Paternò–Büchi reaction between aromatic carbonyl compounds and 1-(3-furyl)alkanols
Received
23rd October 2007
, Accepted 6th November 2007
First published on 29th November 2007
Abstract
The photochemical reaction between aromatic carbonyl compounds and 3-furylmethanol derivatives occurs with high regioselectivity. In most of the experiments formation of oxetanes occurs at the hydroxyalkylated double bond. With chiral 1-(3-furyl)alkanols the reaction occurs with good-high stereoselectivity. In the case of 1-(3-furyl)-benzyl alcohol the stereoselectivity can be explained on the basis of the conformers of the reagent, assuming the formation of a complex between the carbonyl compound and the hydroxyl group.
Introduction
The stereoselectivity of the Paternò–Büchi reaction on allylic alcohols has been studied in the last years.1 Adam and co-workers showed that the allylic alcohol 1 reacted with benzophenone to give the corresponding adducts 2 and 3 with high regio- and stereoselectivity (Scheme 1).2
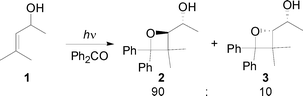 |
| Scheme 1 Paternò–Büchi reaction on allylic alcohols. | |
The reaction of 1-(2-furyl)ethanol 4 with benzophenone gave a 1
:
1 mixture of two diastereoisomers (Scheme 2).3 On the contrary, enantiopure 1-(2-furyl)-benzyl alcohol 6 gave the corresponding adduct 7 with d.e. > 98% (Scheme 2). The reaction of 1-(2-furyl)alkanols with aliphatic aldehydes and ketones gave the corresponding adducts 8 with high regioselectivity but no diastereoselectivity (Scheme 2).4 Finally, the reaction of 1-(2-furyl)-1-phenylethanol 9 with benzophenone gave the adduct 10 with d.e. = 48% (Scheme 2).5
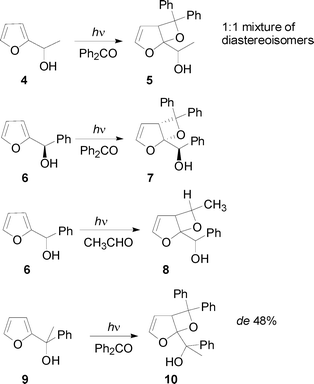 |
| Scheme 2 Paternò–Büchi reaction on 1-(2-furyl)alkanols. | |
These results were explained assuming the attack of the excited carbonyl compound on the C2/C3 double bond, through the formation of a hydrogen bond or of a complex. This type of attack gave the corresponding biradical intermediate in preferential conformations. The relative energies of these conformers account for the observed stereoselectivity.5,6
On the basis of these results we were not able to predict whether the presence of a hydroxyl group in the β position on the furan ring could induce regio- and stereoselectivity in the reaction with aromatic carbonyl compounds. Furthermore, recently no hydroxy directing effect was found in 2,3-dihydrofuran-3-ol derivatives.7 In this work we tested the reactivity of some 1-(3-furyl)alkanol derivatives.
Materials and methods
Photochemical synthesis of oxetanes—general procedure
3-Furylmethanol derivative (3 mmol) was dissolved in benzene (70 mL) in the presence of the carbonyl compound (4.5 mmol). The mixture was purged with nitrogen for 1 h and then irradiated with a 125 W high pressure mercury arc (Helios-Italquartz, Milan, Italy). At the end of the reaction the solvent was evaporated and the crude product was chromatographed on silica gel. Elution with ethyl acetate–hexanes 1
:
9 gave pure products. All the yields reported refer to isolated chromatographically pure products.
5-Hydroxymethyl-6,6-diphenyl-2,7-dioxabicyclo[3.2.0]-3-heptene
12
δ
H
(300 MHz; CDCl3; Me4Si) 7.4–7.2 (10 H, m, aromatic protons), 6.44 (1 H, d, J 2.6, 3-H), 6.26 (1 H, s, 1-H), 5.37 (1 H, d, J 2.6, 4-H), 3.71 (1 H, d, J 11, CHaHbOH), 3.54 (1 H, d, J 11, CHaHbOH), 1.42 (1 H, br s, OH); δC (CDCl3; Me4Si) 147.7 (C-3), 128.9, 128.0, 127.8, 127.1, 125.9, 105.9 (C-4), 105.6 (C-1), 96.9 (C-6), 66.6 (C-5), 62.1 (CH2OH). Analysis Found: C, 77.20; H, 5.62. C18H16O3 requires: C, 77.12; H, 5.75%.
5-(1-Hydroxy-n-heptyl)-6,6-diphenyl-2,7-dioxabicyclo-[3.2.0]-3-heptene
14
δ
H
(300 MHz; CDCl3; Me4Si) 7.7–7.2 (10 H, m, aromatic protons), 6.41 (1 H, d, J 2.4, 3-H), 6.20 (1 H, s, 1-H), 5.35 (1 H, d, J 2.4, 4-H), 3.58 (1 H, m, CHOH), 1.55 (1 H, d, J 4.2, OH), 1.28 (10 H, m, CH2), 0.88 (3 H, m, CH3); δC (CDCl3, Me4Si) 146.9 (C-3), 128.9, 128.7, 128.6, 128.3, 128.1, 127.9, 127.8, 127.6, 127.5, 127.2, 127.0, 126.2, 109.5 (C-4), 105.3 (C-1), 98.1 (C-6), 70.3 (CHOH), 69.6 (C-5), 32.9, 31.9, 30.0, 25.9, 22.8, 14.4. Analysis Found: C, 78.89; H, 7.85. C24H28O3 requires: C, 79.09; H, 7.74%.
5-(1-Hydroxy-n-heptyl)-6-phenyl-2,7-dioxabicyclo[3.2.0]-3-heptene
15
δ
H
(300 MHz; CDCl3; Me4Si) 7.5–7.4 (5 H, m, aromatic protons), 6.75 (1 H, s, 1-H), 6.20 (1 H, s, 3-H), 5.77 (1 H, s, 6-H), 5.63 (1 H, d, J 1, 4-H), 3.60 (1 H, m, CHOH), 3.50 (1 H, m, OH), 1.20 (10 H, m, CH2), 0.86 (3 H, m, CH3); δC (CDCl3; Me4Si) 148.3 (C-3), 138.5, 130.7, 129.9, 128.8, 128.5, 126.2, 108.2 (C-4), 105.9 (C-1), 68.8 (C-6), 65.1 (CHOH), 31.6, 31.4, 29.2, 29.0, 22.4, 14.0. Analysis Found: C, 74.81; H, 8.30. C18H24O3 requires: C, 74.97; H, 8.39%.
4-(1-Hydroxy-n-heptyl)-6-phenyl-2,7-dioxabicyclo[3.2.0]-3-heptene
16
δ
H
(500 MHz; CDCl3; Me4Si) 7.5–7.2 (5 H, m, aromatic protons), 6.74 (1 H, d, J 2.5, 1-H), 6.38 (1 H, s, 3-H), 5.61 (1 H, s, 6-H), 5.32 (1 H, d, J 2.5, 5-H), 3.47 (1 H, br s, CHOH), 1.25 (1 H, s, OH) 1.2-0.65 (13 H, m); δC (CDCl3; Me4Si) 150.3 (C-3), 138.2, 129.2, 126.3, 109.7 (C-4), 104.1 (C-1), 94.2 (C-6) 69.0 (C-5), 64.8 (CHOH), 32.3, 31.8, 30.0, 28.5, 25.5, 14.3. Analysis Found: C, 74.78; H, 8.54. C18H24O3 requires: C, 74.97; H, 8.39%.
5-(α-Hydroxybenzyl)-6,6-diphenyl-2,7-dioxabicyclo[3.2.0]-3-heptene
18
δ
H
(500 MHz; CDCl3; Me4Si) 7.7–7.1 (15 H, m, aromatic protons), 6.05 (1 H, d, J 3, 3-H), 6.04 (1 H, s, 1-H), 5.39 (1 H, d, J 3, 4-H), 4.62 (1 H, s, CHOH), 1.81 (1 H, br s, OH); δC (CDCl3; Me4Si) 144.6 (C-3), 140.7, 140.3, 137.3, 126.2, 125.8, 125.7, 125.3, 124.5, 124.2, 124.0, 123.7, 102.1 (C-4), 101.9 (C-1), 95.4 (C-6), 69.5 (CHOH), 67.8 (C-5). Analysis Found: C, 80.97; H, 5.54. C24H20O3 requires: C, 80.88; H, 5.66%.
Preparation of enantiomerically enriched 1-(3-furyl)alkanols by kinetic resolution
L-(+)-DIPT (diisopropyl L-tartrate) (0.54 mL, 5.15 mmol) was added to a solution of Ti(O-i-Pr)4 (0.64 mL, 4.30 mmol) in anhydrous CH2Cl2 (10.5 mL) at –20 °C. The temperature was lowered to –30 °C and maintained for 10 min. Then, (±)-3-furylmethanol derivative (0.750 g, 4.3 mmol), dissolved in anhydrous CH2Cl2 (0.51 mL), and t-butyl hydroperoxide (0.43 mL) were added. The mixture was maintained at –21 °C for 40 h under stirring. The mixture was poured into a mixture of 10% tartaric acid (0.09 mL), diethyl ether (3.51 mL), and NaF (0.54 g). The mixture was stirred at room temperature for 3 h and then filtered over Celite. The solvent was evaporated and the residue was dissolved in diethyl ether (18 mL) and treated with 1 N NaOH (8.8 mL) for 30 min under stirring at 0 °C. The mixture was washed with brine and dried over anhydrous Na2SO4. Evaporation of the solvent gave a crude product that was chromatographed on silica gel. The elution with 8
:
2 hexanes–diethyl ether gave pure products.
A solution of 17 (610 mg), Et3N (5.6 mL), acetic anhydride (2.7 mL) and a trace of N,N-dimethylaminopyridine (DMAP) was stirred at room temperature. The reaction was checked by TLC (9
:
1 hexanes–Et2O) and quenched after two hours with methanol (3–4 mL) by putting the flask in an ice bath. The solvents were evaporated and the residue was treated with n-hexane until the acetic acid was totally removed. The crude product (∼6 g) was chromatographed on a silica gel column (9
:
1 hexanes–Et2O) giving 189 mg of the acetylated product 19: δH (500 MHz; CDCl3; Me4Si) 7.45–7.30 (7 H, m), 6.88 (1 H, s), 6.36 (1 H, s), 2.15 (3 H, s); δC (CDCl3; Me4Si) 170.3, 143.7, 141.1, 139.6, 128.8, 128.4, 127.2, 125.8, 109.9, 70.6, 21.5; m/z, 216 (28%), 175 (15), 174 (82), 157 (30), 156 (32), 155 (24), 145 (25), 129 (30), 128 (100), 127 (22), 115 (10), 102 (14), 77 (20), 43 (38).
Photocycloaddition of acetylated 3-furylphenylmethyl alcohol and benzophenone
A solution of acetylated 3-furylphenylmethyl alcohol (100 mg) and benzophenone (1 g) in benzene (70 mL) was irradiated with a high pressure mercury lamp (125 W) for 27 h. Benzene was evaporated and the residue was chromatographed on a silica gel column (9
:
1hexanes–Et2O) giving two products (20: 49 mg; 21: 45 mg). 20: δH (500 MHz; CDCl3; Me4Si) 7.5–6.8 (15 H, m, aromatic protons), 6.71 (1 H, s, CHOAc), 6.27 (1 H, d, J 3, 3-H), 6.08 (1 H, s, 1-H), 5.01 (1 H, d, J 3, 4-H), 1.78 (3 H, s, OAc); δC (CDCl3; Me4Si) 169.7 (CO), 146.6 (C-3), 105.5 (C-1), 96.9 (C-6), 73.4 (CHOAc), 68.3 (C-5), 20.6 (CH3). 21: δH (500 MHz; CDCl3; Me4Si) 7.6–7 (15 H, m, aromatic protons), 6.24 (1 H, s, CHOAc), 6.17 (1 H, d, J 3, 3-H), 5.50 (1 H, s, 1-H), 5.44 (1 H, d, J 3, 4-H), 2.16 (3 H, s, OAc); δC (CDCl3; Me4Si) 168.2 (CO), 145.8 (C-3), 104.8 (C-1), 97.2 (C-6), 74.2 (CHOAc), 68.3 (C-5), 21.2 (CH3).
Results and discussion
The irradiation of 3-furylmethanol 11 in the presence of benzophenone with a 125 W high pressure mercury arc gave the corresponding adduct 12 (Scheme 3, Table 1): the reaction occurs only at the C2/C3 double bond. This behaviour is in agreement with our previous reported results in this field. The regioselectivity of the reaction depended on the relative stability of the biradical intermediate.1 In this case, addition of an excited carbonyl compound to C2 forms a tertiary radical site at C3, while such addition to C5 would generate only a secondary radical at C4. Unfortunately, the reaction of 11 in the presence of benzaldehyde did not occur (Scheme 3, Table 1). Probably, considering the mechanism of this reaction (see below), in this case, the retrocleavage reaction of the biradical intermediate does not allow the isolation of an oxetane.
Table 1 Paternò–Büchi reaction on 3-furylmethanol derivatives
Furan
|
Carbonyl compound
|
Irradiation time/h |
Product |
Yield (%)a |
All the yields refer to isolated chromatographically pure products.
|
11
|
Ph2CO
|
44 |
12
|
42 |
11
|
PhCHO
|
27 |
— |
— |
13
|
Ph2CO
|
18 |
14
|
73 |
(R)-13 |
Ph2CO
|
18 |
(1S,5S,5′R)-14 |
56 |
13
|
PhCHO
|
24 |
15
|
42 |
|
|
|
16
|
27 |
(R)-13 |
PhCHO
|
24 |
(1S,5S,6S,5′R)-15 |
54 |
|
|
|
16
|
36 |
17
|
Ph2CO
|
24 |
18
|
58 |
(R)-17 |
Ph2CO
|
24 |
(1S,5S,5′S)-18 |
60 |
17
|
PhCHO
|
18 |
— |
— |
The irradiation of 1-(3-furyl)-n-heptanol 13 in the presence of benzophenone gave the adduct 14 (Scheme 4, Table 1). In this case the reaction could be performed on enantiomerically enriched 13. The kinetic resolution of 13 by using Sharpless oxidation gave (R)-13 in 30% yield with an e.e. = 60% ([α]D = –20.4 (c 1, CHCl3)).8
The irradiation of (R)-13 in the presence of benzophenone gave the (1S,5S,5′R)-14 (Scheme 4, Table 1). For the stereochemical assignment see below. Chiral HPLC (Chiralcel OD) gave a d.e. = 23%. The irradiation of 13 in the presence of benzaldehyde gave a mixture of regioisomeric products 15 and 16 (Scheme 4, Table 1). The formation of regioisomers is due to the relative stability of the regioisomeric biradical intermediates.1 The same reaction was performed on (R)-13 showing that (1S,5S,6S,5′R)-15 was obtained with a d.e. = 43% while 16 was obtained as a mixture of diastereoisomers (Scheme 4, Table 1).
The stereochemical assignment of the products could be obtained on the basis of NOE (nuclear Overhauser effect) experiments on (1S,5S,6S,5′R)-15. In fact, the irradiation on the singlet at δ 5.77 ppm (proton at C-6) gave a positive NOE (7%) on the ortho aromatic protons at δ 7.48 ppm. Furthermore, we observed a negative NOE on the CHOH proton at 3.60 ppm. PM3 optimization of the structures showed that the most stable conformation of 15 with 1R,5R,6R,1′R configuration should not present this negative NOE (the distance between the ortho aromatic protons and CHOH was 4.76 Å) while this effect should be observable in the 1S,5S,6S,5′R diastereoisomer (the distance between the ortho aromatic protons and CHOH was 2.48 Å).
The irradiation of the 3-furylmethanol derivative 17 in the presence of benzophenone gave the adduct 18 (Scheme 5, Table 1). Kinetic resolution of 17 gave (R)-17 in 20% yield and e.e = 90% ([α]D = –9.6 (c 1, CHCl3)). The reaction of (R)-17 with benzophenone gave the (1S,5S,5′S)-18 diastereoisomer with a d.e. > 99% (Chiralcel OD) ([α]D = 67.0 (c 1, CHCl3)) (Scheme 5, Table 1). The relative configuration was assigned on the basis of the NOE experiments on 15. The irradiation of 17 in the presence of benzaldehyde gave only decomposition products (Scheme 5, Table 1). The reaction occurs but we were not able to isolate the product after chromatography. Probably, it decomposed during the purification process.
To confirm the presence of a hydroxyl directing effect in this reaction, we performed the reaction of the acetyl derivative of the compound 17 with benzophenone (Scheme 6). The reaction of 19 with benzophenone gave the corresponding adducts 20 and 21 with high regioselectivity. However, it was a 1
:
1 mixture of two diastereoisomeric compounds. This result is in agreement with the presence of a hydroxyl directing effect: if the hydroxy group is not present no diastereoselectivity is observed.
As reported above the observed stereoselectivity in the reaction of 2-furylmethanol derivatives was explained on the basis of the possible conformers of the biradical intermediates obtainable admitting a directing effect of the hydroxyl group through coordination or hydrogen bond with the carbonyl compound.5 Recently, a transient absorption with λmax at 531 nm has been observed in the reaction between furan and benzophenone in agreement with the presence of a biradical intermediate.9 The presence of the biradical intermediate is in agreement with the mechanism depicted in Scheme 7. We followed the reaction kinetics in order to unravel whether the kinetics of the reactions of 3-furylmethanol derivatives should be compatible with this mechanism. The reaction of 13 with benzophenone follows a second order kinetics (k = 1.6 × 10–3 M–1 s–1, Fig. 1) in agreement with a mechanism depicted in Scheme 7, where k = k1k2/k–1 (see appendix).10 These results are in agreement with the presence of a biradical intermediate.
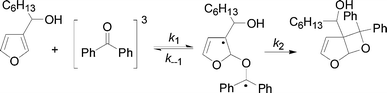 |
| Scheme 7 Proposed mechanism for the reaction 13 → 14. | |
To explain the stereochemistry, we performed some calculations (DFT/B3LYP/6-31G+(d,p)).11 We have only two conformers, 17A and 17B. 17A is more stable than the other one by 1.18 kcal mol–1 (Fig. 2). Then, there is not a preferential conformation.
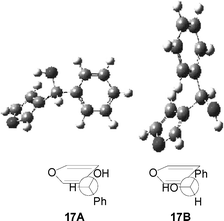 |
| Fig. 2 Conformers of 17. | |
We used the approach described in another paper.5 The attack of photoexcited benzophenone on 17 affects the formation of diastereoisomeric biradical intermediates. The directing effect exerted by the hydroxyl group is either due to the formation of a hydrogen bond between the hydroxyl group and the oxygen of the excited carbonyl compound, or to the formation of a complex. This type of interaction could favour the formation of a preferential conformation in the biradical intermediate where the hydroxyl group and the oxygen of the carbonyl compound are near. These conformations could have different energies for different diastereoisomeric biradicals, giving an explanation of the observed behaviour. The above described hypothesis requires that the biradical intermediates have a very short lifetime enabling them to equilibrate to the most stable one. In Fig. 3 we reported the calculated conformations of the biradical intermediates.
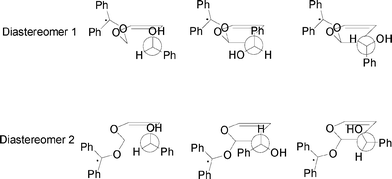 |
| Fig. 3 Calculated conformations of the diastereomeric biradical intermediates in the reaction between 17 and photoexcited benzophenone. | |
The above described conformations of 17, when attacked by benzophenone and assuming the formation of a hydrogen bond between the hydroxy group and the oxygen atom of the excited carbonyl compound, can give the biradical intermediate conformation described in Fig. 4. The conformation of the biradical intermediate is able to induce the observed stereoselectivity. In fact, it induces the formation of the 1S,5S stereoisomer, if we start from (R)-17, and the 1R,5R stereoisomer starting from (S)-17.
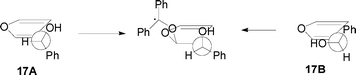 |
| Fig. 4 The biradical intermediate obtained from the calculated conformations of 17. | |
In the case of the compound (R)-13, calculations revealed two relevant conformers 13A and 13B (Fig. 5). Also in this case, there is not a preferential conformation (13A is more stable than 13B by 0.7 kcal mol–1).
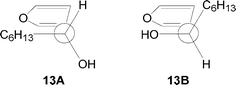 |
| Fig. 5 Relevant conformers of 13. | |
In the reaction of (R)-13 with benzophenone six conformers of the biradical intermediates could be obtained. These conformers are reported in Fig. 6(A).
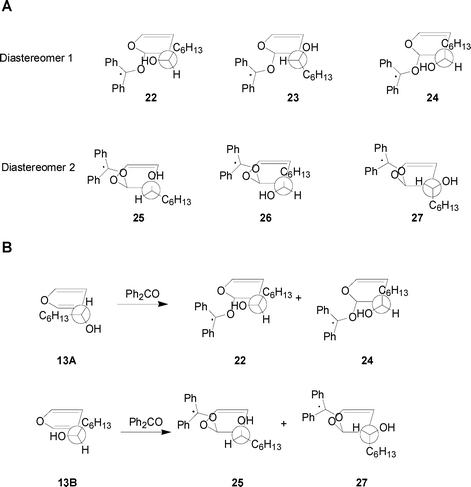 |
| Fig. 6 (A) Calculated conformations of the two diastereoisomeric biradical intermediates in the reaction between 13 and photoexcited benzophenone. (B) Relevant conformers of the possible biradical intermediates between 13 and photoexcited benzophenone. | |
Assuming the presence of a hydroxy directing effect, benzophenone can give only four conformers of the biradical intermediates, two of them are RS conformers (22, 24) while the other two are RR ones (25, 27) (Fig. 6(B)).
The energies of the conformers 22, 24, 25, and 27 are reported in Table 2. We can see that RS conformers are more stable than the RR ones. Furthermore, the energy difference between the most stable RS conformer (22) and the most stable RR conformer (25) is 10.88 kcal mol–1. This energy difference is in agreement with the observed diastereoisomeric excess.5
Table 2 Energy (in hartree, Eh) of the relevant conformers obtained in the reaction between 13 and benzophenone
Conformer |
Energy/Eh |
22
|
–1157.07698862 |
24
|
–1157.07585758 |
25
|
–1157.07284048 |
27
|
–1157.07134174 |
In conclusion, we showed that the Paternò–Büchi reaction on 3-furylmethanols can occur with high regioselectivity and with a good stereoselectivity, depending on the hydroxyl directing effect.
Appendix
For a sequence |  | (1) |
Assuming that the reaction of A with B follows a second order kinetics, we have |  | (2) |
|  | (3) |
Applying the steady state approximation |  | (4) |
| 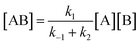 | (5) |
Then | 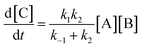 | (6) |
Assuming k–1 ≫ k2, we obtain | 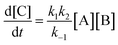 | (7) |
References
- M. D'Auria, L. Emanuele and R. Racioppi, Regio- and stereoselectivity in the Paternò–Büchi reaction on furan derivatives, Int. J. Photoenergy, 2006, 2006, ID 84645 Search PubMed; M. D'Auria, L. Emanuele and R. Racioppi, 1,2-Cycloaddition reaction of carbonyl compounds and pentaatomic heterocyclic compounds, Adv. Photochem., 2005, 28, 81–127 CAS; M. D'Auria, L. Emanuele and R. Racioppi, Regio- and diastereoselectivity in the Paternò–Büchi reaction on furan derivatives, The Spectrum, 2004, 17, 22–27 Search PubMed; M. D'Auria, Paternò–Büchi reaction on furan: regio- and stereochemistry, Targets Heterocycl. Syst., 2003, 7, 157–173 Search PubMed; M. D'Auria, L. Emanuele, R. Racioppi and G. Romaniello, The Paternò–Büchi reaction on furan derivatives, Curr. Org. Chem., 2003, 7, 1443–1459 CAS.
- W. Adam, K. Peters, E. M. Peters and V. R. Stegmann, Hydroxy-directed regio- and diastereoselective [2 + 2] photocycloaddition (Paternò–Büchi reaction) of benzophenone to chiral allylic alcohols, J. Am. Chem. Soc., 2000, 122, 2958–2959 CrossRef CAS; W. Adam and V. R. Stegmann, Hydroxy-group directivity in the regioselective and diastereoselective [2 + 2] photocycloaddition (Paternò–Büchi reaction) of aromatic carbonyl compounds to chiral and achiral allylic substrates: the preparation of oxetanes with up to three stereogenic centers as synthetic building blocks, Synthesis, 2001, 1203–1214 CrossRef CAS; M. Prein and W. Adam, The Schenck ene reaction: diastereoselective oxyfunctionalization with singlet oxygen in synthetic applications, Angew. Chem., Int. Ed. Eng., 1996, 35, 477–478 CrossRef CAS.
- M. D'Auria, R. Racioppi and G. Romaniello, The Paternò–Büchi Reaction on 2-furylmethanols, Eur. J. Org. Chem., 2000, 3265–3272 CrossRef CAS.
- M. D'Auria, L. Emanuele, R. Racioppi and G. Romanielllo, On the stereoselectivity of the Paternò–Büchi reaction between carbonyl compounds and 2-furylmethanol derivatives. The case of aliphatic aldehydes and ketones, Tetrahedron, 2002, 58, 5045–5051 CrossRef CAS.
- M. D'Auria, L. Emanuele and R. Racioppi, The stereoselectivity of the Paternò–Büchi reaction between tertiary 2-furylmethanol derivatives and aromatic carbonyl compounds. On the nature of the hydroxy directing effect, Photochem. Photobiol. Sci., 2004, 3, 927–932 RSC.
- M. D'Auria, L. Emanuele and R. Racioppi, Stereoselectivity in the Paternò–Büchi reaction on chiral allylic alcohol, for a discussion of the hydroxy directing effect, Lett. Org. Chem., 2005, 2, 132–135 Search PubMed.
- M. Abe, M. Terazawa, K. Nozaki, A. Masuyama and T. Hayashi, Notable temperature effect on the stereoselectivity in the photochemical [2 + 2] cycloaddition reaction (Paternò–Büchi reaction) of 2,3-dihydrofuran-3-ol derivatives with benzophenone., Tetrahedron Lett., 2006, 47, 2527–2530 CrossRef CAS.
- Y. Kobayashi, M. Kukasabe, Y. Kitano and F. Sato, Preparation of optically active 2-furylcarbinols by kinetic resolution using Sharpless reagent, J. Org. Chem., 1988, 53, 1586–1587 CrossRef CAS; T. Kametani, M. Tsubuchi, T. Tatsuzaki and T. Honda, Kinetic resolution of 2-furylcarbinols using the Sharpless oxidation and its application to the synthesis of (5R,6S)-6-acetoxy-5-hexadecanolide, Heterocycles, 1988, 27, 2107–2110 CrossRef CAS; M. Kukasabe, Y. Kitano, Y. Kobayashi and F. Sato, Preparation of optically active 2-furylcarbinols by kinetic resolution using Sharpless reagent and their application in organic synthesis, J. Org. Chem., 1989, 54, 2085–2091 CrossRef.
- M. Abe, T. Kawakami, S. Ohata, K. Nozaki and M. Nojima, Mechanism of stereo- and regioselectivity in the Paternò–Büchi reaction of furan derivatives with aromatic carbonyl compounds: importance of the conformational distribution in the intermediary triplet 1,4-dicardicals, J. Am. Chem. Soc., 2004, 126, 2838–2846 CrossRef CAS.
- H. Buschmann, H.-D. Scharf, N. Hoffmann and P. Esser, The isoinversion principle-a general model of chemical selectivity, Angew. Chem., Int. Ed. Engl., 1991, 30, 47–515.
-
M. J. Frisch, G. W. Trucks, H. B. Schlegel, G. E. Scuseria, M. A. Robb, J. R. Cheeseman, J. A. Montgomery, Jr., T. Vreven, K. N. Kudin, J. C. Burant, J. M. Millam, S. S. Iyengar, J. Tomasi, V. Barone, B. Mennucci, M. Cossi, G. Scalmani, N. Rega, G. A. Petersson, H. Nakatsuji, M. Hada, M. Ehara, K. Toyota, R. Fukuda, J. Hasegawa, M. Ishida, T. Nakajima, Y. Honda, O. Kitao, H. Nakai, M. Klene, X. Li, J. E. Knox, H. P. Hratchian, J. B. Cross, V. Bakken, C. Adamo, J. Jaramillo, R. Gomperts, R. E. Stratmann, O. Yazyev, A. J. Austin, R. Cammi, C. Pomelli, J. Ochterski, P. Y. Ayala, K. Morokuma, G. A. Voth, P. Salvador, J. J. Dannenberg, V. G. Zakrzewski, S. Dapprich, A. D. Daniels, M. C. Strain, O. Farkas, D. K. Malick, A. D. Rabuck, K. Raghavachari, J. B. Foresman, J. V. Ortiz, Q. Cui, A. G. Baboul, S. Clifford, J. Cioslowski, B. B. Stefanov, G. Liu, A. Liashenko, P. Piskorz, I. Komaromi, R. L. Martin, D. J. Fox, T. Keith, M. A. Al-Laham, C. Y. Peng, A. Nanayakkara, M. Challacombe, P. M. W. Gill, B. G. Johnson, W. Chen, M. W. Wong, C. Gonzalez and J. A. Pople, GAUSSIAN 03 (Revision A.1), Gaussian, Inc., Wallingford, CT, 2003 Search PubMed.
|
This journal is © The Royal Society of Chemistry and Owner Societies 2008 |
Click here to see how this site uses Cookies. View our privacy policy here.