DOI:
10.1039/B816273E
(Paper)
Analyst, 2009,
134, 93-96
Rapid detection of drug metabolites in latent fingermarks
Received
17th September 2008
, Accepted 4th November 2008
First published on 25th November 2008
Abstract
Magnetic particles functionalised with anti-cotinine antibody have been used to image latent fingermarks through the detection of the cotinine antigen in the sweat deposited within the fingerprints of smokers. The antibody–magnetic particle conjugates are readily applied to latent fingerprints while excess reagents are removed through the use of a magnetic wand. The results have shown that drug metabolites, such as cotinine, can be detected and used to image the fingermark to establish the identity of an individual within 15 minutes.
Introduction
Fingerprints are the most important form of physical evidence in the identification of an individual. Since no two humans possess identical fingerprints, fingermark recognition is vital in any forensic investigation.1,2 Latent fingerprints are marks left by a finger when it touches a surface and are not usually visible to the naked eye. To visualise latent prints, chemical or physical treatments are required.3 Recently, there has been considerable interest in the use of nanomaterials for the enhancement and visualisation of latent fingerprints.4–7 We have developed antibody–gold nanoparticle conjugates for the detection of the major metabolite of nicotine (cotinine) deposited in the sweat of latent fingerprints.8 These nanoconjugates were used to detect both the presence of the drug metabolite and simultaneously provide fluorescence images of the latent fingermark to enable identification. We have recently taken this concept further by conjugating antibodies to magnetic particles to detect narcotic drugs and metabolites in the fingerprints of drug users.9 Magnetic particles are an attractive option for fingerprinting as these are analogous to magnetic fingerprint powder – an enhancer which is currently used for the visualisation of fingermarks in forensic investigations.10,11
In many forensic situations rapid analysis/identification is essential. Here we show that antibody–magnetic particle conjugates can be used to image a fingermark through the detection of a drug metabolite within 15 min. For this study, with consideration of the wide availability of volunteers who smoke tobacco products, we have chosen to detect cotinine, a metabolite of nicotine.12 Therefore, magnetic microparticles were functionalised with an anti-cotinine antibody in order to detect cotinine in the sweat deposited with latent fingerprints. The optimisation of the application of the antibody–magnetic particle conjugates, including the removal of excess reagents with a forensic magnetic wand, are reported. A schematic representation of the method is shown in Fig. 1.
 |
| Fig. 1 A schematic representation of the detection ofcotinine antigen in the latent fingermark of an individual who smokes cigarettes. In the first step, Protein A/G-coated magnetic particles were conjugated with anti-cotinine antibody to prepare antibody–magnetic particles. Secondly, the anti-cotinine–magnetic particle conjugates were pipetted over the fingermark deposited on a glass microscope slide. Excess particles were removed by washing or by the use of a magnet. In the next step, secondary antibody fragment tagged with Alexa Fluor 488 dye was incubated over the fingerprint. Finally, the fluorescently labelled fingermark was imaged using a stereomicroscope. | |
Experimental
Formation of antibody–magnetic particle conjugates
Magnetic particles (ca. 1 µm) coated with recombinant fusion Protein A/G (1 : 1 molar ratio) were purchased from Bioclone Inc, USA, and were purified according to the manufacturer's instructions. Antibody was bound to the particles by mixing 27 µl of anti-cotinine antibody (5.54 mg/ml; Azog Inc, USA) with 150 µl of the magnetic particles. The mixed solution was incubated for 24 h at 4 °C. Unbound antibodies were removed using a magnetic separator (Bioclone Inc, USA) and the antibody–magnetic particle conjugates were washed 5 times with 1 ml of 100 mM phosphate buffer (57.5 mM Na2HPO4, 42.3 mM NaH2PO4, pH 7.0). Finally, the antibody–magnetic particle conjugates were resuspended in 600 µl of 100 mM phosphate buffer.
Fingermark collection
Glass microscope slides were cleaned using methanol (HPLC grade); a volunteer then applied a fingermark to each glass slide. The presence of a latent fingermark on each slide was confirmed by imaging with brightfield microscopy.
Imaging of the latent fingermarks
To retain the antibody–magnetic particle conjugates over the surface of the fingermark on the glass slide, a hydrophobic pen (Dako Pen, Dako, Denmark) was applied around the fingerprint. Then 100 µl of the anti-cotinine antibody–magnetic particle conjugates were applied to the fingerprint. The glass slide was incubated for either 10, 20 or 30 min at 37 °C in a wet chamber (consisting of a moist tissue surrounding the glass slide and contained within a covered Petri dish). After the incubation period, excess magnetic particles were removed either by washing with 400 µl (2 × 200 µl) of 100 mM phosphate buffer or through removal with a magnet (Magnetic Wand, Tetra Scene of Crime, UK). Subsequently, 100 µl of a 50 µg/ml solution of anti-mouse secondary antibody fragment (F(ab′)2 fragment of goat anti-mouse IgG), tagged with Alexa Fluor 488 dye (Invitrogen) was applied to the fingerprint. This fluorescently tagged secondary antibody fragment was incubated over the fingermark for 30 min at 37 °C in the wet chamber. The excess secondary antibodies were removed by washing with 400 µl (2 × 200 µl) of 100 mM phosphate buffer. Brightfield and fluorescence microscopy images of the fingerprints were obtained using a Zeiss M2 Bio Quad SV 11 stereomicroscope. The fingerprints were illuminated with either a halogen lamp (brightfield) or a 100 W Hg arc-lamp (fluorescence). The fluorescence images were obtained using a 470 nm excitation filter (40 nm bandpass) and a 525 nm emission filter (50 nm bandpass). All images were captured with an AxioCam HRc CCD camera and Axio Vision software (Carl Zeiss, UK). The images were processed using Adobe Photoshop.
Results and discussion
Anti-cotinine antibodies conjugated to recombinant fusion Protein A/G-coated magnetic particles were used to detect the presence of cotinine within a fingermark that had been deposited on a glass surface. Fig. 2 shows the brightfield (a and b) and fluorescence (c) images of a fingermark of an individual who reportedly smoked between 6 and 10 cigarettes per day. Fig. 2a is the brightfield image obtained immediately after collection of the fingerprint, prior to addition of the antibody–magnetic particle conjugates, to confirm the presence of the fingermark on the glass surface. Fig. 2b, also a brightfield image, was obtained after the subsequent incubation with anti-cotinine–magnetic particle conjugates and the fluorescently tagged secondary antibody fragment. From the brightfield images it can be seen clearly that a change in colour of the fingermark to yellow/brown occurs after the application of the particles. This colour change is due to the binding of the antibody–magnetic particles to the cotinine antigen deposited within the sweat of the fingerprint. The binding of the particles to the fingermark confirms the presence of the cotinine antigen in the fingerprint. The image shown in Fig. 2c confirms that the fluorescently tagged secondary antibody fragment has bound to the antibody–magnetic particle conjugates revealing the fluorescence image of the fingerprint. Previously we have shown that supporting the primary antibody on a nanoparticle surface significantly enhances the fluorescence imaging of the cotinine antigen within a fingermark as compared to the use of the anti-cotinine antibody alone.8Fig. 2b further highlights the advantage of conjugating the anti-cotinine antibody to the microparticles in that a change of colour is obtained when the antibody–magnetic particles bind to the target antigen within the fingerprint.
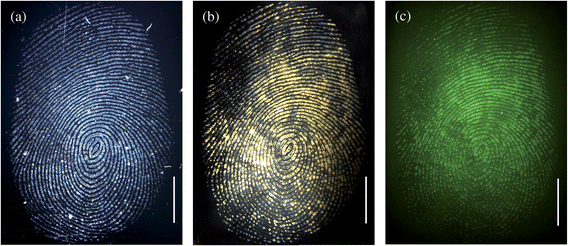 |
| Fig. 2 Brightfield images of a fingermark before (a) and after (b) incubation with the magnetic particles and secondary antibody fragment; (c) represents the fluorescence image of the fingerprint. In this experiment, the excess magnetic particles were removed by washing excess reagents with phosphate buffer. The scale bars = 5 mm. | |
Following the successful imaging of fingerprints of smokers based on the detection of the cotinine, a series of time-dependent experiments was performed to establish the speed with which the antigen could be detected whilst still providing images suitable for identification purposes. In Fig. 3 both brightfield and fluorescence microscopy images are shown which were obtained following the incubation of the fingermarks with antibody–magnetic particle conjugates for between 10 and 30 min. The brightfield images shown in Fig. 3(a, d, g) were obtained prior to the incubation with the particles, while the brightfield images (b, e, h) are those obtained from the fingerprints following application and incubation with the magnetic particle conjugates for 30, 20 and 10 min respectively. The fluorescence images shown in Fig. 3(c, f, i) are obtained from the fingerprints after 30, 20 and 10 min incubation of the antibody–magnetic particle conjugates, respectively. The images of Fig. 3 show that following incubation with the anti-cotinine–magnetic particles, for only 10 min, the distinctive colour change to yellow/brown due to binding of the particles is observed. For all three incubation times (30, 20 and 10 min) the typical fingermark ridge pattern, that is required to identify an individual, could be seen clearly in both brightfield and fluorescence images. Allowing time for the removal of the unbound magnetic particles, these results suggest that by using brightfield imaging with the antibody–magnetic particle conjugates (but without the fluorescently tagged secondary antibody), drug metabolites, such as cotinine, can be detected and used to image a latent fingermark within 15 min.
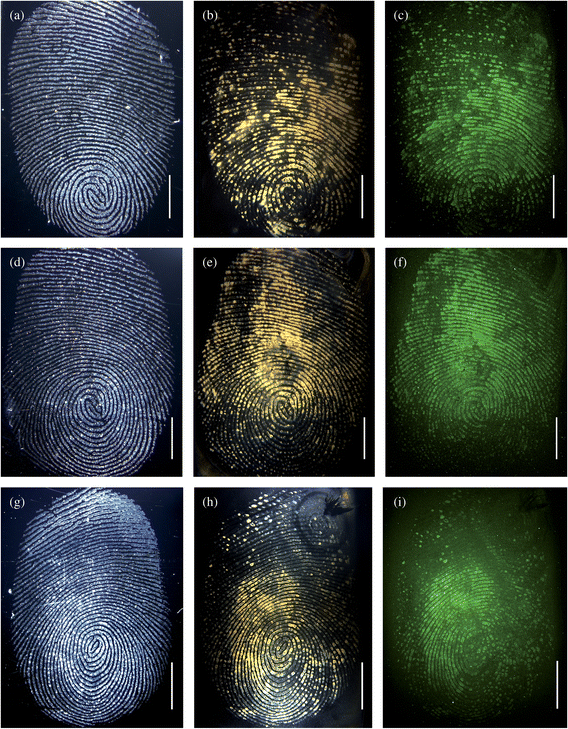 |
| Fig. 3 Images (a, d, g) represent the brightfield images of 3 different fingermarks prior to incubation with the conjugates and secondary antibody fragments; (b, e, h) represent the brightfield images after application of the anti-cotinine magnetic particle conjugates and secondary antibody fragments; (c, f, i) represent the fluorescence images after incubation with the particles and secondary antibody fragments. The fingerprints were incubated with magnetic particles for 30 (b, c), 20 (e, f), and 10 (h, i) minutes. In this experiment, the excess particles were removed with a magnet. The scale bars = 5 mm. | |
Fig. 4 shows the brightfield (upper row) and fluorescence (lower row) images of the fingerprints shown in Fig. 3 but at higher magnification. The magnified images of Fig. 4 clearly show secondary level details of the fingermark ridge pattern, such as bifurcations, which are used by fingermark examiners to match prints against fingermark databases and thereby confirm the identity of an individual. At this magnification the images also reveal third-level details such as the sweat pores, visible as dark spots along the ridge length, which are also used for identification purposes.
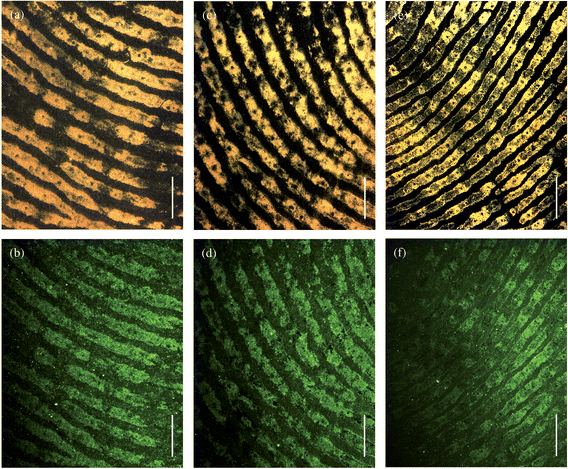 |
| Fig. 4 Magnified brightfield (a, c, e) and fluorescence (b, d, f) images of the fingerprints shown in Fig. 3. The magnetic particles were incubated for 30 (a, b), 20 (c, d), and 10 (e, f) minutes. The scale bars = 1 mm. | |
In summary, it has been shown that by functionalising magnetic particles with anti-cotinine antibody the cotinine antigen can be detected in latent fingerprints of smokers and used to image the fingerprints with sufficient clarity to enable identification of an individual. The results have clearly shown that latent fingerprints can reveal the presence of drug metabolites, as exemplified by cotinine, within 15 min.
Acknowledgements
This work was financially supported by the EPSRC (Grant EP/D041007/1). The authors gratefully acknowledge Andrew Bliss (Foster and Freeman), Sean Doyle (Forensic Explosives Laboratory, DSTL), Duncan Harding (Home Office Scientific Development Branch) and Andrew Hopwood (Forensic Science Service).
References
- H. Faulds, Nature, 1880, 22, 605 Search PubMed.
-
R. Saferstein, Criminalistics: An Introduction to Forensic Science, Prentice Hall, Inc., New Jersey, 7th edn, 2001 Search PubMed.
- S. Oden and B. Von Hofsten, Nature, 1954, 173, 449–450 CAS.
- A. Becue, C. Champod and P. Margot, Forensic Sci. Int., 2007, 168, 169–176 CrossRef CAS.
- M. Sametband, I. Shweky, U. Banin, D. Mandler and J. Almog, Chem. Commun., 2007, 11, 1142–1144 RSC.
- E. Stauffer, A. Becue, K. V. Singh, K. R. Thampi, C. Champod and P. Margot, Forensic Sci. Int., 2007, 168, e5–e9 CrossRef CAS.
- M. J. Choi, A. M. McDonagh, P. Maynard and C. Roux, Forensic Sci. Int., 2008, 179, 87–97 CrossRef CAS.
- R. Leggett, E. E. Lee-Smith, S. M. Jickells and D. A. Russell, Angew. Chem., Int. Ed., 2007, 46, 4100–4103 CrossRef CAS.
- P. Hazarika, S. M. Jickells, K. Wolff and D. A. Russell, Angew. Chem., Int. Ed., 2008 DOI:10.1002/anie.200804348.
- M. J. Choi, T. Smoother, A. A. Martin, A. M. McDonagh, P. J. Maynard, C. Lennard and C. Roux, Forensic Sci. Int., 2007, 173, 154–160 CrossRef CAS.
- L. K. Seah, U. S. Dinish, W. F. Phang, Z. X. Chao and V. M. Murukeshan, Forensic Sci. Int., 2005, 152, 249–257 CrossRef CAS.
- H. B. Hucker, J. R. Gillette and B. B. Brodie, J. Pharmacol. Exp. Ther., 1960, 129, 94–100 Search PubMed.
|
This journal is © The Royal Society of Chemistry 2009 |
Click here to see how this site uses Cookies. View our privacy policy here.