DOI:
10.1039/B806899B
(Paper)
CrystEngComm, 2009,
11, 122-129
Four 2D metal–organic networks incorporating Cd-cluster SUBs: hydrothermal synthesis, structures and photoluminescent properties†
Received
23rd April 2008
, Accepted 4th August 2008
First published on 8th October 2008
Abstract
Hydro(solvo)thermal reactions between 1,1′-biphenyl-2,2′,3,3′-tetracarboxyl acid (H4bptc) and Cd(NO3)2·4H2O at the presence of the ‘second’ ligands of bpydo, bpe, bpp and bix (bpydo = 4,4′-bipyridine-N,N′-dioxide; bpe = 1,2-bis-(4-pyridyl)ethane; bpp = 1,3-Bis-(4-pyridyl)propane; bix = 1,4-bis(imidazol-1-yl-methyl)benzene) yield four new 2D metal–organic coordination polymers [Cd9(bptc)4(μ3-OH)2(H2O)14]·(bpydo)·2H2O (2), [Cd2(bptc)(bpe)(H2O)2]·H2O (3), [Cd4(bptc)(Hbptc)(bpp)(μ3-OH)(H2O)4] (4), [Cd2(bptc)(bix)(H2O)] (5). Compound 2 presents a 2D grid structure with heptanuclear cadmium cluster and two kinds of discrete Cd atoms secondary building units (SUBs), 3 has a 2D structure with the novel Schläfli symbol of (4.62)(43.63)(44.610.8), 4 gives a 2D grid structure with a pentanuclear cadmium cluster, binuclear cadmium cluster and discrete Cd atom SUBs, and 5 has a 2D distorted CdI2-type topology structure with the Schläfli symbol of (43)2(46.66.83). The novel achiral crown-ether-like cycles in 2, 4 and 5 are found and occupied by the single Cd atom or binuclear cadmium cluster. All compounds give strong luminescent emission and have potential application as optical materials.
Introduction
The construction of metal–organic coordination polymers is of current interest in the field of supramolecular chemistry and crystal engineering, not only for their interesting molecular topologies and crystal packing motifs,1,2 but also for their potential applications as functional materials.3-6 The selection of the special ligand is very important in the construction of these coordination polymers. As an important family of multidentate O-donor ligands, organic aromatic polycarboxylate ligands have been extensively employed in the preparation of such metal–organic complexes with multidimensional networks and interesting properties.7 More importantly, the carboxylato groups in these ligands can form metal-carboxylato cluster SUBs to avoid interpenetrating and stabilize the resulting porous structures,2,3,7,8 give good magnetic9-11 and luminescent properties.12 Now, searching for a new kind of versatile polycarboxylate ligands deserves attention with regard to investigating new topologies and various functional materials.13-16 Following our previous studies,15,16 in this research we choose H4bptc (1,1′-biphenyl-2,2′,3,3′-tetracarboxyl acid, Scheme 1) as the organic ligand, and the considerations are: (1) being an axial chiral ligand, it may allow the induction of a chiral unit when coordinating with metal atoms; (2) H4bptc multiple bridging moieties, a variety of connection modes with metal centers, are possible and provide abundant structural motifs; (3) four adjacent “dense” tetracarboxyl acid have a high potential to form a novel metal-cluster when reacting with metal atoms; (4) only one coordination polymer constructed from the H4bptc ligand has been reported,16 and much work is still necessary to understand the coordination chemistry of H4bptc.
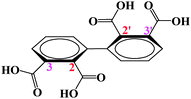 |
| Scheme 1 The structure of H4bptc. | |
Recently, a novel host–guest metal–organic compound [Cd4(bptc)2(bpy)(H2O)8]·5.5H2O (1) with both chiral hydrophilic and achiral hydrophobic channels has been obtained by us through the reaction of Cd2+ ion and H4bptc based on hydro(solvo)thermal reactions.16 With the aim of further understanding the coordination chemistry of this new ligand and preparing new materials with interesting structural topologies and excellent physical properties, we have recently engaged in the research of coordination polymers based on it.
Experimental
H4bptc and bix were synthesized according to the literature.16,17 Other starting materials were of reagent quality and obtained from commercial sources without further purification.
[Cd9(bptc)4(µ3-OH)2(H2O)14]·(bpydo)·2H2O (2)
2 was synthesized hydrothermally in a 23 ml Teflon-lined autoclave by heating a mixture of 0.1 mmol 2,2′,3,3′-H4bptc, 0.1 mmol bpydo, 0.2 mmol Cd(NO3)2·4H2O and one drop of Et3N (pH ∼ 8) in 8 ml water and 2 mL acetonitrile at 120 °C for 3 d. Colorless diamond-like single crystals were collected in 75% yield based on Cd(NO3)2·4H2O. Anal. found (calcd) for C74H66Cd9N2O52: C, 31.51 (31.44); H, 2.75 (2.72); N, 1.05 (0.99); IR (KBr, cm−1): 3423(w, br), 3113(w), 1552(vs), 1453(s), 1392(vs), 1238(m), 1113(m), 1090(m), 942(m), 854(s), 777(s), 715(s), 660(s).
[Cd2(bptc)(bpe)(H2O)2]·H2O (3)
3 can also be obtained following the same synthetic procedures as that of 2 except for using bpe instead of bpydo as the starting material. Colorless rectangle single crystals were collected in 83% yield based on Cd(NO3)2·4H2O. Anal. found (calcd) for C28H24Cd2N2O11: C, 42.77 (42.61); H, 3.14 (3.06); N, 3.67 (3.55)%; IR (KBr, cm−1): 3421(s, br), 1612(s), 1546(vs), 1455(s), 1430(s), 1382(vs), 1210(m), 1063(m), 1026(m), 854(m), 823(w), 779(m), 760(m), 713(m), 660(m), 549(w).
[Cd4(bptc)(Hbptc)(bpp)(μ3-OH)(H2O)4] (4)
4 can also be obtained following the same synthetic procedures as that of 2 except using bpp instead of bpydo. Colorless plate single crystals were collected in 77% yield based on Cd(NO3)2·4H2O. Anal. found (calcd) for C45H35Cd4N2O21: C, 38.79 (38.90); H, 2.72 (2.54); N, 1.97 (2.02)%; IR (KBr, cm−1): 3424(s, br), 1708(vs), 1604(s), 1562(vs), 1458(s), 1380(vs), 1051(m), 835(m), 764(m), 707(m), 656(m).
[Cd2(bptc)(bix)(H2O)] (5)
5 can also be obtained following the same synthetic procedures as that of 2 except using bix instead of bpydo. Colorless needle single crystals were collected in 67% yield based on Cd(NO3)2·4H2O. Anal. found (calcd) for C30H22Cd2N4O9: C, 44.69 (44.63); H, 2.82 (2.75); N, 6.97 (6.94) %; IR (KBr, cm−1): 3422(s, br), 3113 (vs), 1551(s), 1453(vs), 1392(s), 1238(vs), 1090(vs), 853(vs), 777(vs), 715(vs), 659(vs).
Physical measurements
Elemental analyses were performed on a Perkin-Elmer 240C elemental analyzer. The IR spectra were obtained as KBr disks on a VECTOR 22 spectrometer. Thermal analyses were performed on a TGA V5.1A Dupont 2100 instrument from room temperature to 700 °C with a heating rate of 10 °C min−1 in air. Luminescence spectra for the solid samples were recorded on a Hitachi 850 fluorescence spectrophotometer.
X-Ray crystallographic studies
Single crystals for 2, 3, 4 and 5 were used for structural determinations on a Bruker SMART APEX CCD diffractometer using graphite-monochromated Mo Kα radiation (λ = 0.71073 Å) at room temperature using the ω-scan technique. Lorentz polarization and absorption corrections were applied. The structures were solved by direct methods and refined with the full-matrix least-squares technique using the SHELXS-97 and SHELXL-97 programs.18 Anisotropic thermal parameters were assigned to all non-hydrogen atoms. Analytical expressions of neutral-atom scattering factors were employed, and anomalous dispersion corrections were incorporated. The crystallographic data and selected bond lengths for 2, 3, 4 and 5 are listed in Tables 1 and 2. CCDC reference numbers: 685455 (2); 685456(3); 685457(4); 685458(5).†
Compound |
2
|
3
|
4
|
5
|
R1 = ∑||Fo| − |Fc||/|Fo|. wR2 = [∑w(∑Fo2 − Fc2)2/∑w(Fo2)2]1/2.
|
Empirical formula |
C74H66Cd9N2O52 |
C28H24Cd2N2O11 |
C45H35Cd4N2O21 |
C30H22Cd2N4O9 |
FW
|
2826.89 |
789.29 |
1389.35 |
807.34 |
Crystal system |
triclinic |
triclinic |
triclinic |
triclinic |
Space group |
P-1
|
P-1
|
P-1
|
P-1
|
a/Å |
10.642(2) |
10.2134(4) |
10.1503(10) |
10.0622(6) |
b/Å |
12.354(2) |
11.7040(5) |
15.0272(15) |
11.1733(7) |
c/Å |
16.323(3) |
12.3421(5) |
16.7597(17) |
13.2450(8) |
α/° |
79.113(4) |
79.7200(10) |
66.575(2) |
89.4170(10) |
β/° |
79.926(4) |
79.2680(10) |
77.358(2) |
75.1850(10) |
γ/° |
83.023(4) |
72.6080(10) |
81.220(1) |
76.9370(10) |
V/Å3 |
2066.2(6) |
1371.36(10) |
2282.6(4) |
1400.56(15) |
Z
|
1 |
2 |
2 |
2 |
R
int
|
0.0212 |
0.0320 |
0.0322 |
0.0501 |
ρ/g cm−3 |
2.272 |
1.911 |
2.021 |
1.905 |
F(000)
|
1372 |
780 |
1358 |
796 |
Goodness of fit on F2 |
1.108 |
1.140 |
1.073 |
0.934 |
R1, wR2a [I > 2σ(I)] |
0.0535, 0.1036 |
0.0479, 0.1160 |
0.0584, 0.1297 |
0.0358, 0.0594 |
R1, wR2a [all data] |
0.0805, 0.1084 |
0.0584, 0.1192 |
0.0837, 0.1350 |
0.0572, 0.0643 |
Table 2 Selected bond lengths [Å] for 2–5
Symmetry codes: #1 −x + 1, −y + 1, −z + 1; #2 −x, −y +1, −z + 1; #3 x + 1, y, z; #4 −x + 1, −y + 2, −z; #5 −x, −y + 2, −z.
Symmetry codes: #1 x −1, y, z; #2 −x + 2, −y, −z + 1; #3 x, y − 1, z + 1.
Symmetry codes: #1 −x + 1, −y, −z + 1; #2 −x, −y, −z + 1; #3 x − 1, y, z; #4 −x + 1, −y, −z + 2.
Symmetry codes: #1 −x, −y + 1, −z; #2 −x + 1, −y + 1, −z; #3 x − 1, y, z; #4 −x + 1, −y, −z + 1.
|
Compound 2a |
Cd(1)–O(18) |
2.224(5) |
Cd(1)–O(3) |
2.226(5) |
Cd(1)–O(12#1) |
2.253(5) |
Cd(1)–O(20) |
2.249(5) |
Cd(1)–O(14#2) |
2.262(4) |
Cd(1)–O(19) |
2.456(5) |
Cd(2)–O(18) |
2.163(5) |
Cd(2)–O(4) |
2.257(4) |
Cd(2)–O(10) |
2.275(5) |
Cd(2)–O(16#3) |
2.307(5) |
Cd(2)–O(7#5) |
2.319(5) |
Cd(2)–O(8#4) |
2.553(5) |
Cd(3)–O(18#1) |
2.243(5) |
Cd(3)–O(15#2) |
2.320(4) |
Cd(3)–O(11) |
2.339(5) |
Cd(4)–O(5#4) |
2.250(5) |
Cd(4)–O(21) |
2.265(5) |
Cd(4)–O(2#4) |
2.307(5) |
Cd(5)–O(22) |
2.273(5) |
Cd(5)–O(23) |
2.290(5) |
Cd(5)–O(24) |
2.301(5) |
Cd(5)–O(6) |
2.301(4) |
Cd(5)–O(16#5) |
2.307(5) |
Cd(5)–O(7) |
2.390(5) |
Cd(6)–O(25#2) |
2.229(5) |
Cd(6)–O(9#2) |
2.261(5) |
Cd(6)–O(13#2) |
2.350(5) |
|
|
compound 3b |
Cd(1)-O(7#1)
|
2.198(4) |
Cd(1)–O(8#2) |
2.305(4) |
Cd(1)-N(1#3)
|
2.313(4) |
Cd(1)–O(9) |
2.330(4) |
Cd(1)-O(2)
|
2.371(4) |
Cd(1)–O(1) |
2.438(4) |
compound 4c |
Cd(1)–O(5) |
2.232(5) |
Cd(1)–O(1#1) |
2.254(5) |
Cd(1)–O(17#1) |
2.330(6) |
Cd(2)–O(21#2) |
2.176(5) |
Cd(2)–O(3#3) |
2.306(6) |
Cd(2)–O(7#2) |
2.408(5) |
Cd(3)–O(21) |
2.229(5) |
Cd(3)–O(4#3) |
2.229(5) |
Cd(3)–O(6) |
2.256(5) |
Cd(3)–N(1) |
2.304(6) |
Cd(3)–O(11) |
2.395(5) |
Cd(3)–O(9) |
2.566(5) |
Cd(4)–O(12) |
2.245(5) |
Cd(4)–O(13) |
2.258(5) |
Cd(4)–O(19) |
2.257(6) |
Cd(4)–O(20) |
2.304(5) |
Cd(4)–O(18) |
2.342(5) |
Cd(4)–O(13#4) |
2.422(5) |
Cd(5)–O(8#2) |
2.167(5) |
Cd(5)–O(21) |
2.164(5) |
Cd(5)–O(2#1) |
2.172(5) |
Cd(5)–O(10) |
2.256(5) |
Compound 5d |
Cd(1)–O(1) |
2.248(3) |
Cd(1)–O(8#3) |
2.264(3) |
Cd(1)–O(3#1) |
2.359(3) |
Cd(1)–O(4#1) |
2.394(4) |
Cd(1)–O(7#3) |
2.620(4) |
Cd(1)–C(14#1) |
2.724(5) |
Cd(2)–O(2) |
2.205(3) |
Cd(2)–N(2#4) |
2.224(4) |
Cd(2)–O(9) |
2.314(3) |
Cd(2)–O(5#2) |
2.326(3) |
Cd(2)–O(5) |
2.350(3) |
Cd(2)–O(6#2) |
2.465(3) |
Results and discussion
Syntheses
The successful isolation of 1 in our previous work prompted us to extend our study to other complexes based on the 2,2′,3,3′-H4bptc. Recently, the ‘mix-ligand’ method in the design of high dimensional MOFs has aroused chemists' attention.19 In this method, the selection of a second ligand is important in the construction of the resulting architecture. The common ‘second’ ligands are two kinds: rigid or flexible. In our investigation of the coordination chemistry of 2,2′,3,3′-H4bptc, we have selected two rigid ‘second’ ligands of bpy and bpydo and three flexible ligands of bpe, bpp and bix.
Crystal structures
[Cd9(bptc)4(μ3-OH)2(H2O)14]·(bpydo)·2H2O (2).
The asymmetric unit of 2 contains six kinds of crystallographically non-equivalent Cd atoms (Cd1, Cd2, Cd3, Cd4, Cd5, and Cd6), two unique bptc4− ligands in different coordination modes (Fig. 1 and Scheme 2), one three-coordinate hydroxy group (μ3-OH), one half of bpydo (the bpydo molecule lies about an inversion center), seven coordinated and one solvated water molecules (Fig. 1 and Scheme 2). Three Cd atoms (Cd3, Cd4 and Cd6) lie on inversion centers. The coordination geometries for the six-coordinate Cd1, Cd2, Cd3, Cd4, Cd5 and Cd6 atoms are all close to that of an octahedron with Cd–O bands ranging from 2.186 to 2.561Å.
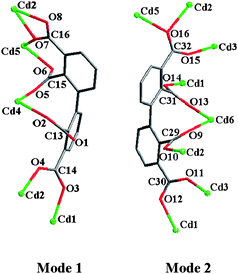 |
| Scheme 2 Two different coordination modes of the bptc4− ligand in 2. Each of the two modes is actually chiral and has its mirror coexisting in the crystal. | |
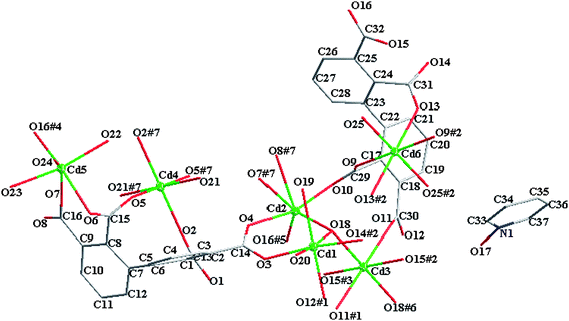 |
| Fig. 1 The molecular structure of 2. Solvent water molecules and hydrogen atoms are omitted for clarity. | |
As to 2,2′,3,3′-bptc4− in mode 1, the dihedral angle between two phenyl rings is 68.2°, four carboxylate groups (2-, 2′-, 3- and 3′-COO−) have a dihedral angle of 67.9, 69.9, 35.4 and 25.3° towards the plane of the corresponding linking phenyl rings, respectively. Compared with mode 1, the dihedral angle between two phenyl rings of bptc4− is 69.2°, four carboxylate groups (2-, 2′-, 3- and 3′-COO−) have a dihedral angle of 68.7, 72.7, 45.9 and 15.7° towards the plane of the corresponding linking phenyl rings in mode 2, respectively.
Compound 2 presents a 2D layer structure along (011) plane (Fig. 2a), in which the bpydo molecules don't coordinate to any Cd atom and exist between the layers acting as guest molecules and templates (Fig. S1).† In each layer, Cd atoms are connected together through carboxylate groups of the ligand bptc4− in two coordination modes and µ3-O18. Cd1, Cd2, Cd3 and Cd5 atoms are united together through four carboxylate groups (C14, C16, C30 and C32) and µ3-O18 to present a central symmetrical heptanuclear cadmium cluster (Fig. 2c), the symmetrical center is Cd3 atom. These heptanuclear cadmium clusters are united together through ligands in modes 1 and 2 along the c and a axis to give a 2D metal–organic network. More interestingly, Cd4 and Cd6 atoms can be locked in their cavities built from 2,2′,3,3′-bptc4− ligands (mode 1 and mode 2, respectively) and heptanuclear clusters (Fig. 3). The cavities can be regarded as crown-ether-like cycles with four donor oxygen atoms (two pairs of symmetry related O2 and O5 atoms in cavity A and two pairs of symmetry related O9 and O13 atoms in cavity B), which can lock the Cd atom (Cd4 or Cd6) in their cavities. It should be noted that each cavity is achiral, now that it includes two ligands with opposite chirality (P and M). In addition, a 48 metal–organic cycle built from four heptanuclear clusters, four discrete Cd atoms and organic ligands in mode 1 and mode 2 is found in compound 2. If we take heptanuclear clusters and discrete Cd4 and Cd6 atoms as SUBs, this complicated 2D structure can be regarded as a simple (4,4) grid structure (Fig. S2).† Such layers are united together through hydrogen bonds (Table S1)† to give a 3D supramolecular structure.
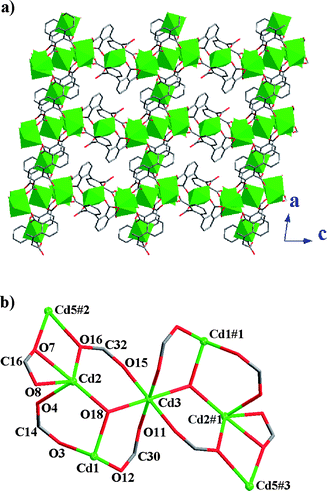 |
| Fig. 2 (a) 2D metal–organic network in 2. Cd atoms are drawn as polyhedrons. (b) The heptanuclear cadmium cluster. | |
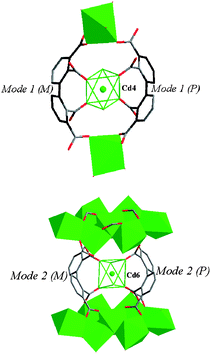 |
| Fig. 3 View of the crown-ether-like cycles (A: top and B: bottom) built from bptc4− ligands (mode 1 and mode 2, respectively) and Cd atoms in compound 2. The cavities (A and B) are occupied by Cd4 and Cd6 atoms, respectively. | |
[Cd2(bptc)(bpe)(H2O)2]·H2O (3).
The asymmetric unit of 3 contains two crystallographically non-equivalent Cd atoms (Cd1 and Cd2), one 2,2′,3,3′-bptc4−, one bpe ligand, two coordinated and one solvated water molecules (Fig. 4). The coordination geometries for Cd1 and Cd2 atoms are all close to that of distorted octahedron with bands ranging from 2.198 to 2.509 Å. As to 3,3′-bptc4− in 3, the dihedral angle between two phenyl rings is 52.9°, four carboxylate groups (2-, 2′-, 3-, and 3′-COO−) have a dihedral angle of 60.0, 78.1, 56.0 and 7.0° towards the plane of corresponded linking phenyl rings, respectively.
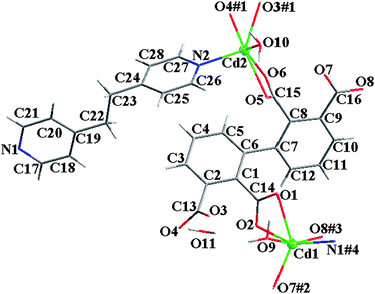 |
| Fig. 4 The molecular structure of 3 (50% thermal ellipsoids). Labels for all hydrogen atoms are omitted for clarity. | |
In compound 3, Cd1 and Cd2 atoms are first connected together through 2,2′,3,3′-bptc4− to give a 1D ribbon (Fig. 5a), in which two symmetry related Cd1 atoms are connected together through two syn-bridged carboxylate groups (2 and 3′-COO−) from two adjacent bptc4− ligands to give a binuclear cluster. Such binuclear cadmium clusters are arranged in the middle of the ribbon, whereas Cd2 atoms are suspended along the edge of the ribbon through coordination to 3- and 2′-COO−groups from two adjacent bptc4− ligands. Such ribbons are further united together through bpe to present a 2D double layer structure (Fig. 5b and Fig. S3†). Considering the coordination modes of bptc4−, which is linked four times to the metal center (four discrete cadmium atoms and one binuclear cadmium cluster), it can be viewed as a four-connected node. Cd2 atom and binuclear cadmium cluster can be viewed as three- and four-connected nodes, respectively. Taking Cd2 atom and binuclear cadmium cluster as nodes, bptc4− as rods, the topology representation for 3 is illustrated in Fig. 5b. The net of this framework can be described with the Schläfli symbol of (4.62)(43.63)(44.610.8). As far as we know, this topology is completely new within coordination polymer chemistry. The discovery of this new topology is useful at the basic level in the crystal engineering of coordination networks. In the bpe molecular structure, two pyridine rings arrange at two sides of the C22–C23 single bond and are nearly perpendicular to each other, with a dihedral angle of 88.4°. These 2D layers are further united together through hydrogen bonds (Table S1†) to give a 3D supramolecular structure.
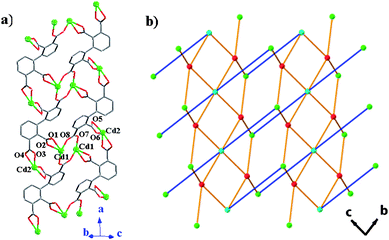 |
| Fig. 5 (a) 1D Cd-bptc ribbon structure of 3. (b) Schematic diagram of the extended net with the Schläfli symbol of (4.62)(43.63)(44.610.8): bptc ligand (red), binuclear cadmium cluster (turquoise), Cd2 atom (green) and bpe ligand (blue). | |
[Cd4(bptc)(Hbptc)(bpp)(μ3-OH)(H2O)4] (4).
The asymmetric unit of 4 contains five kinds of crystallographically non-equivalent Cd atoms (Cd1, Cd2, Cd3, Cd4 and Cd5), one 2,2′,3,3′-bptc4−, one 2,2′,3,3′-Hbptc3−, one hydroxy group(µ3-OH, O21), one bpp and four coordinated water molecules (Fig. 6). Two of the five Cd atoms (Cd1 and Cd2) lie on inversion centers. The coordination geometries for the six-coordinate Cd1, Cd2, Cd3, Cd4 and Cd5 atoms are all close to octahedron with bands ranging from 2.169 to 2.838 Å. As to 2,2′,3,3′-bptc4−, the dihedral angle between two phenyl rings is 78.9°, four carboxylate groups (2-, 2′-, 3- and 3′-COO−) have a dihedral angle of 82.1, 83.2, 17.8 and 13.6° towards the plane of the corresponding linking phenyl rings, respectively. The dihedral angle between two phenyl rings is 64.1°, four carboxylate groups (2-, 2′-, 3-COO− and 3′-COOH) have a dihedral angle of 68.3, 66.4, 46.9 and 49.1° towards the plane of the corresponding linking phenyl rings in 2,2′,3,3′-Hbptc3−, respectively.
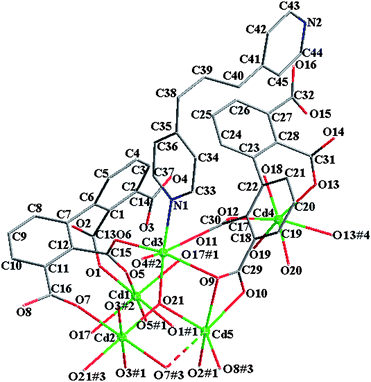 |
| Fig. 6 The molecular structure of 4. Solvent water molecules and hydrogen atoms are omitted for clarity. | |
Compound 4 presents a 2D grid structure in the ac plane (Fig. 7). Cd2, Cd3 and Cd5 atoms are united together through six symmetry related carboxylate groups (C14, C16 and C29) and two µ3-O21 to give a central symmetrical pentanuclear cadmium cluster with a symmetry center of Cd2 atom. Two symmetry related Cd4 atoms are connected together through two oxygen atoms (O13) from two symmetry related carboxylate groups (C31) to give a binuclear cadmium cluster, symmetry code is 1 − x, −y, 2 − z. It should be noted that two pentanuclear cadmium clusters can be connected through two 2,2′,3,3′-bptc4− ligands to give a crown-ether-like cycle with four donor oxygen atoms (two pairs of symmetry related O1 and O5 atoms), which can lock the Cd1 atom in the cavity (Fig. 7). In addition, a 70 metal–organic cycle built from four pentanuclear cadmium clusters, two binuclear cadmium clusters, two discrete Cd atoms and organic ligands are found in compound 4. Its stability may be related to the formation of cadmium clusters.
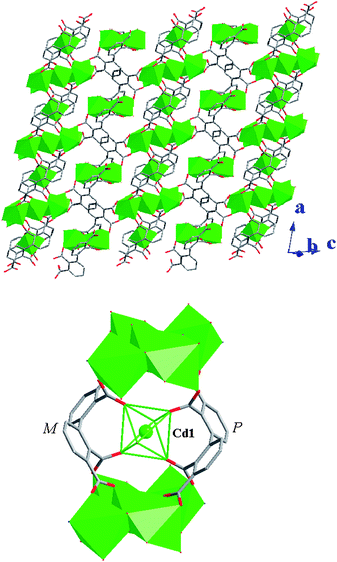 |
| Fig. 7 Top: 2D metal–organic layer structure in 4. Cd atoms are drawn as polyhedrons. Bottom: view of the crown-ether-like cycle built from two 2,2′,3,3′-bptc4− ligands and two pentanuclear cadmium clusters. Its cavity is occupied by the Cd1 atom. The cavity is achiral, now that it includes two opposite-chirality ligands (P and M). | |
Bpp adopts a mono-coordinate mode to the Cd3 atom and the other pyridine ring is distributed between two layers (Fig. 8). N2 and O16 from the carboxylate group (O15–O16) atoms share one hydrogen atom (H2) and are linked together through the formation of a strong hydrogen bond (N2⋯H2⋯O16) (Table S1).† In the bpp molecular structure, two pyridine rings have a dihedral angle of 109.5 and 99.5° towards the C38–C39–C40 plane, respectively. The two pyridine rings give a dihedral angle of 97.1° towards each other. Through the hydrogen bonds (Table S1),† compound 4 gives a 3D supramolecular structure (Fig. 8).
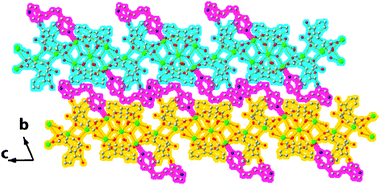 |
| Fig. 8 The 3D supramolecular structure of 4. Two adjacent 2D metal–organic frameworks are marked as blue and yellow, whereas the mono-coordinate “second” ligands (bpp) are highlighted as sanguine. | |
[Cd2(bptc)(bix)(H2O)] (5).
The asymmetric unit of 5 contains two crystallographically non-equivalent Cd atoms (Cd1 and Cd2), one 2,2′,3,3′-bptc4−, one bix ligand, one coordinated water molecule (Fig. 9). The coordination geometries for the six-coordinate Cd1 and Cd2 atoms are all close to that of a distorted octahedron with bands ranging from 2.205 to 2.620 Å. As for 2,2′,3,3′-bptc4− in 3, the dihedral angle between the two phenyl rings is 82.7°, four carboxylate groups (2-, 2′-, 3- and 3′-COO−) have a dihedral angle 97.0, 61.8, 15.4 and 37.8° towards the plane of the corresponding linking phenyl rings, respectively.
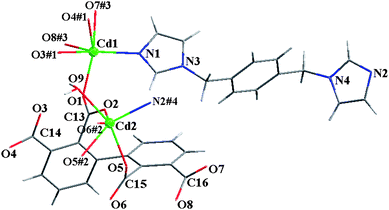 |
| Fig. 9 The molecular structure of 5. | |
In 5, Cd1 and Cd2 atoms are first connected together through 2,2′,3,3′-bptc4− to give a 1D chain (Fig. 10a). Two symmetry related bptc4− with opposite chirality (P and M) are coordinated to two Cd1 atoms through 3-, and 3′-COO−groups to form a crown-ether-like cycle with six oxygen atoms (one bichelating 2′- and monochelating 2-COO−groups), in which two symmetry related Cd2 atoms are fixed. Such crown-ether-like cycles are connected together through 2-COO−groups in an anti–anti conformation and Cd1, Cd2 atoms give a 1D diamond-like chain along the a axis (Fig. 10a). Bix takes an anti-conformation and coordinates to such chains to give a 2D metal–organic network.
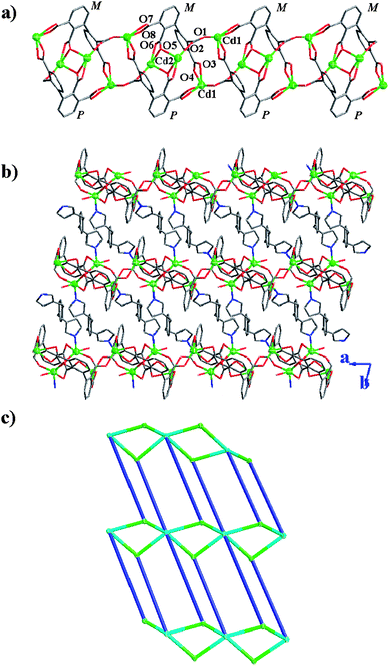 |
| Fig. 10 (a) View of the diamond-like Cd-bptc chain structure along the a axis in 5. Cd2-cluster is locked in the cavity built from Cd1 and the ligand bptc4− with opposite chirality. (b) 2D layer structure along the bc plane. (c) View of the distorted CdI2-type topology for 5. Cd1 and the binuclear cadmium cluster are drawn as green and turquoise, whereas the bix are highlighted as blue. | |
From a topology view, the Cd1 atom can be viewed as a 3-connected node and the binuclear cadmium cluster acts as the 6-connected nodes. In this way this framework can be simplified to be a (3,6) -connected network with a distorted CdI2 topology (Fig. 10) having the Schläfli symbol of (43)2(46.66.83). Although CdI2-type structures are quite commonly found in inorganic compounds, only a few examples are known in organic–inorganic hybrid materials.20 In the molecular structure of bix, two imidazole rings have a dihedral angle of 93.4 and 100.7° towards the benzene ring plane, respectively. Two imidazole rings give a dihedral angle of 30.1°.
According to the above structural description of our example compounds and compound 1, 2,2′,3,3′-Hbptc3− and 2,2′,3,3′-bptc4− ligands exhibit eight distinct kinds of bridging modes as shown in Schemes 2 and 3. Two kinds of coordination modes (µ6:η2η2η2η1 and µ4:η2η2η2η2) of 2,2′,3,3′-bptc4− in compound 1 are shown in Schemes 3a and 3b. In complex 2, two unique bptc4− ligands in different coordination modes (µ5:η2η1η2η2 in mode I and µ8:η3η2η2η2 in mode II) are found (Scheme 2). In compound 3, 2,2′,3,3′-bptc4− ligand takes a trans-conformation with four carboxylate groups to coordinate to five Cd atoms with µ5:η1η1η1η2 coordination mode (Scheme 3c). In compound 4, 2,2′,3,3′-bptc4− ligand takes a trans-conformation with four carboxylate groups to coordinate to seven Cd atoms with µ7:η2η2η2η2 coordination mode (Scheme 3d). The 3- and 3′-COO−groups take a syn–syn conformation, whereas the 2- and 2′-COO−groups take a syn–trans conformation. In comparison with 2,2′,3,3′-bptc4−, 2,2′,3,3′-Hbptc4− takes a trans-conformation with three carboxylate groups to coordinate to four Cd atoms with µ4:η2η2η2η0 coordination mode (Scheme 3e). The 3′-COOH group remains protonized and withholds from coordinating to any Cd atoms. In compound 5, 2,2′,3,3′-bptc4− ligand takes a trans-conformation with four carboxylate groups to coordinate to five Cd atoms with µ5:η2η2η1η1 coordination mode (Scheme 3f). Though each of the coordination modes for 2,2′,3,3′-bptc4− is actually chiral and has its mirror coexisting in the crystals in this paper, chiral metal–organic frameworks are expected when single chirality crystallizes in a single crystal.
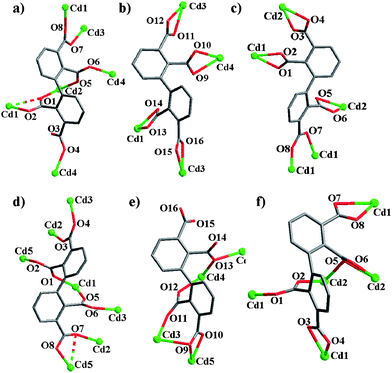 |
| Scheme 3 Coordination modes of the bptc4− (a, b, c, d and f) and Hbptc3− (e) ligands. a and b in 1; c in 3; d and e in 4; f in 5. Other two kinds of coordination modes of the bptc4− in 2 can be found in Scheme 2. | |
Thermal analyses and luminescent properties
For 2, the weight loss of 10.15% (calcd. 10.20%) below 300 °C corresponds to the loss of one solvent and seven coordinated water molecules per formula. For 3, the weight loss of 7.00% (calcd. 6.85%) below 133 °C is attributed to the release of one solvent and two coordinated water molecules per formula. For 4, the weight loss of 5.02% (calcd. 5.19%) below 245 °C is attributed to the release of four coordinated water molecules per formula. For 5, the weight loss of 2.01% (calcd. 2.24%) below 198 °C is attributed to the release of one coordinated water molecule per formula (Fig. S4–S7 show the TG curves for 2–5).†
The photoluminescence properties of the compounds were investigated. While the free H4bptc ligand displays oppositely weak luminescence (λ = 390 nm) in the solid state at room temperature, the compounds exhibit distinct radiation emission maxima (λ = 377 nm for 2, 440 nm for 3, 446 nm for 4 and 430 nm for 5) upon excitation at λ = 340 nm for 2 and 3 and 33 5nm for 4 and 5, (Fig. S8†). The emission bands in the four compounds might be attributed to the intra-ligand emission from H4bptc. The enhancement of luminescence may be attributed to ligand chelation to the metal center, which effectively increases the rigidity of the ligand and reduces the loss of energy by radiationless decay.12 Baseochromic shifts of emission occur in 2, 3, 4 and 5 which are probably due to the differences of the coordination environment around the Cd ions since photoluminescence behavior is closely associated with the metal ions and the ligands coordinated around them.21 In addition, the oppositely strong luminescence of 2 and 4 may be ascribed to the presence of high-nucleus metal clusters, since the µ3-OH ligand may tighten the whole skeleton, resulting in much weaker vibrations.22
Conclusion
In this paper, a new multicarboxylate ligand has been introduced to construct new MOFs based on Cd-cluster SUBs. Though some metal–organic frameworks based on a uniform metal-cluster have been found, one framework with two different kinds of clusters is very rare. In compound 4, a binuclear cadmium cluster, a pentanuclear cadmium cluster and a discrete Cd atom concomitant in one structure is amazing, and this kind of ‘mix’ systems may yield new interesting topologies and physical properties. The crown-ether-like cycles in 2, 4 and 5 are of interest in supramolecular chemistry. The topology of 3 and 5 represents the rare reported 2D MOFs with novel Schläfli symbols of (4.62)(43.63)(44.610.8) and (43)2(46.66.83). These results demonstrated that H4bptc could serve as a flexible ligand to construct novel supramolecular architectures.
Acknowledgements
This work was financially supported by the National Natural Science Foundation of China (NSFC: 20771056), the National Basic Research Program of China (2007CB925102), National Nature Science Foundation of China (Grant No. 20490218), Jiangsu Science and Technology Department and the Center of Analysis and Determining of Nanjing University.
References
-
(a)
A. F. Wells, Three-Dimensional Nets and Polyhedra, Wiley-Interscience, New York, 1977 Search PubMed;
(b) S. R. Batten and R. Robson, Angew. Chem., Int. Ed., 1998, 37 Search PubMed;
(c) R. Robson, J. Chem. Soc., Dalton Trans., 2000, 3735 RSC;
(d) B. Moulton and M. J. Zaworoko, Chem. Rev., 2001, 101, 1629 CrossRef CAS;
(e) L. Carlucci, G. Ciani and D. M. Proserpio, Coord. Chem. Rev., 2003, 246, 247 CrossRef CAS;
(f) V. A. Blatov, L. Carlucci, G. Cianib and D. M. Proserpio, CrystEngComm, 2004, 6, 377 RSC;
(g) A. Y. Robin and K. M. Fromm, Coord. Chem. Rev., 2006, 250, 2127 CrossRef CAS.
-
(a) N. W. Ockwig, O. Delgado-Friedrichs, M. O'Keefee and O. M. Yaghi, Acc. Chem. Res., 2005, 38, 176 CrossRef CAS;
(b) D. Bradshaw, J. B. Claridge, E. J. Cussen, T. J. Prior and M. J. Rosseinsky, Acc. Chem. Res., 2005, 38, 273 CrossRef CAS;
(c) J.-H. Chou, M. E. Kosal, S. Nakagaki, D. W. Smithenry and S. R. Wilson, Acc. Chem. Res., 2005, 38, 283 CrossRef CAS;
(d) R. J. Hill, D. L. Long, N. R. Champness, P. Hubberstey and M. Schroöder, Acc. Chem. Res., 2005, 38, 377;
(e) L. Perez-Garcia and D. B. Amabilino, Chem. Soc. Rev., 2007, 36, 941 RSC;
(f) C. He, Y.-G. Zhao, D. Guo, Z.-H. Lin and C.-Y. Duan, Eur. J. Inorg. Chem., 2007, 3451 CrossRef CAS.
-
(a) M. Eddaoudi, D. B. Moler, H. L. Li, B. L. Chen, T. M. Reineke, M. O'Keeffe and O. M. Yaghi, Acc. Chem. Res., 2001, 34, 319 CrossRef CAS;
(b) S. Kitagawa, R. Kitaura and S. Noro, Angew. Chem., Int. Ed., 2004, 43, 2334 CrossRef CAS;
(c) G. Férey, C. Mellot-Draznieks, C. Serre and F. Millange, Acc. Chem. Res., 2005, 38, 217 CrossRef CAS;
(d) X.-C. Huang, Y.-Y. Lin, J.-P. Zhang and X.-M. Chen, Angew. Chem., Int. Ed., 2006, 45 Search PubMed;
(e) M. Higuchi, S. Horike and S. Kitagawa, Supramol. Chem., 2007, 19, 75 CrossRef CAS;
(f) Y.-L. Liu, A. J. Cairns, J. Eckert, V. C. Kravtsov, R. Luebke and M. Eddaoudi, Angew. Chem., Int. Ed., 2007, 46 Search PubMed.
-
(a) S. L. James, Chem. Soc. Rev., 2003, 32, 276 RSC;
(b) D. Maspoch, D. Ruiz-Molina and J. Veciana, J. Mater. Chem., 2004, 2713 RSC.
-
(a) O. Evans, R.-G. Xiong, Z. Wang, G.-K. Wong and W. Lin, Angew. Chem., Int. Ed., 1999, 38 Search PubMed;
(b) O. R. Evans and W. Lin, Acc. Chem. Res., 2002, 35, 511 CrossRef CAS;
(c) J. F. Eubank, V. C. Kravtsov and M. Eddaoudi, J. Am. Chem. Soc., 2007, 129, 5820 CrossRef CAS.
-
(a) B. F. Abrahams, P. A. Jackson and R. Roboson, Angew. Chem., Int. Ed., 1998, 37, 2656 CrossRef CAS;
(b) B. F. Abrahams, M. Moylan, S. D. Orchard and R. Robson, Angew. Chem., Int. Ed., 2003, 42, 1848 CrossRef CAS;
(c) Q. Ye, X.-S. Wang, H. Zhao and R.-G. Xiong, Chem. Soc. Rev., 2005, 34, 208 RSC.
-
(a) H. Li, M. Eddaoudi, M. O'Keeffe and O. M. Yaghi, Nature, 1999, 402, 276 CrossRef CAS;
(b) S. S.-Y. Chui, S. M.-F. Lo, J. P. H. Charmant, A. G. Orpen and I. D. Williams, Science, 1999, 283, 1148 CrossRef CAS;
(c) M. Eddaoudi, J. Kim, N. Rosi, D. Vodak, J. Wachter, M. O'Keefe and O. M. Yaghi, Science, 2002, 295, 469 CrossRef;
(d) M. Eddaoudi and J. Kim, Nature, 2003, 423, 705 CrossRef CAS;
(e) H. K. Chae, D. Y. Siberio-Pėrez, J. Kim, Y. B. Go, M. Eddaoudi, A. J. Matzger, M. O'Keeffe and O. M. Yaghi, Nature, 2004, 427, 523 CrossRef CAS;
(f) B.-H. Ye, M.-L. Tong and X.-M. Chen, Coord. Chem. Rev., 2005, 249, 545 CrossRef CAS.
-
(a) J. S. Seo, D. Whang, H. Lee, S. I. Jun, J. Oh, Y. J. Jeon and K. Kim, Nature, 2000, 404, 982 CrossRef CAS;
(b) R.-Q. Fang, X.-H. Zhang and X.-M. Zhang, Cryst. Growth Des., 2006, 6, 2637 CrossRef CAS.
-
(a) S.-C. Xiang, X.-T. Wu, J.-J. Zhang, R.-B. Fu, S.-M. Hu and X.-D. Zhang, J. Am. Chem. Soc., 2005, 127, 16352 CrossRef CAS;
(b) S. M. Humphrey, R. A. Mole, M. McPartlin, E. J. L. McInnes and P. T. Wood, Inorg. Chem., 2005, 46, 5981 CrossRef;
(c) S. O. H. Gutschke, D. J. Price, A. K. Powell and P. T. Wood, Angew. Chem., Int. Ed., 2001, 40, 1920 CrossRef CAS;
(d) D. Cave, J.-M. Gascon, A. D. Bond, S. J. Teat and P. T. Wood, Chem. Commun., 2002, 1050 RSC;
(e) S. M. Humphrey and P. T. Wood, J. Am. Chem. Soc., 2004, 126, 13236 CrossRef CAS.
-
(a) M.-H. Zeng, W.-X. Zhang, X.-Z. Sun and X.-M. Chen, Angew. Chem., Int. Ed., 2005, 44 Search PubMed;
(b) X.-N. Cheng, W.-X. Zhang, Y.-Z. Zheng and X.-M. Chen, Chem. Commun., 2006, 3603 RSC;
(c) Y.-Z. Zheng, M.-L. Tong, W.-X. Zhang and X.-M. Chen, Chem. Commun., 2006, 165 RSC;
(d) Y.-Z. Zheng, M.-L. Tong, W.-X. Zhang and X.-M. Chen, Angew. Chem., Int. Ed., 2006, 45, 6310 CrossRef.
-
(a) J. Pasán, J. Sanchiz, C. Ruiz-Pérez, F. Lloret and M. Julve, New J. Chem., 2003, 27, 1557 RSC;
(b) J. Pasán, J. Sanchiz, C. Ruiz-Pérez, F. Lloret and M. Julve, Eur. J. Inorg. Chem., 2004, 4081 CrossRef CAS;
(c) J. Pasán, J. Sanchiz, C. Ruiz-Pérez, F. Lloret and M. Julve, Inorg. Chem., 2005, 44, 7794 CrossRef CAS;
(d) J. Pasán, J. Sanchiz, C. Ruiz-Pérez, J. Campo, F. Lloret and M. Julve, Chem. Commun., 2006, 2857 RSC.
-
(a)
B. Valeur, Molecular Fluorescence: Principles and Application, Wiley-VCH, Weinheim, 2002 Search PubMed;
(b) S.-L. Zheng, J.-H. Yang, X.-L. Yu, X.-M. Chen and W.-T. Wong, Inorg. Chem., 2004, 43, 830 CrossRef CAS;
(c) J.-H. He, J.-H. Yu, Y.-T. Zhang, Q.-H. Pan and R.-R. Xu, Inorg. Chem., 2005, 46, 9279 CrossRef.
-
(a) X.-M. Chen and G.-F. Liu, Chem.–Eur. J., 2002, 8, 4811 CrossRef CAS;
(b) X.-L. Wang, C. Qin, E.-B. Wang, Y.-G. Li and Z.-M. Su, Chem. Commun., 2005, 5450 RSC;
(c) H. Furukawa, J. Kim, K. E. Plass and O. M. Yaghi, J. Am. Chem. Soc., 2006, 128, 8398 CrossRef CAS;
(d) M. Du, C.-P. Li, X.-J. Zhao and Q. Yu, CrystEngComm, 2007, 9, 1011 RSC;
(e) R. Sun, S.-N. Wang, H. Xing, J.-F. Bai, Y.-Z. Li, Y. Pan and X.-Z. You, Inorg. Chem., 2007, 46, 8451 CrossRef CAS;
(f) S.-N. Wang, H. Xing, Y.-Z. Li, J.-F. Bai, M. Scheer, Y. Pan and X.-Z. You, Chem. Commun., 2007, 2239 Search PubMed;
(g) J. Wang, Z.-J. Lin, Y.-C. Ou, N.-L. Yang, Y.-H. Zhang and M.-L. Tong, Inorg. Chem., 2008, 47, 190 CrossRef CAS.
-
(a) J.-M. Rueff, S. Pillet, N. Claiser, G. Bonaventure, M. Souhassou and P. Rabu, Eur. J. Inorg. Chem., 2002, 895 CrossRef CAS;
(b) R.-H. Wang, M.-C. Hong, J.-H. Luo, R. Cao and J.-B. Weng, Chem. Commun., 2003, 1018 RSC;
(c) H. Chun, D. Kim, D. N. Dybtsev and K. Kim, Angew. Chem., Int. Ed., 2004, 43, 971 CrossRef CAS;
(d) B.-L. Chen, N. W. Ockwig, A. R. Millward, D. S. Contreras and O. M. Yaghi, Angew. Chem., Int. Ed., 2005, 44, 4745 CrossRef CAS;
(e) J. J. Perry IV, V. C. Kravtsov, G. J. McManus and M. J. Zaworotko, J. Am. Chem. Soc., 2007, 129, 10076 CrossRef CAS.
-
(a) S.-Q. Zang, Y. Su, Y.-Z. Li, Z.-P. Ni and Q.-J. Meng, Inorg. Chem., 2006, 45, 174 CrossRef CAS;
(b) S.-Q. Zang, Y. Su, Y.-Z. Li, H.-Z. Zhu and Q.-J. Meng, Inorg. Chem., 2006, 45, 2972 CrossRef CAS;
(c) S.-Q. Zang, Y. Su, Y.-Z. Li, Z.-P. Ni, H.-Z. Zhu and Q.-J. Meng, Inorg. Chem., 2006, 45, 3855 CrossRef CAS;
(d) S.-Q. Zang, Y. Su, Y. Song, Y.-Z. Li, Z.-P. Ni, H.-Z. Zhu and Q.-J. Meng, Cryst. Growth Des., 2006, 6, 2369 CrossRef CAS;
(e) Y. Su, S.-Q. Zang, C.-Y. Duan and Q.-J. Meng, Inorg. Chem. Commun., 2006, 10, 339;
(f) Y. Su, S.-Q. Zang, Y.-Z. Li and Q.-J. Meng, Cryst. Growth Des., 2007, 6, 1277 CrossRef.
- S.-Q. Zang, Y. Su, C.-Y. Duan, Y.-Z. Li, H.-Z. Zhu and Q.-J. Meng, Chem. Commun., 2006, 4997 RSC.
- B. F. Hoskins, R. Robson and D. A. Slizys, J. Am. Chem. Soc., 1997, 119, 2952 CrossRef CAS.
-
(a) G. M. Sheldrick, Acta Crystallogr., Sect. A: Found. Crystallogr., 1990, 46, 457;
(b)
G. M. Sheldrick, SHELXL-97, Program for Crystal Structure Refinement; University of Göttingen: Göttingen, Germany, 1997 Search PubMed.
-
(a) B. Q. Ma, K. L. Mulfort and J. T. Hupp, Inorg. Chem., 2005, 44, 4912 CrossRef CAS;
(b) Y.-T. Wang, H.-H. Fan, H.-Z. Wang and X.-M. Chen, Inorg. Chem., 2005, 44, 4128;
(c) M. Oh, C. L. Stern and C. A. Mirkin, Inorg. Chem., 2005, 44, 2647 CrossRef CAS;
(d) R. P. Feazell, C. E. Carson and K. K. Klausmeyer, Inorg. Chem., 2006, 45, 2627 CrossRef CAS.
-
(a) C. G. Subhash, A. M. Michael, Y. C. Michael and E. B. William, J. Am. Chem. Soc., 1991, 113, 1844 CrossRef CAS;
(b) I. A. M. Pohl, L. G. Westin and M. Kritikos, Chem.–Eur. J., 2001, 7, 3438 CrossRef;
(c) T. Devic, M. Evain, Y. Moelo, E. Canadell, P. Auban-Senzier, M. Fourmigue and P. Batail, J. Am. Chem. Soc., 2003, 125, 3295 CrossRef CAS;
(d) S.-N. Wang, H. Xing, Y.-Z. Li, J.-F. Bai, Y. Pan, M. Scheer and X.-Z. You, Eur. J. Inorg. Chem., 2006, 3041 CrossRef CAS;
(e) E.-Y. Choi, P. M. Barron, R. W. Novotney, C.-H. Hu, Y.-U. Kwon and W.-Y. Choe, ChrystEngComm, 2008, 10, 824–826 Search PubMed (this reference gives a clear presentation for this topology).
-
(a) L.-Y. Zhang, G.-F. Liu, S. L. Zheng, B.-H. Ye, X.-M. Zhang and X.-M. Chen, Eur. J. Inorg. Chem., 2003, 2965 CrossRef CAS;
(b) J.-C. Dai, X.-T. Wu, Z. Y. Fu, C.-P. Cui, S.- M. Wu, W.-X. Du, L.-M. Wu, H.-H. Zhang and Q. Sun, Inorg. Chem., 2002, 41, 1391 CrossRef CAS;
(c) X.-L. Wang, C. Qin, Y.-G. Li, N. Hao, C. W. Hu and L. Xu, Inorg. Chem., 2004, 43, 1850 CrossRef CAS.
- X.-L. Wang, C. Qin, E.-B. Wang, L. Xu, Z.-M. Su and C.-W. Hu, Angew. Chem., Int. Ed., 2004, 43, 5036 CrossRef CAS.
Footnote |
† Electronic supplementary information (ESI) available: Hydrogen bond distances and angles for 2–5 (Table S1); 3D supramolecular structure of 2 (Fig. S1); topology structure of 2 (Fig. S2); 2D metal–organic double layer structure of 3 (Fig. S3); TG curve of 2–5 (Fig. S4–S7); Fluorescent emission spectra of 2–5 (Fig. S8). CCDC reference numbers 685455–685458. For ESI and crystallographic data in CIF or other electronic format see DOI: 10.1039/b806899b |
|
This journal is © The Royal Society of Chemistry 2009 |
Click here to see how this site uses Cookies. View our privacy policy here.