 psi and 2000 psi) followed by a 10 min dynamic extraction which allowed the water solvent to flow through a heated restrictor out of the system. To evaluate the temperature effect on the dielectric constant of water, the extractions were performed under the same static/dynamic extraction parameters just described, at a constant pressure of 1000 psi and the three evaluated temperatures (50 °C, 90 °C, and 130 °C). The extracted materials were then placed in previously baked out 10mL beakers, allowed to dry in the oven at a temperature of 100 °C for a period of 24 h, and then transferred to 10 mL glass vial to conduct SPME-GC-MS analysis.
All Soxhlet extractions were conducted in triplicate. A piece of DUKAL gauze ∼0.36 g was extracted with ∼200 mL for 9 h using either methanol or chloroform HPLC grade solvents (Fischer Scientific, Pittsburgh, PA). After the Soxhlet extractions were completed the gauze was placed inside a previously baked out glass beaker and covered with aluminium foil for a period of 24 h to allow for solvent evaporation at room temperature, then re-placed inside the 10 mL glass vial for SPME-GC-MS analysis.
Steam sterilization
For the steam sterilization experiments, a commercial autoclave (Consolidate Stills & Sterilizers, Boston, MA) was utilized whereby the conditions were set up at a temperature of 250 °C at a pressure of 20 psi. The treatment was programmed for an autoclave process of 30 min of sterilization followed by a 10 min drying period. The absorber material tested was placed in non-sterile containers each with four loaded absorbent pieces of an approximate size of 2 in × 2 in per run.
SPME-GC-MS methods
Prior to extraction all materials were placed inside a 10 mL screw-top vial (Supelco, Bellefonte, PA) and a headspace analysis via SPME-GC-MS was conducted utilizing Divinylbenzene/Carboxen on PDMS fibers (Supelco, Bellefonte, PA). The fiber type was chosen in accordance with the optimal fiber for the extraction for human odor samples1 and the exposure time utilized was fifteen hours, which was determined previously to be the optimal extraction time for collected armpit odor samples.19 All SPME exposures were conducted at room temperature. After extraction, despite the method employed, each gauze pad was then placed inside a 10-mL glass vial for analysis and re-evaluated through the same SPME-GC-MS method. The instrumentation used for the separation and analysis of the analytes was an Agilent 6970 GC/5973 MSD. The column used was a HP5-MS, 30 meter, 0.25 μm, 0.25 mm with helium as the carrier gas (flow rate: 1.0 mL min−1). The method for the GC-MS began when analytes were desorbed in the injection port of the GC with an inlet temperature of 250 °C. The GC method begins with an initial oven temperature of 40 °C for 5 min., then ramped at 10 °C min−1 until the temperature reaches 300 °C, and held at 300 °C for 2 min. (total run time: 33 min.). The mass spectrometer used was an HP 5973 MSD with a Quadrupole analyzer in full scan mode (range: 50–550).
Results
Evaluation of sorbents
In an effort to analyze and evaluate the distinct signatures of VOCs from the different types of sorbent materials, a 15 h SPME extraction was performed for each brand studied in 10 mL glass vials at normal room temperature. Fig. 2 illustrates the number of different human scent volatile organic compounds found in the gauze pads which ultimately yielded a contaminated chromatographic background for further scent sampling purposes. The most highly contaminated brand tested was found to be Johnson & Johnson, which currently is the most common used brand by law enforcement agencies within the US. In turn, the DUKAL and Nexcare brand had the fewest VOCs. The Nexcare brand in the study disintegrated after SFE treatment, which could have been a direct effect of its initial fiber composition. This brand of sterile pads consists of a triple layer of polypropylene and wood fiber combination which may have influenced the melting of the pad at the evaluated extraction method due to possible polymer chain degradation at high temperatures. Dukal brand sterile pads were chosen as the control collection medium for the development of the SFE cleaning method due to their purity in fiber composition being that they are 100% cotton and all of the other materials analyzed were blends.
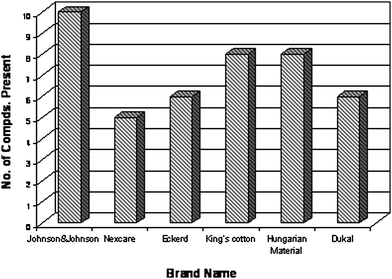 |
| Fig. 2 Brand Comparison of VOCs Extracted from Untreated Sorbents. | |
As can be observed in Table 2, the sterilization procedures vary among brands as well as the gauze pad textiles utilized in their manufacturing. In turn, these variations in initial fiber structure and material handling are important factors to consider in the extraction efficiencies of the textile in question. As a further evaluation of the different fiber structures and woven patterns found in the gauze pads tested, SEM and FESEM images were taken at magnitudes of 25×, 500×, and 2000×. The results are shown in Fig. 3–6.
Table 2 Sorbent Textile and Sterilization Comparisons
Brand Name |
Textile Material |
Initial Sterilization Method |
Dukal |
Cotton |
Gamma Radiation |
Nexcare |
Polypropylene/wood fiber |
Ethylene Oxide |
Johnson & Johnson |
Rayon/polyester/cellulose |
Autoclave/radiation |
Eckerd |
Rayon/polyester/cellulose |
Ethylene Oxide |
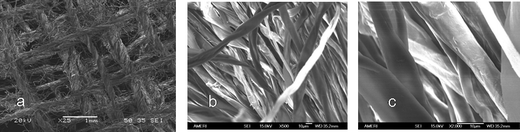 |
| Fig. 3 SEM and FESEM Images of Dukal Cotton Absorber a. 25× b. 500× c. 2000×. | |
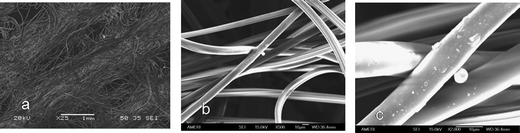 |
| Fig. 4 SEM and FESEM Images of Johnson & Johnson Gauze Absorber a. 25× b. 500× c. 2000×. | |
Static extraction time optimization
The compounds targeted for removal contain compound classes that are both polar and non-polar, thus the ability of pure CO2 may not be adequate for complete removal of the polar compounds as CO2 has high solubility for non-polar analytes. Static/dynamic extraction times of 30/10 min and 45/10 min at (130 °C and 4500 psi) were evaluated without the presence of the modifiers to evaluate the effect of static extraction time on extraction efficacy as can be seen in Fig. 7. While the extended exposure to the carbon dioxide solvent removed 100% of the target long chain alkanes such as dodecane, tridecane, hexadecane, and heptadecane; the removal of the aldehydes were not as successful. As can be observed in Table 3, the longer static extraction time demonstrated minimal extraction recoveries when compared to the shorter extraction period for the polar compounds. Nonanal, for example, had a removal rate of 68.1% at 30 min compared to 63.3% at the 45 min period. Furthermore, decanal, another key human scent component showed a relatively high 92.3% removal rate at the 30 min static extraction period compared to a lower 66.7% removal rate at the prolonged static extraction time. It is important to note that there was a high variation within the results obtained of the triplicate samples tested, possibly due to a variable fiber composition among the sorbent materials. However, the results reinforce the fact that the 45 min extraction provides minimal extraction recoveries and thus low removal rates of target human scent volatile organic compounds found within sorbent material evaluated. The complete removal of the non-polar compounds, yet the persistence of the polar compounds through both extraction times evaluated demonstrates that there is a solubility impediment in the complete removal of the aldehydes from the sample matrix. The application of polar modifiers to the extraction cell can enhance the solubility of the polar compounds of interest and thus enhancing the ability to attain complete removal of all compounds from the matrix.
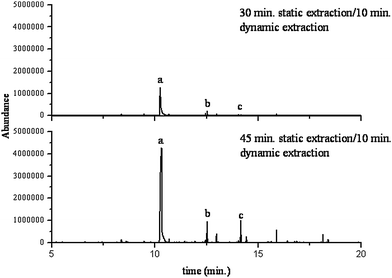 |
| Fig. 7 Extraction Time Comparison Sterile Dukal Cotton Absorber a. phenol b. nonanal c. decanal. | |
Table 3 Human Scent VOC Extraction Rates for SFE, Soxhlet, SWE, and Steam Methods
Avg. SFE % Removal on Dukal Sterile Cotton Material |
Target Compd. |
130 °C, 4500 psi (%RSD) |
36 °C, 30 min (%RSD) |
4500 psi, 30 min (%RSD) |
130 °C, 4500 psi, 30 min (%RSD) |
30 min static |
45 min static |
2500 psi |
4500 psi |
36 °C |
130 °C |
150 °C |
MeOH |
MeOH–water |
chloroform |
water |
Nonanal |
68.1(81) |
63.3(82) |
96.6(0.1) |
78.0(0.4) |
78.0(0.4) |
68.1(81) |
84.2(26) |
100(0) |
100(0) |
93.1(13) |
90.0(17) |
Decanal |
92.3(14) |
66.7(86) |
91.6(0.2) |
76.8(27) |
76.8(27) |
92.3(14) |
86.6(22) |
100(0) |
74.3(60) |
77.9(49) |
87.0(26) |
Extraction Method Comparison of Avg.% Removal on Dukal sterile Cotton Material |
Target Compd. |
SFE |
Soxhlet (9 h) |
SWE |
Sream |
30 min, 4500 psi, 130 °C, MeOH |
MeOH |
Chloroform |
1000 psi, 30 min |
130 °C, 30 min |
50 °C |
90 °C |
130 °C |
1000 psi |
2000 psi |
Nonanal |
100(0) |
94.5(10) |
75.1(19) |
100(0) |
100(0) |
100(0) |
100(0) |
100(0) |
62.8(0) |
Decanal |
100(0) |
75.6(32) |
74.6(26) |
100(0) |
38.0(138) |
100(0) |
100(0) |
96.8(6) |
59.8(15) |
Another method to increase the solubility of the CO2 solvent is to increase the pressure at a constant temperature. In this case the temperature was held at 36 °C (as the critical point for carbon dioxide gas is 31.1 °C), and the extraction efficiencies were measured at pressures of 2500 and 4500 psi, and optimized extraction period (30 min static and 10 min dynamic). No additional modifiers were added to the samples to evaluate the extraction capacity of the supercritical carbon dioxide at the specified pressure.
The results, observed in Table 3, indicate that the increase in pressure did not increase the removal rates of the target organic compounds in the sample matrix. Reported RSD values are based on the extraction recoveries of three consecutive samplings. This result was not expected since increasing the pressure at a constant temperature greatly increases solvent strength of CO2 and thus increases the solubility of most analytes found within the sample matrix. The low temperature (36 °C) at which both type of extraction pressures were evaluated may have affected the results obtained, as the material extracted could have been affected by solid CO2 found in the sample after extraction procedures indicating that perhaps the temperature within the extraction vessel dropped below the critical temperature. Perhaps, the increase of pressure could have rendered better removal rates if conducted at higher temperatures. The removal of target human scent hydrocarbons, however, was greater at the higher pressure. These compounds included tetradecane, pentadecane, and undecane as they were not seen in the chromatographic analysis of the extracted samples. However, the target volatile aldehydes showed more variable results which yielded contrasting results to the expected hypothesis. Nonanal showed a 96.6% removal rate at the lower pressure of 2500 psi while dropping to a removal rate of 78.0% at the higher pressure. Decanal was found to be removed at a rate of 91.6% at the lower pressure compared to a lower value of 76.8% removal rate at 4500 psi of pressure. Solubility limitations as well as kinetic/desorption interactions between the target compound and the matrix could have affected the elution of the compounds of interest. Furthermore, the use of pure CO2 as an extraction solvent for polar analytes may be overcome with the addition of polar modifiers.
Effect of temperature
Varying the temperature during SFE affects both the density of the fluid and the volatile property of the analytes. An increase in extraction efficiency with increasing temperature is dependent on molecular weight as well as the vapor pressure of the analytes. Thus, analytes with no significant vapour pressure will show a decreased solubility by an increase in temperature at constant pressure due to the decrease in carbon dioxide density.15 Again utilizing the static/dynamic time of 30/10min along with a pressure of 4500 psi, three temperatures (36, 130, and 150 °C) were evaluated. The results show that increasing the temperature up through 150 °C was beneficial for nonanal, however, decanal yielded much better results at a temperature of 130 °C vs. 150 °C. Also, a lower temperature of 36 °C provided nonanal with a much higher removal rate (78.0%) compared to the amount removed at 130 °C (68.1%). Decanal, on the other hand, was observed to give the best removal rate (92.3%) at the 130 °C temperature value. In all the extractions performed, decanal proved to be the hardest target VOC to be removed from the sample matrix, thereby giving the 130 °C temperature an advantage to optimize overall extraction efficiency. Even though alcohols such as phenol, a less frequently occurring human scent compound in untreated sorbents, still remain in high abundance at the 130 °C temperature, the addition of modifiers could enhance its removal as later described due to the polarity of the hydroxyl group. However, regardless of the fluctuating results obtained for the two target human scent aldehydes contaminating sorbent material, the overall trend showed that the increase of temperature was beneficial for the removal rates as shown in Fig. 8. Overall, however, none of the conditions evaluated resulted in the complete removal of human scent VOCs.
Effect of modifiers
Due to the non-polar characteristics of the CO2, there is an inherent limited ability to dissolve polar analytes from the matrix. The use of modifiers enhances the extraction when the solubility of the target analytes is not sufficient to yield reasonable extraction rates (solubility hindrances), and can also enhance the rate of kinetic/desorption process resulting in a greater interaction with the sample matrix. Thus, different modifiers were evaluated and their relation to extraction efficiencies of target compounds found in sorbent materials was determined. The extractions performed with each modifier at a static/dynamic timeframe of 30/10min were conducted at a fixed pressure and temperature of 4500 psi, 130 °C respectively. The modifiers were all spiked directly onto the gauze while inside the extraction cell and the solvents evaluated included methanol, chloroform, HPLC water, and a methanol–water combination where 5% water by weight (50 μL) along with 500 μL of methanol was used.
For all modifiers studied, long chain alkanes such as tridecane, undecane, pentadecane, hexadecane, and heptadecane were completely removed from the sample matrix. The percent removed for nonanal and decanal, the main polar compounds of interest, can be seen for the various modifiers in Table 3. Methanol demonstrated 100% removal of the polar compounds of interest. The methanol–water combination did not produce the same removal efficiency, i.e. the percent removal of decanal drops to 74.3%. When water is used as the only modifier in the extraction, the removal rates improve slightly than those of the MeOH–water mixture modified samples. This strongly indicates that the combination of water with methanol a less basic solvent reduces the extraction capability of the modifier. Chloroform (more similar to water when located in Snyder's triangle) generated a lower removal percentage for decanal (77.9%) compared to nonanal (93.3%). Water and chloroform both of which are similarly clustered in Snyder's triangle do not appear to be good solvent choices for these key aldehydes.
Two different pressures were evaluated (1000 psi and 2000 psi) while maintaining a fixed extraction temperature of 130 °C. All extractions were performed at 30 min of continuous static flow of water solvent through the loaded sample at the indicated pressures. To evaluate the temperature effect on the dielectric constant of water, the extractions were performed at a low temperature of 50 °C, 90 °C, and a much higher temperature reaching 130 °C. When using SWE, the absorptivity of the material can only be enhanced by increasing temperature or contact time, thus static extraction was the mode utilized. However, it is important to consider that increasing temperature decreases water's dielectric constant thus reducing polar- or middle-polar-analyte extraction rate and could also provoke analyte degradation at high temperature. The results obtained at the three different temperatures evaluated demonstrate that an increase of temperature from 50 °C to 90 °C decreased the percent removal rate of the polar organic aldehyde decanal by 62% as can be seen in Table 3. This decrease of removal rate seen in decanal is supported by the polarity reduction theory stated above. However, at the high temperature of 130 °C, both nonanal and decanal exhibit a 100% removal rate regardless of the decrease of the polarity of the water solvent.
The increase in pressure provided minimal improvements in the overall removal of the target compounds. This result was expected as increasing the pressure has a limited influence on the solvent characteristics of water and does not achieve significant changes in polarity. As demonstrated in Table 3, target human scent aldehydes yielded 100% removal rates at a moderate pressure of 1000 psi. An increase of pressure of 1000 psi resulted in a minimal drop in the removal rate of decanal (3.2%) as was to be expected since no change of polarity in the water solvent was anticipated thus corroborating the notion that pressure is not a critical parameter in this procedure.
Table 3 shows Soxhlet extraction rates compared to SFE, SWE, and steam methods. The nine hours of Soxhlet extraction gave minimal recoveries when compared to the much faster 30 min SFE procedure. Also, it reduced the amount of chloroform and methanol solvent needed to carry out the extraction, thereby reducing the potential exposure to toxic organic solvent. Table 3 exhibits the results of the Soxhlet extraction versus the SFE conditions described above, but with methanol as the solvent employed. The data obtained suggest that the much shorter 30 min SFE extraction achieves 100% removal rates of both nonanal and decanal, two of the target human scent aldehydes, which are typically the most difficult compounds to remove. However, when compared to the nine hour methanol Soxhlet extraction conducted, nonanal was only removed 94.5% and decanal was removed to a level of 75.6%. The longer exposure to the methanol solvent in the Soxhlet extraction proved to yield minimal removal rates when compared to the SFE method developed. The second type of Soxhlet extraction solvent (chloroform) study reinforced the disadvantage seen by the liquid solvent extraction method in contrast with the SFE method. The nine hour Soxhlet extraction yield a 75.1% removal rate for nonanal and a 74.6% removal rate for decanal thereby resulting in a decreased extraction efficiency compared to the methanol solvent. Even though only two solvents were evaluated using the liquid solvent extraction, in both comparisons the SFE methodology not only yielded an advantage in extraction time, but also in the highest removal rates of key target compounds.
Steam sterilization
A parallel study was conducted to evaluate the common steam sterilization (frequently referred to as autoclaving). Steam sterilization is normally used for the treatment of surgical dressings such as gauze pad materials. Dukal, an initially sterile material and Kings cotton, an initially non-sterile material were autoclaved through steam sterilization. This technique has the advantage of rapid penetration with the destruction of all viruses and bacteria. The additional drying cycle was used to dry the materials before removal. The temperature was set at 250 °C and a pressure of 20 psi, for a total of a 30 min run followed by a 10 min drying period. Table 3 summarizes the comparison between the SFE method and autoclave treatments and their effect on the removal of target human scent aldehydes from the two types of materials studied. The target long chain alkanes found in the collection medium studied such as tridecane, tetradecane, and undecane were effectively removed under SFE conditions, however, autoclave process yielded minimum removal results for target human scent aldehydes. Regardless of initial sterilization conditions of material evaluated, the SFE methodology yielded complete extraction of compounds; whereas, the autoclave treatment yielded minimal removal rates. Fig. 9 depicts Dukal and Kings Cotton after extraction procedures and autoclave treatment thus reinforcing the conclusion that autoclaving does not efficiently remove target VOCs and that supercritical fluid extraction yields a clean absorber material with the original adsorbent VOCs removed to below the detection limit of the method employed for human scent detection.
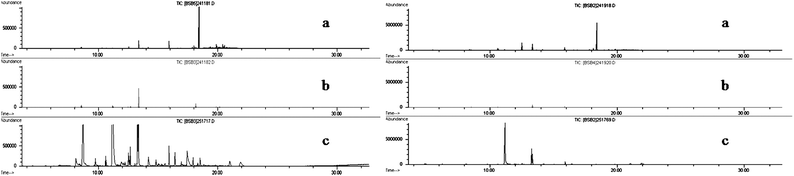 |
| Fig. 9 Comparison of SFE vs. Autoclave Treatment Left: Dukal Brand Sterile Material Right: King's Cotton Non-Sterile Material a. Prior to Treatment b. After SFE method c. After Autoclaving. | |
Method discussion and comparison
After evaluation of all the extraction methods on the sorbent materials of interest, the results of each experiment conducted clearly demonstrate the advantage of SFE over other extraction methods evaluated. When comparing SFE to traditional liquid solvent extractions, it was evident from experimental results that the choice of solvents employed for liquid extraction yielded minimal removal of the compounds in question. The organic solvents evaluated represented both prolonged and less efficient sample extractions and more toxicity to the laboratory environment.
The parallel study carried out using subcritical water extraction tested parameters such as temperature and pressure of which both presented efficient results, however the lengthy sample handling time from pre-treatment to after-treatment presented a disadvantage when compared to SFE. Both Soxhlet and steam sterilization experiments yielded much lower removal rates of target organic compounds, and yielded a more prolonged sample analysis time thus providing inefficient treatment to sorbent material tested. Additionally, obtaining a wet sorbent material after Soxhlet treatment presents contamination issues upon drying not observed with SFE which yields a sorbent that is ready to use. The optimized choice of modifier in SFE treatment generated complete removal of the target compounds with a short analysis time. When SFE is compared to subcritical water extraction the major experimental differences include the lack of an added modifier in the sample vessel and additional drying of the materials post-extraction. However, the solvating power of water was demonstrated by an efficient removal rate that paralleled the results of the SFE analysis which implies a lower solvent cost and thus an apparent advantage to the more expensive supercritical grade carbon dioxide gas implemented in the SFE procedures. Overall, however, the analysis time of SWE was significantly higher due to the need to dry the material after extraction. Table 4 summarizes the important issues to consider when developing the optimal method for the pre-treatment of sorbent material prior to utilization as human scent collection mediums.
Table 4 Method Development Comparison Chart
|
Liquid extraction (Soxhlet) |
Supercritical Fluid extraction |
Subcritical water extraction |
steam sterilization (Autoclave) |
Solvent/Modifier Selection |
Must choose a solvent system based on boiling point criteria |
Must choose modifier based on analyte polarity and active site consideration of matrix |
No added solvent is utilized in system |
No solvent use |
Temperature and Pressure |
Temperature is important; affects solubility. Pressure generally not as critical. |
Both temperature and pressure have a critical effect on solvating power. |
Temperature increase is quite important for increasing solvating power of water solvent; pressure maintained to keep water in liquid form. |
High temperature and low pressures are essential for maintaining water vapors running throughout the chamber. |
Time Required |
9 h (extraction) 24 h drying period |
40 min |
40 min > 24 h drying |
40 min |
Method Efficiency |
Somewhat efficient; long handling time |
Highly efficient, rapid handling time |
Highly efficient, long handling time |
Least efficient, rapid handling time |
Conclusions
Biologically sterile sorbents were previously reported by the authors not to equate to analytically clean, and thus the collection medium proves to be a limiting factor in the instrumental analysis of human scent. These experiments were conducted with the objective of producing an analytically clean sorbent material for the purpose of collecting human scent samples more reproducibly by minimizing interferences. The chemical methods evaluated included Supercritical Fluid Extraction (SFE), Subcritical Water Extraction (SWE), traditional Soxhlet extraction, and autoclave re-sterilization. An optimum set of SFE conditions was developed which resulted in the complete removal of VOCs from sorbents used in human scent collection. The use of a 30 min static extraction time followed by a 10 min dynamic extraction time with an extraction temperature of 130 °C, a pressure of 4500 psi, and the direct spiking of methanol in the extraction vessel, resulted in a rapid and efficient extraction procedure. This SFE method proved to remove the target aldehydes such as decanal and nonanal, as well as long chain hydrocarbons such as tetradecane, pentadecane, hexadecane, and heptadecane from the sample matrix.
The ruggedness of the developed SFE method was confirmed through trials utilizing other materials of varying composition as well as thickness and initial sterilization condition. It is concluded that with the developed SFE methodology as a pre-treatment of all sorbent materials employed for human scent collection, a more reliable collection medium has been achieved thus aiding human scent identification in becoming a more valuable evidentiary tool within the criminal justice system.
Notes and references
-
People v. Kelly, 1976 17 Cal. 3d. 24 Search PubMed.
-
People v. Salcido, 2005 Cal. App. 2nd Search PubMed.
-
G. A. A. Schoon and Ruud Haak, K9 Suspect Discrimination. Detselig Enterprises Ltd, Canada:., 2002 Search PubMed.
- J. Szinak, International Criminal Police Review, 1985, 386, 58–63 Search PubMed.
- H. Kalmus, The British Journal of Animal Behaviour, 1955, 3, 25–31 Search PubMed.
- P. G. Hepper, Perception, 1988, 17(4), 549–554 CrossRef CAS.
- R. H. Settle, B. A. Sommerville, J. McCormick and D. M. Broom, Anim. Behav., 1994, 48(6), 1443–1448 CrossRef.
- G. A. A. Schoon, J Forensic Sci, 1998, 43(1), 70–75 CAS.
- B. A. Sommerville, J. P. McCormick and D. M. Broom, Pestic. Sci., 1994, 41(4), 365–368 CrossRef CAS.
-
S. B. Warner, in Fiber Science, Prentice Hall, New Jersey, 1995 Search PubMed.
-
C. J. Kim, in Textile Science: An Outline, Kendall/Hunt Publishing Co, Iowa, 2000 Search PubMed.
- A. M. Curran, S. I. Rabin, P. A. Prada and K. G. Furton, J Chem Ecol, 2005, 31
:
7, 1613–1625.
- A. M. Curran, S. I. Rabin and K. G. Furton Forensic, Science Communications, 2005, 7
:
2 Search PubMed.
-
A. M. Curran, P. A. Prada, A. A. Schoon, J. R. Almirall and K. G. Furton, Proceedings from The International Society for Optical Engineering, SPIE Biometric Technology for Human Identification II Meeting Vol. 5779, Orlando, FL March 2005.
- A. M. Curran, P. A. Prada and K. G. Furton, J. Forensic Sci., 2010, 55(1), 50–57 CrossRef CAS.
- A. M. Curran, C. F. Ramirez, A. A. Schoon and K. G. Furton, J. Chromatogr., B: Anal. Technol. Biomed. Life Sci., 2007, 846, 86–97 CrossRef CAS.
-
C. Huang, Masters Thesis, Florida International University, 1994 Search PubMed.
- C. Radcliffe, K. Maguire and B. Lockwood, J. Biochem. Biophys. Methods, 2000, 43, 261–272 CrossRef CAS.
- M. Zougagh, M. Valcarcel and A. Rios, TrAC, Trends Anal. Chem., 2004, 23, 399–405 CrossRef CAS.
- M. C. Henry and C. R. Yonker, Anal. Chem., 2006, 78, 3909–3915 CrossRef CAS.
- K. A. Abbas, A. Mohamed, A. S. Abdulamir and H. A. Abas, Am J Biochem Biotechnol, 2008, 4
:
4, 345–353 Search PubMed.
- M. Mukhopadhyay, J. Chem. Technol. Biotechnol., 2009, 84, 6–12 CrossRef CAS.
- L. T. Taylor, Anal. Chem., 1995, 67, 364A–370A CAS.
-
S. B. Hawthorne and J. W. King, in Practical Supercritical Fluid Chromatography and Extraction., ed. Marcel Caude, Didier Thiebaut, Harwood Academic Publishers, Australia, 1999, pp.219–282 Search PubMed.
- J. H. Kimberly, L. Mazeas, C. B. Grabanski, D. J. Miller and S. B. Hawthorne, Anal. Chem., 1996, 68, 3892–3898 CrossRef CAS.
|
This journal is © The Royal Society of Chemistry 2010 |
Click here to see how this site uses Cookies. View our privacy policy here.