Enantioanalysis of S-Ibuprofen using [5–6] fullerene-C70 and diethyl (1,2-methanofullerene C70)-71-71-dicarboxylate
Received
11th March 2009
, Accepted 28th October 2009
First published on
17th November 2009
Abstract
[5–6] Fullerene-C70 and diethyl (1,2-methanofullerene C70)-71-71-dicarboxylate are used as chiral selectors for the construction of enantioselective, potentiometric membrane electrodes used in the enantioanalysis of S-Ibuprofen. The sensitivities (slopes) of the electrodes were 55.70 mV/pS-ibuprofen for [5–6] fullerene-C70 based electrode and 56.10 mV/pS-ibuprofen for diethyl (1,2-methanofullerene C70)-71-71-dicarboxylate based electrodes and the linear concentration ranges were between 10−10 and 10−3 mol L−1 (limit of detection 1 × 10−11 mol L−1), and between 10−10 and 10−4 mol L−1 (limit of detection 5.4 × 10−11 mol L−1), respectively. The recovery tests proved that these electrodes can be reliable used for the enantioanalysis of S-ibuprofen raw material (recovery higher than 99.00%) and from its pharmaceutical formulations (recovery higher than 98.40%).
Introduction
Chiral recognition has become a very important concept in biological, chemical and pharmaceutical sciences.1 It implies a set of weak forces of interactions ranging from hydrogen bonding to van der Waals interactions through to ionic and dipole–dipole interactions.2 Keeping this in mind, it seems obvious that artificial drugs have to be chiral to be integrated correctly into the vast and complex game of molecular interactions of living systems, although there are a lot of drugs which are not chiral and have the proper effect on the body.3 Thus, it is of great importance to develop separation and sensing systems to discriminate enantiomers, in parallel to asymmetric synthesis methodologies. Also, because the presence of the unwanted enantiomer can reduce the activity of the main one, or can produce secondary effects, or is without any pharmacological effect—this results in an inefficient treatment of the patient.
In the past few decades, many chiral selectors have been used in chiral separation. High performance liquid chromatography (HPLC),4 gas chromatography (GC),5 and capillary electrochromatography (CE),6 were used in the pharmaceutical industry for enantioseparations.
The use of electrochemical techniques offers for many enantioanalysis great advantages such as simplicity, high sensitivity, rapidity, accuracy and precision of analytical information, as well as low cost of analysis.7,8 The design and construction of such electrodes require the impregnation of a chiral selector in the carbon paste matrix. Different types of chiral selectors such as cyclodextrins,9 crown ethers,10 maltodextrins11 and antibiotics12 were used for enantioanalysis.
A literature survey on C70 fullerenes proved that since their discovery, they were mostly used for studies related to their electrochemistry,13–16 and possibilities of their utilization in drug design.17–19
Ibuprofen (Ibu), (R,S)-2-(4-isobutylphenyl) propionic acid (Fig. 1), is an anti-inflammatory analgesic and antipyretic drug widely used in the treatment of rheumatic disorders, musculoskeleton, fever, acute and chronic pain.20 This drug is normally administrated as the racemate, but only the S-enantiomer is responsible for the pharmacological effect.21 To date, chromatographic and spectrometric techniques have been reported for the analysis of ibuprofen.22–24
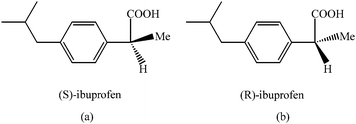 |
| Fig. 1 Ibuprofen (a) S-Ibuprofen; (b) R-Ibuprofen. | |
In this paper, we proposed two enantioselective, potentiometric membrane electrodes (EMPEs) based on [5–6] fullerene-C70 and diethyl (1,2-methanofullerene C70)-71-71-dicarboxylate as chiral selectors, for the enantioanalysis of S-ibuprofen. Modified carbon paste proved to be a very reliable matrix for the design of other EPMEs.7 Therefore, we proposed it for the electrodes' design.
Experimental
Reagents and materials
[5–6] Fullerene-C70 (I) and diethyl (1,2-methanofullerene C70)-71-71-dicarboxylate (II) were supplied by Fluka (Buchs, Switzerland). Phosphate buffer of pH 5.30 was supplied by Merck (Darmstadt). Deionized water from a Modulab system (Continental Water Systems, San Antonio, TX, USA) was used for all solutions preparation. The S- and R-ibuprofen solutions necessary for characterization of the enantioselective, potentiometric membrane electrodes were prepared from standard S-and R-ibuprofen solutions (10−2 mol L−1), respectively, by serial dilutions. All standard and diluted solutions were buffered with phosphate buffer (pH 5.30) using the ratio of buffer: distilled water, 1
:
1 (v/v).
Myprodol capsules (200 mg ibuprofen/capsule) and Nurofen tablets (200 mg ibuprofen/tablet) were purchased from Nutrent (Sandton, South Africa).
Apparatus
A PGSTAT 12 and software (Eco Chemie version 4.9) were used for all potentiometric measurements. An Ag/AgCl (0.1 mol L−1 KCl) served as the reference electrodes in the cell.
The design of the enantioselective, potentiometric membrane electrodes.
Paraffin oil, graphite powder, and the chiral selector (Fullerene-C70 (I) or diethyl (1,2-methanofullerene C70)-71-71-dicarboxylate (II)) were mixed to give the modified carbon paste. A certain quantity of carbon paste free of chiral selector was prepared and it was placed into a plastic pipette peak leaving 3 to 4 mm empty on the top to be filled with the carbon paste that contains the chiral selector. The diameters of the EPMEs were 3 mm. Electric contacts were obtained by inserting an Ag/AgCl wire in the carbon paste. A solution of 0.1 mol L−1 KCl were utilized as the internal solution. The surface of the electrodes were washed with deionized water and polished with alumina paper (polishing strips 30144-001, Orion) before using them for each experiment.
Recommended procedures
Direct potentiometry.
The potentiometric technique was used for all potential measurements. The electrodes were placed into the stirred standard and samples' solutions for which the potential E(mV) was measured. Graphs of E(mV) versus pS-ibu (pS-ibu = −log[S-ibu]) were plotted for the standard solutions (Fig. 2). The unknown concentrations of ibuprofen were determined from the calibration graphs by interpolation method.
![Calibration graph of the enantioselective, potentiometric membrane electrodes based on: 1 - [5–6] fullerene-C70 and 2 - diethyl (1,2-methanofullerene C70)-71-71-dicarboxylate.](/image/article/2010/AY/b9ay00086k/b9ay00086k-f2.gif) |
| Fig. 2 Calibration graph of the enantioselective, potentiometric membrane electrodes based on: 1 - [5–6] fullerene-C70 and 2 - diethyl (1,2-methanofullerene C70)-71-71-dicarboxylate. | |
Content uniform assay of ibuprofen Myprodol capsules and Nurofen tablets.
Each of the five capsules and five tablets were placed into 100 mL calibrated flask, dissolved and diluted to the mark using a phosphate buffer (pH 5.34)
:
deionized water 1
:
1 (v/v) solution. The unknown concentration of S-ibuprofen was determined using the direct potentiometric method.
Results and discussion
Fig. 2 shows the calibration graphs for the proposed electrode, where the functional relation between the potential, E measured at I = 0 (chronopotentiometry at zero current), and −logC (where C is the concentration of the enantiomer) gives the electrode function, E = f(−logC). Near-Nernstian and linear responses were obtained only for S-ibuprofen (Fig. 2). No linear and non-Nernstian response was obtained for R-ibuprofen. The response characteristics exhibited by these electrodes are summarized in Table 1. The correlation coefficients obtained for the equations of calibration were 0.9999. The limits of detection were of 10−11 mol L−1 magnitude order. While the relative standard deviation of the slopes (S) of the electrodes was less than 1%, the relative standard deviations of standard potentials (Eo) were 2.5 and 1.3% for the electrodes based on [5–6] fullerene-C70 and 2 - diethyl (1,2-methanofullerene C70)-71-71-dicarboxylate, respectively.
EPME based on |
Slope (mV/decade of concentration) |
Intercept, Eo/mV |
Linear concentration range/mol L−1 |
Limit of quantification/mol L−1 |
Detection limit/mol L−1 |
All measurements were made at room temperature; all values are the average of 10 determinations.
|
I |
55.7 ± 0.2 |
610.5 ± 0.9 |
10−10–10−3 |
10−3 |
1 × 10 × 10−11 |
II |
56.1 ± 0.1 |
575.8 ± 0.8 |
10−10–10−4 |
10−4 |
5.4 × 10−11 |
The response time for the EPME based on (I) was 1 min for concentrations between 10−10 and 10−6 mol L−1 and 30 s for concentrations between 10−5 and 10−3 mol L−1. For the EPME based on (II), the response time is 1 min for the concentrations between 10−10 and 10−8 mol L−1 and 30 s for concentrations between 10−7 and 10−4 mol L−1.
Effect of pH on the response of the electrodes
The influence of pH on the response of the EPMEs was determined by recording the EMF of the cell for solutions containing 10−6 mol L−1S-ibu at different pH values (pH, 1–12). These solutions were prepared by adding very small volume of HCl and/or NaOH solution (0.1 mol L−1 or 1.0 mol L−1 of each) to a S-ibu solution. The graphs of E(mV) versus pH (Fig. 3) indicate that the response of the electrodes does not depend on the pH value in the following pH range: 3.0–10.0 (I) and 4.0–11.0 (II), respectively.
![The influence of pH on the response of the enantioselective potentiometric membrane electrodes (S-ibuprofen = 10−6 mol L−1); for [5–6] fullerene-C70 based electrode (I) and diethyl (1,2-methanofullerene C70)-71-71-dicarboxylate based electrode (II).](/image/article/2010/AY/b9ay00086k/b9ay00086k-f3.gif) |
| Fig. 3 The influence of pH on the response of the enantioselective potentiometric membrane electrodes (S-ibuprofen = 10−6 mol L−1); for [5–6] fullerene-C70 based electrode (I) and diethyl (1,2-methanofullerene C70)-71-71-dicarboxylate based electrode (II). | |
The selectivity of the potentiometric membrane electrodes was checked by using mixed solutions method.25
The potential of the electrode is determined for the solution that contains both main and interfering ion, and its value is compared with the one recorded for the solution that contains only the main ion. The main ion must have the same activity in both solutions. The equation recommended to calculate the potentiometric selectivity coefficient is:
where Δ
E is the difference between the potential recorded for mixed solution (
Ei,j) and for the solution that contains only the main ion (
Ei), Δ
E =
Ei,j −
EI (all recorded in mV); S is the slope of the
electrode deducted from the equation of calibration (mV/decade of concentration);
ai is the activity of the main ion, i,
aj is the activity of interfering species, j;
zi is the charge of the main ion, i,
zj is the charge of interfering species, j.
Concentrations of interfering ions and S-ibu were in a ratio of 10
:
1. The potentiometric selectivity coefficients (KpotSel), (Table 2) show that paracetamol, polyvinylpyrolidone (PVP), creatine and creatinine do not interfere in the determination of S-ibu (KpotSel ≤ 10−3) and that the electrodes are enantioselective (since the value of KpotSel obtained over R-ibu was less than 10−4).
Table 2 Potentiometric selectivity coefficients of the electrodes used for assay of S-Ibuprofena
EPME based on |
KpotSel |
Interfering Species/J |
Paracetamol |
PVP |
Creatine |
Creatinine |
All measurements were made at room temperature; all values are the average of 10 determinations.
|
I |
3.4 × 10−3 |
1.1 × 10−3 |
1.2 × 10−3 |
1.0 × 10−3 |
II |
1.9 × 10−3 |
2.0 × 10−3 |
1.2 × 10−3 |
7.9 × 10−4 |
Analytical applications
A uniformity contents test was performed for Myprodol® capsules (1) and Nurofen® tablets (2) (Table 3). S-Ibuprofen can be reliably assayed in the capsules and tablets with average recoveries (n = 5) 99.25 ± 0.09% (1), 98.45 ± 0.16% (2) when the EPME based on (I) was used, and 99.39 ± 0.19% (1) and 98.47 ± 0.06% (2), respectively when the EPME based on (II) was used. Table 3 shows that these results were in good agreement for the two proposed electrodes as well as with those obtained using a HPLC method: 99.19 ± 0.81% S-ibuprofen in the Myprodol and 98.40 ± 0.80% S-ibuprofen in the Nurofen, but as can be seen the RSD values are higher for the HPLC method and the time spent for the analysis was higher due to the sampling necessary in order to perform the analysis.
Table 3 Recovery of S-Ibuprofen in Myprodol capsules (200mg ibuprofen/capsule) and Nurofen tablets (200mg ibuprofen/tablet)a
EPME based on |
mg, S-ibuprofen recovery |
Myprodol |
Nurofen |
All measurements were made at room temperature; all values are the average of 5 determinations.
|
I |
198.50 |
196.90 |
II |
198.78 |
196.94 |
Conclusions
This paper describes two enantioselective, potentiometric membrane electrodes designed using [5–6] fullerene-C70 and diethyl (1,2-methanofullerene C70)-71-71-dicarboxylate as chiral selectors for the enantioanalysis of S-ibuprofen. The enantioselectivity is good for both electrodes. The proposed EPMEs based on fullerene and its derivative have good feature in enantioselective analysis of pharmaceutical compounds. The simple, fast and reproducible construction of the electrodes assures the reliability of the analytical information. The good enantioselectivity of the designed electrodes makes the enantiopurity test of S-ibuprofen raw material as well as its direct enantioanalysis from Myprodol® capsules and Nurofen® tablets possible.
References
-
H. Y. Aboul-Enein and I. W. Wainer, The Impact of Stereochemistry on Drug Development and Use, Wiley, New York, 1997 Search PubMed.
-
A. J. Stone, The Theory of Intermolecular Forces, Clerendon Press, Oxford, 2002 Search PubMed.
- M. Simonyi, Med. Res. Rev., 1984, 4, 359 CrossRef CAS.
-
G. Subramanian, A Practical Approach to Chiral Separation by Liquid Chromatography, VCH, Weinheim, 1994 Search PubMed.
- V. Schurig, TrAC, Trends Anal. Chem., 2002, 21, 647 CrossRef CAS.
- D. Wistuba and V. Schurig, J. Chromatogr., A, 2000, 875, 255 CrossRef CAS.
-
R. I. Stefan, J. F. van Staden and H. Y. Aboul-Enein, Electrochemical Sensors in Bioanalysis, Marcel Dekker Inc., New York, 2001 Search PubMed.
- R. I. Stefan and R. M. Nejem, Instrum. Sci. Technol., 2004, 32, 311 CrossRef CAS.
- R. I. Stefan, J. F. van Staden and H. Y. Aboul-Enein, Chirality, 1999, 11, 631 CrossRef CAS.
- V. Horváth, T. Takács, G. Horvai, P. Huszthy, J. S. Bradshaw and R. M. Izatt, Anal. Lett., 1997, 30, 1591 CAS.
- A. D. Hulst and N. Verbeke, Enantiomer, 1997, 2, 69.
- A. A. Rat'ko, R. I. Stefan, J. F. van Staden and H. Y. Aboul-Enein, Sens. Actuators, B, 2004, 99, 539 CrossRef.
- D. E. Cliffel and A. J. Bard, J. Phys. Chem., 1994, 98, 8140 CrossRef CAS.
- J. Chlistunoff, D. Cliffel and A. J. Bard, Thin Solid Films, 1995, 257, 166 CrossRef CAS.
- S. Z. Kang, S. L. Xu, H. M. Zhang, L. B. Gan, C. Wang, L. J. Wan and C. L. Bai, Surf. Sci., 2003, 536, L408 CrossRef CAS.
- H. W. Kroto, J. R. Heath, S. C. O'Brien, R. F. Curl and R. E. Smalley, Nature, 1985, 318, 162 CrossRef CAS.
- H. Kato, A. Yashiro, A. Mizuno, Y. Nishida, K. Kobayashi and H. Shinohara, Bioorg. Med. Chem. Lett., 2001, 11, 2935 CrossRef CAS.
- T. Da Ros and M. Prato, Chem. Commun., 1999, 663 RSC.
- L. L. Dugan, D. M. Turetsky, C. Du, D. Lobner, M. Wheeler, C. R. Almli, C. K. F. Shen, T. Y. Luh, D. W. Choi and T. S. Lin, Proc. Natl. Acad. Sci. U. S. A., 1997, 94, 9434 CrossRef CAS.
- S. S. Adams, K. F. McCullough and J. S. Nicholson, Arch. Int. Pharmacodyn, 1969, 178, 115 Search PubMed.
- A. J. Hutt and J. Caldwell, J. Pharm. Pharmacol., 1983, 35, 693 CAS.
- H. B. Lee, T. E. Peart and M. L. Svoboda, J. Chromatogr., A, 2005, 1094, 122 CrossRef CAS.
- J. J. Nevado, J. R. Flores, G. C. Penalvo and R. M. R. Dorado, Anal. Bioanal. Chem., 2006, 384, 208 CAS.
- B. N. Nelson, L. J. Schieber, D. H. Barich, J. W. Lubach, T. J. Offerdahl, D. H. Lewis, J. P. Heinrich and E. J. Munson, Solid State Nucl. Magn. Reson., 2006, 29, 204 CrossRef CAS.
- K. Renk, Seletivity Problems of Membrane Ion-Selective Electrodes, Fresenius J. Anal. Chem., 1999, 365, 389 CrossRef CAS.
|
This journal is © The Royal Society of Chemistry 2010 |
Click here to see how this site uses Cookies. View our privacy policy here.